DOI:
10.1039/C9JA00022D
(Technical Note)
J. Anal. At. Spectrom., 2019,
34, 1272-1278
FI-ICP-TOFMS for high-throughput and low volume multi-element analysis in environmental and biological matrices
Received
18th January 2019
, Accepted 25th March 2019
First published on 28th March 2019
Abstract
The capabilities of flow injection inductively coupled plasma time-of-flight mass spectrometry (FI-ICP-TOFMS) were evaluated for accurate multi-element analysis addressing water and serum reference materials with a sample intake of 5 μL in comparison to FI-ICP-MS/MS analysis. A comparison of the standard operation mode and the collision/reaction cell technology (CCT) of the ICP-TOFMS yielded lower limits of quantification, higher mass resolving power and improved precision for most elements when using H2/He gas. Overall, in the studied water matrix, accuracy of quantification for a large panel of elements was obtained in both modes (with the exception of low iron concentration in the standard mode). By increasing matrix complexity, as exemplified in the multi-element quantification exercise in serum, the FI-ICP-TOFMS approach proved to be fit-for-purpose. Accurate quantification of key elements with major biological function (Mg, Al, Fe, Cu, Zn, Se and Cd) was possible in serum ClinCheck reference material in standard operation mode without the need for prior sample mineralization and dilution. Cross-validation by quadrupole-based FI-ICP-MS/MS was performed showing the unique capability of ICP-TOFMS regarding accurate high-throughput quantification within transient signals of few seconds. For accurate FI-ICP-MS/MS analysis, multiple sample injections using standard mode and O2 gas mode were required for multi-element analysis in a short transient signal. Hence, this study demonstrates that the presented method is a valuable tool for trace element determination in environmentally and biologically relevant matrices at unprecedented speed.
Introduction
Inductively coupled plasma-mass spectrometry (ICP-MS) provides multi-element detection in complex matrices with high sensitivity at concentrations over a linear working range that spans several orders of magnitude. Especially clinical applications demand miniaturization of sample volumes required for analysis, shortening sample preparation steps and analysis time to enable high-throughput methods gaining multi-element information to elucidate elemental pattern or biomarkers responsible for certain diseases.1 Due to the complexity of biological matrices, in most cases samples are either digested or diluted reaching an end volume in the mL range and the interference removal is addressed by using high mass resolving power or by collision/reaction cell ICP-MS technology.2 In recent years, the latter one has gained more attention due to the upcoming of a tandem mass spectrometry configuration for ICP-quadrupole-based MS instruments allowing improved interference control over single quadrupole-based instruments as demonstrated by various studies.3,4 However, most of these studies focused on the accurate interference-free quantification of one or a few elements such as S, Ti, As, Se, etc. in a complex matrix.5–8 Aiming at multi-element profiling in biological fluids, several studies developed ICP-MS/MS methods for ionomics approaches in serum samples.9,10 These methods were based on 200–500 μL sample intake and further dilution to the mL range and made use of different gas modes in one measurement session resulting in increased sample volume and analysis time due to the transition time between different gas modes (typically between 10–20 s) while measuring around 20–30 elements.9,10 Although the further development of ICP-MS instruments has been on-going over the last years,11 quadrupole-based and single collector sector field (SF) instruments are still the most widely used ICP-MS systems. A detailed overview of common mass analyzers and detectors used in the field of ICP-MS and their limitations for measuring short transient signals is given by Tanner et al.12 In terms of analysis involving transient signals, ICP-TOFMS provides multi-element detection speed unavailable to scanning-based ICP-MS instruments as it permits the quasi-simultaneous analysis (ions are sampled simultaneously but read-out sequentially) of a large part of the full mass range. The advantages of a time-of-flight ICP-MS compared to a quadrupole-based ICP-MS regarding the multi-element detection of short transient signals was already shown in 2002.13 From the commercially available ICP-TOFMS instruments, the ‘CyTOF’ instrument from Fluidigm is a specialized design for single cell analysis and multiplex imaging using laser ablation (LA) after labelling of antibodies with metal tags.14 The instrument provides sensitivities approaching those of ICP-SFMS instruments, but has at the same time decreased mass resolving power and a restricted mass range (m/z = 75–209).15 Based on the design of a previously reported prototype,16 a commercial ICP-TOFMS instrument, ‘icpTOF’ was developed. The icpTOF is available with mass resolving power (R = m/Δm) of 3000 and 6000 (FWHM definition) and detection of m/z ranging from 2–256. ICP-MS applications demanding multi-element detection in the sub-second time range are for example high spatial resolution imaging by laser ablation using low dispersion ablation cell setups,17–19 single cell analysis20 and single particle analysis.21–23 There is on-going discussion about the performance of ICP-TOFMS instruments as their major limitation has long been recognized to be limited linear dynamic range together with sensitivities below that of quadrupole and sectorfield-ICP-MS instruments. Recent studies reported on the performance characteristics of the icpTOF instrument using different introduction systems.24,25 One report investigated the impact of using H2, He or a mixture thereof as collision/reaction gas for multi-element analysis.26
In this work, we present a flow injection ICP-TOFMS method using an icpTOF 2R instrument for multi-element quantification in TM-28.4 Lake Ontario water reference material and in serum ClinCheck Serum Control reference material based on a sample intake of 5 μL. The performance characteristics of the ICP-TOFMS method using standard operation mode and CCT mode were compared to a tandem ICP-MS/MS configuration using standard mode and oxygen gas mode.
Experimental
Chemicals and reagents
Ultrapure water (18.2 MΩ cm, ELGA Water purification system, Purelab Ultra MK 2, UK) and nitric acid (≥69% (m/m), Rotipuran Supra, Germany) was used for all dilutions for ICP-MS measurements. A multi-element stock solution containing aluminum, antimony, arsenic, barium, beryllium, boron, cadmium, calcium, chromium, cobalt, copper, iron, lead, magnesium, manganese, molybdenum, nickel, selenium, silver, sodium, thallium, titanium, vanadium and zinc with a concentration of 100 mg L−1 was purchased from Labkings (The Netherlands). The TM-28.4 Lake Ontario water certified reference material (Environment and Climate Change, Burlington, Canada) was used as reference for environmental matrices. The serum reference materials Seronorm (Seronorm Trace Elements Serum L-1, Norway) and ClinCheck Serum Control (Recipe Chemicals, Munich, Germany) were reconstituted according to the manufacturer's protocol and were used as reference for biological matrices. All other reagents and solvents were obtained from commercial sources and have been used without further purification. Sample preparation and measurements were carried out in clean room classes 100.000 and 10.000, respectively.
Instrumentation
ICP-TOFMS.
Measurements were performed with an icpTOF 2R (TOFWERK AG, Thun, Switzerland) TOF-based ICP-MS instrument with a mass resolution (R = m/Δm) of 6000 (FWHM definition). The standard operation mode was used which balances mass resolving power, sensitivity and ion transmission across the entire measured mass range and which allows the analysis of ions with m/z = 14–256 and complete mass spectra were acquired every 46 μs. For the analysis of ions with m/z < 14 tuning for low-mass ion transmission is required due to the cut-off of ions with low m/z values in the RF transfer optics of e.g. the notch filter. As tuning of low masses is accompanied by a loss of sensitivity of ions with higher m/z and the low mass range was not of interest for this study, only the standard operation mode was used. The sample introduction system consisted of a MicroFlow PFA-ST nebulizer (100 μL min−1 nominal sample uptake, Elemental Scientific Inc., Omaha, USA) and a quartz cyclonic spray chamber (Elemental Scientific Inc., Omaha, USA) that was Peltier-cooled to 2 °C. The optimized nebulizer Ar gas flow rate of ∼1.18 L min−1 resulted in a net sample uptake rate of ∼100 μL min−1. The instrument was equipped with a torch injector of 2.5 mm inner diameter and nickel sample and skimmer cones with a skimmer cone insert of 2.8 mm inner diameter. The instrument was tuned daily in order to achieve maximum intensities based on the 59Co+, 115In+ and 238U+ signal, to achieve a low oxide formation (140Ce16O+/140Ce+ < 1.5%) and a doubly charged ratio of 138Ba2+/138Ba+ < 2%. In case the CCT mode was used, the collision/reaction cell was pressurized with a mixture of H2/He gas with an optimized flow rate of 2.8 mL min−1. The following CCT parameters were used: CCT focus: 1.5 V, CCT entry lens: −40 V, CCT mass: 250 V, CCT bias: 1.5 V, CCT exit lens: −160 V. The instrumental parameters for ICP-TOFMS measurements are summarized in Table 1.
Table 1 Instrumental parameters for ICP-TOFMS and ICP-MS/MS measurements
|
ICP-TOFMS |
ICP-MS/MS |
Plasma power [W] |
1550 |
1550 |
Cone materials |
Ni |
Ni |
Plasma gas flow [L min−1] |
14.0 |
15.0 |
Auxiliary gas flow [L min−1] |
0.80 |
0.80 |
Nebulizer gas flow [L min−1] |
1.18 |
1.10 |
Masses notched |
16O2+, 37Cl+, 40Ar+ |
— |
Nebulizer nominal uptake |
MicroFlow PFA-ST 100 μL min−1 |
MicroMist 200 μL min−1 |
Spray chamber |
Cyclonic spray chamber |
Double-pass spray chamber |
ICP-TOFMS data were saved in the open-source hierarchical data format (HDF5, www.hdfgroup.org). Post-acquisition data processing was performed with Tofware 3.1.0, which is a TOFWERK data analysis package and is used as add-on on IgorPro (Wavemetric Inc., Oregon, USA). The data processing comprised the following steps: (1) drift correction of the mass peak position in the spectra over time via mass calibration (2) determining the peak shape (3) fitting and subtracting the mass spectral base-line and (4) calculating high-resolution peak fits for peak deconvolution. For flow injection measurements, the chromatographic workflow implemented in Tofware was used for data evaluation. Following post-processing in Tofware, integrated mass-spectral signals were exported as csv files and further processed with Microsoft Excel 2016 (Microsoft Corporation, California, USA).
ICP-MS/MS.
Measurements were performed with an Agilent 8800 ICP-MS/MS unit (Agilent Technologies, Tokyo, Japan). The ICP-MS instrument was equipped with an ASX 500 autosampler (Agilent Technologies, Waldbronn, Germany) for measurements using pneumatic nebulization. The sample introduction system consisted of a MicroMist nebulizer (200 μL min−1 nominal sample uptake, AHF Analysentechnik AG, Tuebingen, Germany) and a quartz double-pass spray chamber (Agilent Technologies, Waldbronn, Germany) that was Peltier-cooled to 2 °C. The instrument was tuned on a daily basis in order to achieve maximum sensitivity, low oxide formation (140Ce16O+/140Ce+ < 1.5%) and low doubly charged ratio (140Ce2+/140Ce+ < 2%). Elements were monitored in no gas mode and using oxygen as reaction gas with a flow rate of 0.32 mL min−1 and mass selection steps on both quadrupoles. The following isotopes with a respective integration time of 0.05 s were monitored in no gas mode: 24Mg, 27Al, 44Ca, 51V, 52Cr, 55Mn, 57Fe, 59Co, 60Ni, 65Cu, 66Zn, 95Mo, 107Ag, 111Cd, 205Tl and 208Pb. The following isotopes with a respective integration time of 0.05 s were monitored in oxygen gas mode using mass shift modality: 24Mg → 40Mg, 27Al → 43Al, 44Ca → 60Ca, 47Ti → 63Ti, 56Fe → 72Fe, 57Fe → 73Fe, 66Zn → 82Zn, 75As → 91As, 78Se → 94Se, 111Cd → 127Cd and 208Pb → 224Pb. The data were recorded and evaluated with the Agilent MassHunter Chromatography software package, MassHunter 4.1 Version C.01.01, 2014. Instrumental parameters for ICP-MS/MS measurements are summarized in Table 1.
HPLC system.
For flow injection measurements, a Dionex ICS-5000+ Ion Chromatography system (Thermo Fisher Scientific, Bremen, Germany) was coupled with a PEEK tubing (0.127 mm ID, length approx. 0.5 m) directly to the nebulizer of the ICP-TOFMS or ICP-MS/MS instrument. The following chromatographic conditions were used: injection volume: 5 μL; flow rate: 0.30 mL min−1; isocratic elution; CH3COONH4 (50 mM, pH = 6.8) or 0.1% (v/v) formic acid aqueous solution was employed as mobile phase.
Sample preparation
Quantification was performed with external calibration using two different approaches. For multi-element determination in the TM-28.4 Lake Ontario water reference material, multi-element standards were prepared in 3% (v/v) HNO3 by serial dilution of the multi-element stock solution and the mobile phase of the HPLC system was based on a 0.1% (v/v) formic acid aqueous solution. For multi-element analysis in the serum ClinCheck reference material, matrix-matched standards were prepared using serial dilutions of serum Seronorm reference material in NaCl solution (150 mM). External calibration was performed using the target values of the serum reference material to set up calibration curves. In this case, the mobile phase of the HPLC system was based on a CH3COONH4 solution (50 mM, pH = 6.8). All standards and samples were measured undiluted using flow injection with a sample intake of 5 μL in combination with ICP-TOFMS detection in standard operation mode and using collision/reaction cell pressurized with H2/He gas, and with ICP-MS/MS detection in standard mode and oxygen gas mode with the instrumental parameters given in Table 1.
Results and discussion
Limits of detection and quantification for ICP-TOFMS and ICP-MS/MS
Limits of detection (LOD) and quantification (LOQ) are reported in Table 2 for a wide range of elements for ICP-TOFMS detection in standard operation and CCT mode in comparison to ICP-MS/MS detection in standard operation and oxygen gas mode. Pneumatic nebulization was used to evaluate the instrumental performance of both ICP-MS systems. For the flow injection method used in this study, a metal-free ion chromatography system was employed in order to ensure a low background from the sample introduction system. Limits of quantification were in general lower for ICP-MS/MS than for ICP-TOFMS, however also for ICP-TOFMS they were in the low μg L−1 range. A comparison of the standard operation mode and the collision/reaction cell mode in ICP-TOFMS revealed lower limits of quantification and higher mass resolving power for most elements when using H2/He gas. Both setups, pneumatic nebulization and flow injection using 5 μL as injection volume in combination with ICP-TOFMS detection proved to be fit-for-purpose for accurate multi-element quantification in lake water and serum reference materials.
Table 2 Limits of detection and quantification using direct nebulization and flow injection with an injection volume of 5 μL and multi-element calibration standards in 3% (v/v) nitric acid, followed by ICP-TOFMS detection in standard operation mode and H2/He gas mode, and ICP-MS/MS detection in standard mode and oxygen gas mode. Instrumental LODs and LOQs were calculated using three and ten times the standard deviation of blank measurements (n = 5), respectively and are given in μg L−1. Isotopes marked with ‘—’ were not measured in the case of ICP-MS/MS and not detected in the blank in the case of ICP-TOFMS
Isotope |
ICP-TOFMS |
ICP-MS/MS – standard mode |
ICP-MS/MS – O2 gas mode |
Direct nebulization – standard mode |
Flow injection – standard mode |
Flow injection – H2/He gas mode |
Direct nebulization |
Flow injection |
Direct nebulization |
Flow injection |
LOD |
LOQ |
LOD |
LOQ |
LOD |
LOQ |
LOD |
LOQ |
LOD |
LOQ |
LOD |
LOQ |
LOD |
LOQ |
27Al |
0.022 |
0.073 |
9.92 |
33.0 |
2.42 |
8.07 |
0.080 |
0.266 |
3.22 |
10.7 |
0.548 |
1.83 |
3.70 |
12.3 |
47Ti |
— |
— |
1.46 |
4.88 |
6.04 |
20.1 |
— |
— |
— |
— |
— |
— |
— |
— |
51V |
0.025 |
0.084 |
0.325 |
1.09 |
0.203 |
0.678 |
0.002 |
0.008 |
0.106 |
0.354 |
0.001 |
0.002 |
— |
— |
52Cr |
— |
— |
0.370 |
1.23 |
0.334 |
1.11 |
— |
— |
0.376 |
1.25 |
— |
— |
— |
— |
53Cr |
0.074 |
0.246 |
0.995 |
3.32 |
0.532 |
1.77 |
0.016 |
0.053 |
— |
— |
0.132 |
0.440 |
— |
— |
55Mn |
0.003 |
0.012 |
1.15 |
3.81 |
0.197 |
0.658 |
0.003 |
0.010 |
0.478 |
1.59 |
0.015 |
0.048 |
— |
— |
56Fe |
0.569 |
1.90 |
4.87 |
16.22 |
3.42 |
11.4 |
— |
— |
— |
— |
0.042 |
0.142 |
4.18 |
13.9 |
57Fe |
— |
— |
3.85 |
12.8 |
1.80 |
5.99 |
— |
— |
14.9 |
49.6 |
0.474 |
1.58 |
8.07 |
26.9 |
59Co |
0.005 |
0.016 |
0.101 |
0.338 |
0.086 |
0.286 |
0.002 |
0.006 |
0.469 |
1.56 |
— |
— |
— |
— |
60Ni |
0.001 |
0.004 |
0.311 |
1.04 |
0.337 |
1.12 |
0.039 |
0.131 |
0.402 |
1.34 |
— |
— |
— |
— |
65Cu |
0.033 |
0.109 |
2.86 |
9.55 |
1.25 |
4.16 |
0.017 |
0.056 |
2.51 |
8.35 |
— |
— |
— |
— |
66Zn |
— |
— |
6.36 |
21.2 |
0.704 |
2.35 |
— |
— |
7.88 |
26.3 |
— |
— |
21.0 |
69.9 |
67Zn |
— |
— |
2.83 |
9.44 |
1.56 |
5.21 |
— |
— |
— |
— |
— |
— |
— |
— |
75As |
0.015 |
0.048 |
0.846 |
2.82 |
0.062 |
0.206 |
0.005 |
0.016 |
— |
— |
0.003 |
0.009 |
0.304 |
1.01 |
78Se |
— |
— |
0.922 |
3.08 |
0.326 |
1.09 |
— |
— |
— |
— |
0.011 |
0.036 |
0.666 |
2.22 |
95Mo |
0.054 |
0.181 |
1.05 |
3.48 |
7.40 |
24.7 |
— |
![[thin space (1/6-em)]](https://www.rsc.org/images/entities/char_2009.gif) |
0.284 |
0.948 |
— |
— |
— |
— |
107Ag |
0.003 |
0.009 |
0.672 |
2.24 |
1.45 |
4.84 |
0.014 |
0.045 |
0.603 |
2.01 |
— |
— |
— |
— |
111Cd |
0.004 |
0.014 |
0.030 |
0.098 |
0.081 |
0.270 |
0.001 |
0.003 |
0.091 |
0.304 |
— |
— |
8.68 |
28.9 |
121Sb |
0.105 |
0.349 |
0.127 |
0.423 |
0.337 |
1.12 |
— |
— |
— |
— |
— |
— |
— |
— |
137Ba |
0.006 |
0.021 |
2.09 |
6.96 |
0.874 |
2.91 |
0.022 |
0.074 |
— |
— |
— |
— |
— |
— |
205Tl |
0.003 |
0.009 |
0.024 |
0.081 |
0.024 |
0.079 |
0.001 |
0.004 |
0.012 |
0.041 |
— |
— |
— |
— |
207Pb |
0.003 |
0.011 |
0.612 |
2.04 |
0.345 |
1.15 |
0.004 |
0.013 |
— |
— |
— |
— |
— |
— |
208Pb |
— |
— |
1.54 |
5.12 |
0.356 |
1.19 |
— |
— |
0.828 |
2.76 |
— |
— |
2.02 |
6.72 |
Multi-element analysis of water reference material by FI-ICP-TOFMS
Multi-element determination was first carried out in a TM-28.4 Ontario Lake water reference material using flow injection in combination with ICP-TOFMS and ICP-MS/MS detection. A significant advantage of flow injection is the analysis of sample volumes down to the μL range instead of typically mL-sample volumes. The analyzed water reference material contains elements matching the concentration usually found in lake water. An overview of the results for TM-28.4 water reference material using ICP-TOFMS and ICP-MS/MS is given in Table 3. The calibration range covered two orders of magnitude and the used integration time of 150 ms resulted in four orders of magnitude of dynamic range.
Table 3 Multi-element determination in TM-28.4 Ontario Lake water reference material using flow injection with a sample intake of 5 μL in combination with ICP-TOFMS detection in standard operation mode and in H2/He gas mode, and with ICP-MS/MS detection in standard mode and in oxygen gas mode, for n = 5 independently prepared samples, respectively
|
Target value [μg L−1] |
ICP-TOFMS – standard mode |
ICP-TOFMS – H2/He gas mode |
ICP-MS/MS – standard mode |
ICP-MS/MS – O2 gas mode |
Concentration [μg L−1] |
RSD [%] |
Recovery [%] |
Concentration [μg L−1] |
RSD [%] |
Recovery [%] |
Concentration [μg L−1] |
RSD [%] |
Recovery [%] |
Concentration [μg L−1] |
RSD [%] |
Recovery [%] |
27Al |
54.2 ± 6.2 |
48.6 ± 4.4 |
9.0 |
90 |
46.3 ± 1.1 |
2.3 |
85 |
40.9 ± 1.6 |
3.9 |
75 |
52.3 ± 2.8 |
5.3 |
96 |
47Ti |
8.10 ± 0.69 |
7.60 ± 0.19 |
2.5 |
94 |
<LOQ |
— |
— |
— |
— |
— |
8.36 ± 0.18 |
2.1 |
103 |
51V |
3.17 ± 0.31 |
2.72 ± 0.10 |
3.8 |
86 |
3.00 ± 0.05 |
1.7 |
95 |
3.37 ± 0.09 |
2.7 |
106 |
— |
— |
— |
52Cr |
4.90 ± 0.37 |
4.47 ± 0.21 |
4.8 |
91 |
4.90 ± 0.11 |
2.3 |
100 |
4.43 ± 0.53 |
11.9 |
91 |
— |
— |
— |
55Mn |
7.00 ± 0.44 |
6.53 ± 0.76 |
11.7 |
93 |
7.20 ± 0.12 |
1.7 |
103 |
6.81 ± 0.49 |
7.2 |
97 |
— |
— |
— |
56Fe |
17.8 ± 3.0 |
<LOQ |
— |
— |
15.0 ± 0.3 |
2.2 |
84 |
— |
— |
— |
15.5 ± 1.5 |
9.6 |
87 |
59Co |
3.53 ± 0.39 |
3.44 ± 0.02 |
0.50 |
97 |
3.56 ± 0.06 |
1.7 |
101 |
3.53 ± 0.06 |
1.8 |
100 |
— |
— |
— |
60Ni |
9.90 ± 0.92 |
9.57 ± 0.38 |
4.0 |
97 |
9.89 ± 0.09 |
0.95 |
100 |
9.25 ± 0.20 |
2.1 |
93 |
— |
— |
— |
63Cu |
6.51 ± 0.72 |
9.59 ± 0.87 |
9.1 |
147 |
15.7 ± 0.2 |
1.5 |
242 |
<LOQ |
— |
— |
— |
— |
— |
66Zn |
29.6 ± 3.6 |
39.9 ± 0.50 |
1.3 |
135 |
34.9 ± 0.6 |
1.8 |
118 |
34.9 ± 2.6 |
7.3 |
118 |
<LOQ |
— |
— |
75As |
6.30 ± 0.53 |
6.34 ± 0.10 |
1.6 |
101 |
6.64 ± 0.14 |
2.1 |
105 |
— |
— |
— |
7.02 ± 0.36 |
5.2 |
111 |
78Se |
4.53 ± 0.63 |
4.66 ± 0.17 |
3.7 |
103 |
4.76 ± 0.11 |
2.2 |
105 |
— |
— |
— |
4.58 ± 0.44 |
9.5 |
101 |
95Mo |
3.75 ± 0.84 |
<LOQ |
— |
— |
<LOQ |
— |
— |
1.07 ± 0.10 |
9.0 |
28 |
— |
— |
— |
107Ag |
3.85 ± 0.33 |
3.01 ± 0.22 |
7.3 |
78 |
5.56 ± 0.30 |
5.4 |
144 |
10.2 ± 0.5 |
4.6 |
264 |
— |
— |
— |
111Cd |
1.90 ± 0.15 |
1.85 ± 0.08 |
4.3 |
97 |
1.91 ± 0.05 |
2.6 |
101 |
2.14 ± 0.04 |
1.9 |
113 |
— |
— |
— |
121Sb |
3.40 ± 0.30 |
3.29 ± 0.09 |
2.6 |
97 |
3.57 ± 0.05 |
1.4 |
105 |
— |
— |
— |
— |
— |
— |
138Ba |
16.1 ± 1.1 |
14.4 ± 0.65 |
4.5 |
90 |
19.0 ± 0.2 |
1.2 |
118 |
— |
— |
— |
— |
— |
— |
205Tl |
3.92 ± 0.35 |
3.68 ± 0.24 |
6.5 |
94 |
3.88 ± 0.04 |
1.1 |
99 |
4.29 ± 0.07 |
1.6 |
109 |
<LOQ |
— |
— |
208Pb |
4.34 ± 0.38 |
<LOQ |
— |
— |
4.53 ± 0.09 |
2.0 |
104 |
4.26 ± 0.30 |
7.1 |
98 |
<LOQ |
— |
— |
According to Table 3, the FI-ICP-TOFMS method provided an accurate quantification of a wide range of elements in the water TM-28.4 reference material, including 27Al, 47Ti, 51V, 52Cr, 55Mn, 56Fe, 59Co, 60Ni, 75As, 78Se, 111Cd, 121Sb, 205Tl and 208Pb. Copper, zinc and silver concentrations could not be accurately determined by ICP-TOFMS. The use of the CCT mode where the collision/reaction cell is pressurized with a mixture of H2/He gas improved the precision of the flow injection measurements. For most elements no pronounced difference between quantification accuracy under standard conditions and in CCT mode were found. This was also reported in a study using LA-ICP-TOFMS with pressurized and unpressurized cell in NIST SRM 612 glass analysis.26 A positive impact of the CCT mode was observed on the limit of quantification of iron, allowing its accurate quantification in the analyzed water reference material (at a concentration of about 18 μg L−1) by measuring the 56Fe isotope.
Most of the element concentrations certified in the lake water TM-28.4 reference material were validated by ICP-TOFMS analysis using single injections of independently prepared samples. Flow injection experiments in combination with ICP-MS/MS detection required at least the use of two different modes (standard mode and O2 gas mode) and consequently the use of two sample injections to achieve a similar performance resulting in increased analysis time. In the tandem quadrupole ICP-MS/MS configuration the transition from one gas mode to another requires between 10–20 s, whereas the transient signal delivered by flow injection was approximately 6–8 s. Typical profiles of transient signals of different isotopes of the lake water reference material are shown in Fig. 1. Using ICP-MS/MS detection in standard mode, the less interfered isotopes 51V, 52Cr, 55Mn, 59Co, 60Ni, 111Cd, 205Tl and 208Pb were accurately quantified in 5 μL of water TM-28.4 reference material. For the accurate quantification of the isotopes 27Al, 47Ti, 56Fe, 75As and 78Se the oxygen gas mode using mass shift modality was required. Copper and zinc concentrations could neither be accurately determined in water TM-28.4 reference material using ICP-MS/MS in standard mode nor when the collision/reaction cell was pressurized with O2. Therefore, the use of different gases such as He, H2, NH3etc. would be required for interference removal.3
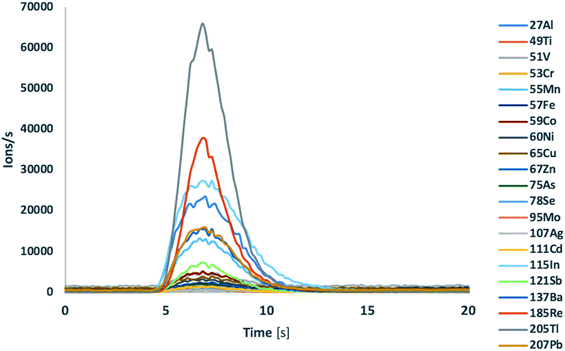 |
| Fig. 1 Transient signal of different isotopes of lake water TM-28.4 reference material after flow injection ICP-TOFMS analysis. | |
Multi-element analysis of serum reference material by FI-ICP-TOFMS
In a second investigation, the capabilities of flow injection ICP-TOFMS for accurate multi-element determination were evaluated for increased matrix complexity by analyzing a serum reference material. The flow injection experiments were based on a sample intake of 5 μL without prior mineralization of the sample material, thereby minimizing the sample preparation and enabling a high-throughput method for biological samples. External calibration was based on matrix-matched standards using serum Seronorm reference material in sodium chloride solution mimicking the salt concentration and thus the resulting matrix interferences in serum sample analysis. The target values of the serum Seronorm reference material were used to set up calibration curves resulting in linearity of >0.99 for most analytes. The results for the flow injection ICP-TOFMS and ICP-MS/MS method are summarized in Table 4 and concentrations are given for elements that are certified in both serum reference materials.
Table 4 Multi-element determination in serum ClinCheck reference material using flow injection with a sample intake of 5 μL in combination with ICP-TOFMS detection in standard operation mode, and ICP-MS/MS detection in standard mode and in oxygen gas mode, for n = 5 independently prepared samples, respectively
|
Target value [μg L−1] |
ICP-TOFMS – standard mode |
ICP-MS/MS – standard mode |
ICP-MS/MS – O2 gas mode |
Concentration [μg L−1] |
RSD [%] |
Recovery [%] |
Concentration [μg L−1] |
RSD [%] |
Recovery [%] |
Concentration [μg L−1] |
RSD [%] |
Recovery [%] |
24Mg |
12 400 ± 1200 |
12 080 ± 110 |
0.91 |
97.4 |
11 799 ± 125 |
1.1 |
95.2 |
11 973 ± 521 |
4.4 |
96.5 |
27Al |
22.7 ± 6.8 |
<LOQ |
— |
— |
21.8 ± 3.0 |
13.9 |
95.8 |
26.0 ± 3.3 |
12.6 |
114 |
54Fe |
719 ± 108 |
751 ± 13 |
1.8 |
105 |
— |
— |
— |
— |
— |
— |
56Fe |
719 ± 108 |
759 ± 2 |
0.29 |
106 |
777 ± 25 |
3.2 |
108 |
708 ± 65 |
9.2 |
98.5 |
57Fe |
719 ± 108 |
812 ± 13 |
1.6 |
113 |
— |
— |
— |
720 ± 73 |
10.1 |
100 |
58Fe |
719 ± 108 |
672 ± 10 |
1.5 |
93.5 |
— |
— |
— |
— |
— |
— |
59Co |
1.74 ± 0.35 |
1.87 ± 0.04 |
2.02 |
108 |
1.92 ± 0.04 |
1.9 |
110 |
— |
— |
— |
61Ni |
4.0 ± 0.8 |
3.89 ± 0.48 |
12.3 |
97.3 |
— |
— |
— |
— |
— |
— |
63Cu |
801 ± 120 |
760 ± 24 |
3.1 |
94.9 |
792 ± 22 |
2.8 |
98.9 |
— |
— |
— |
65Cu |
801 ± 120 |
764 ± 23 |
3.0 |
95.3 |
817 ± 12 |
1.5 |
102 |
801 ± 120 |
15.0 |
97.9 |
64Zn |
1320 ± 200 |
1124 ± 37 |
3.3 |
85.1 |
— |
— |
— |
|
|
|
66Zn |
1320 ± 200 |
1132 ± 34 |
3.0 |
85.8 |
1169 ± 53 |
4.5 |
88.5 |
1127 ± 65 |
5.7 |
85.4 |
67Zn |
1320 ± 200 |
1122 ± 34 |
3.0 |
85.0 |
— |
— |
— |
— |
— |
— |
68Zn |
1320 ± 200 |
1142 ± 37 |
3.0 |
86.5 |
— |
— |
— |
— |
— |
— |
70Zn |
1320 ± 200 |
1137 ± 42 |
3.7 |
86.1 |
— |
— |
— |
— |
— |
— |
78Se |
66.1 ± 13.2 |
64.9 ± 2.4 |
3.7 |
98.3 |
71.6 ± 2.0 |
2.8 |
108 |
69.2 ± 2.9 |
4.3 |
105 |
82Se |
66.1 ± 13.2 |
74.2 ± 2.19 |
2.9 |
112 |
— |
— |
— |
— |
— |
— |
111Cd |
2.28 ± 0.46 |
2.72 ± 0.05 |
1.9 |
119 |
2.53 ± 0.05 |
2.0 |
111 |
— |
— |
— |
The concentrations of elements with major biological function such as magnesium, iron, cobalt, nickel, copper, zinc, selenium and cadmium were successfully validated by FI-ICP-TOFMS analysis of serum reference material, proving that the method is fit-for-purpose for serum sample analysis. Only aluminum in the serum ClinCheck reference material was below the limit of quantification of FI-ICP-TOF-MS. Using ICP-TOFMS measurements and data post-processing strategies including base-line subtraction and peak deconvolution, it was possible to accurately quantify all iron, copper and zinc isotopes at biologically relevant concentrations in the serum reference material. This demonstrates that also isotope dilution strategies can be employed with ICP-TOFMS for quantification of these elements in serum samples. Also for selenium, ICP-TOFMS proved to be suitable to accurately quantify the isotopes 78Se and 82Se in serum.
Using the flow injection approach, the serum sample intake and the sample preparation time is significantly reduced compared to the established trace element analysis procedures, relying on mineralization of serum samples (with sample intakes between 200 μL and 1 mL) and subsequent dilution to achieve enough volume for analysis.9,10 For FI-ICP-MS/MS analysis, the instrument was operated in standard mode or using collision/reaction cell pressurized with O2. Accordingly, again two injections per sample were required for accurate quantification resulting in increase of measurement time. Generally, multi-element analysis using ICP-MS/MS with collision/reaction cell technology involves more efforts regarding method optimization, requiring a careful selection of the gas mode for different analytes, which in turn is facilitated by a priori knowledge about the best suited isotope/isotopes of an element, the best suited gas mode, the sample composition and thus possible interferences due to the matrix. The use of ICP-TOF-MS in contrast does not require prior knowledge about the sample matrix and possible interferences or the choice of the best-suited isotope as elements from the entire mass range are measured for every sample.
Conclusions
This work demonstrates the capabilities of flow injection ICP-TOFMS for accurate multi-element quantification in lake water and serum reference material using a sample intake of 5 μL. The use of the CCT with a H2/He gas mixture improved the limits of quantification and precision for most elements. The quantification accuracy for the elements considered was shown to be unaffected by the use of the CCT but for iron it proved to be beneficial for its quantification at the concentration present in lake water reference material. A wide range of elements at trace element concentrations was accurately quantified in the used reference materials by the developed FI-ICP-TOFMS method and it was also concluded that the proposed method is fit-for-purpose for isotope dilution strategies for iron, copper and zinc in serum samples. FI-ICP-TOFMS analysis proved to be superior in terms of sample throughput compared to FI-ICP-MS/MS analysis where two sample injections using two different gas modes are required for multi-element analysis for a short transient signal. The use of ICP-TOFMS reduces the need for pre-analytical literature research to define study-specific target elements and possible interferences resulting from the matrix. ICP-TOFMS analysis provides a holistic approach which is fit-for-purpose for multi-element profiling in environmental and biological matrices and thus enables the application of the proposed methodology to e.g. clinical studies where a high number of samples needs to be analysed and limited sample volume is available.
Conflicts of interest
There are no conflicts to declare.
Acknowledgements
The authors acknowledge the European ReMiND project (nr: 15HLT02) for funding. The authors thank Olga Borovinskaya, Martin Tanner and Mike Cubison for their help and advice on how to optimize and run the icpTOF as well as how to use Tofware for data post-processing.
References
- C. E. Cicero, G. Mostile, R. Vasta, V. Rapisarda, S. S. Signorelli, M. Ferrante, M. Zappia and A. Nicoletti, Environ. Res., 2017, 159, 82–94 CrossRef CAS PubMed.
- S. D. Tanner, V. I. Baranov and D. R. Bandura, Spectrochim. Acta, Part B, 2002, 57, 1361–1452 CrossRef.
- E. Bolea-Fernandez, L. Balcaen, M. Resano and F. Vanhaecke, J. Anal. At. Spectrom., 2017, 32, 1660–1679 RSC.
- L. Balcaen, E. Bolea-Fernandez, M. Resano and F. Vanhaecke, Anal. Chim. Acta, 2015, 894, 7–19 CrossRef CAS.
- L. Balcaen, G. Woods, M. Resano and F. Vanhaecke, J. Anal. At. Spectrom., 2013, 28, 33–39 RSC.
- L. Balcaen, E. Bolea-Fernandez, M. Resano and F. Vanhaecke, Anal. Chim. Acta, 2014, 809, 1–8 CrossRef CAS PubMed.
- E. Bolea-Fernandez, L. Balcaen, M. Resano and F. Vanhaecke, Anal. Bioanal. Chem., 2015, 407, 919–929 CrossRef CAS PubMed.
- L. Bamonti, S. Theiner, N. Rohr-Udilova, B. K. Keppler and G. Koellensperger, J. Anal. At. Spectrom., 2016, 31, 2227–2232 RSC.
- T. Konz, E. Migliavacca, L. Dayon, G. Bowman, A. Oikonomidi, J. Popp and S. Rezzi, J. Proteome Res., 2017, 16, 2080–2090 CrossRef CAS PubMed.
- S. Meyer, M. Markova, G. Pohl, T. A. Marschall, O. Pivovarova, A. F. H. Pfeiffer and T. Schwerdtle, J. Trace Elem. Med. Biol., 2018, 49, 157–163 CrossRef CAS PubMed.
- C. Engelhard, Anal. Bioanal. Chem., 2011, 399, 213–219 CrossRef CAS PubMed.
- M. Tanner and D. Günther, Anal. Chim. Acta, 2009, 633, 19–28 CrossRef CAS PubMed.
- M. Vázquez Peláez, J. M. Costa-Fernández and A. Sanz-Medel, J. Anal. At. Spectrom., 2002, 17, 950–957 RSC.
- D. R. Bandura, V. I. Baranov, O. I. Ornatsky, A. Antonov, R. Kinach, X. Lou, S. Pavlov, S. Vorobiev, J. E. Dick and S. D. Tanner, Anal. Chem., 2009, 81, 6813–6822 CrossRef CAS PubMed.
- C. Giesen, H. A. O. Wang, D. Schapiro, N. Zivanovic, A. Jacobs, B. Hattendorf, P. J. Schüffler, D. Grolimund, J. M. Buhmann, S. Brandt, Z. Varga, P. J. Wild, D. Günther and B. Bodenmiller, Nat. Methods, 2014, 11, 417–422 CrossRef CAS.
- O. Borovinskaya, B. Hattendorf, M. Tanner, S. Gschwind and D. Günther, J. Anal. At. Spectrom., 2013, 28, 226–233 RSC.
- S. J. M. Van Malderen, A. J. Managh, B. L. Sharp and F. Vanhaecke, J. Anal. At. Spectrom., 2016, 31, 423–439 RSC.
- A. Gundlach-Graham and D. Günther, Anal. Bioanal. Chem., 2016, 408, 2687–2695 CrossRef CAS PubMed.
- A.-L. Ronzani, F. Pointurier, M. Rittner, O. Borovinskaya, M. Tanner, A. Hubert, A.-C. Humbert, J. Aupiais and N. Dacheux, J. Anal. At. Spectrom., 2018, 33, 1892–1902 RSC.
- L. Mueller, H. Traub, N. Jakubowski, D. Drescher, V. I. Baranov and J. Kneipp, Anal. Bioanal. Chem., 2014, 406, 6963–6977 CrossRef CAS PubMed.
- O. Borovinskaya, S. Gschwind, B. Hattendorf, M. Tanner and D. Günther, Anal. Chem., 2014, 86, 8142–8148 CrossRef CAS PubMed.
- M. D. Montaño, J. W. Olesik, A. G. Barber, K. Challis and J. F. Ranville, Anal. Bioanal. Chem., 2016, 408, 5053–5074 CrossRef PubMed.
- L. Hendriks, A. Gundlach-Graham and D. Günther, CHIMIA, 2018, 72, 221–226 CrossRef CAS PubMed.
- L. Hendriks, A. Gundlach-Graham, B. Hattendorf and D. Günther, J. Anal. At. Spectrom., 2017, 32, 548–561 RSC.
- M. Burger, G. Schwarz, A. Gundlach-Graham, D. Käser, B. Hattendorf and D. Günther, J. Anal. At. Spectrom., 2017, 32, 1946–1959 RSC.
- M. Burger, L. Hendriks, J. Kaeslin, A. Gundlach-Graham, B. Hattendorf and D. Guenther, J. Anal. At. Spectrom., 2019, 34, 135–146 RSC.
Footnote |
† These authors contributed equally to the manuscript. |
|
This journal is © The Royal Society of Chemistry 2019 |