DOI:
10.1039/C9FO00353C
(Paper)
Food Funct., 2019,
10, 6492-6502
Optimization of extraction methods for characterization of phenolic compounds in apricot fruit (Prunus armeniaca)†
Received
20th February 2019
, Accepted 2nd July 2019
First published on 19th September 2019
Abstract
Fruits are rich in phenolic compounds with health-promoting activities. However, phenolic profiles vary between fruits. Hence, specific extraction methods are required for accurate profiling of the functional compounds. This study aims to develop an optimised method by response surface methodology to extract phenolics from apricots (Prunus armeniaca) and correctly characterise apricots’ phenolic profile. For this, the effects of the solid-to-liquid ratio, temperature, extraction solvent, extraction time and sequential extraction steps on the extraction of major phenolic families were investigated. Methanol- and ethanol-based extractions were suitable, although methanol was the optimal solvent. The optimised extraction conditions were 20 g mL−1, 38 °C and 72% methanol (1% formic acid). When this method was used in apricots, the characterisation of their phenolic profile by HPLC-ESI-MS/MS showed a higher extraction of phenolic compounds than other studies in the literature that use non-specific extraction methods. The developed method is fast and economically feasible for accurate characterisation of the phenolic profile of apricot fruits and thus can be routinely used to extract apricot phenolic compounds for their characterisation.
1. Introduction
Diets rich in fruits and vegetables are associated with a beneficial role in several human diseases, and these benefits are attributed to the phenolic content of plants.1 Indeed, in recent years, phenolic compounds have attracted interest due to their beneficial health effects. Different phenolic compounds have been reported to exhibit different health-promoting activities.2 In addition, different fruits have different phenolic profiles.3–7 Therefore, their potential roles in human health differ.
In this sense, it is important to note that when characterising the phenolic profile of a food matrix, only compounds that are extracted can be quantified. Given the diversity of food matrixes and phenolic profiles of foods, specific methods that fully extract fruit phenolics should be developed. This is essential to correlate the consumption of a mixture of phenolics with a beneficial health effect. Factors such as the liquid-to-solid ratio (LSR), type of solvent, temperature and extraction time can greatly influence phenolic extraction from food matrixes.8–12 In systems where several factors can affect the output, response surface methodology (RSM) is a useful tool to optimise extraction processes. The primary advantage of this approach is the evaluation of the effect of multiple variables and their interactions on the output variables with a reduced number of trials.13–15 Indeed, RSM has been widely used to optimise the extraction of phenolic compounds from various plant materials and fruits.3–5,7,11–21
Apricots are one of the dietary sources with the highest polyphenol content22 and can be considered as natural functional food. Indeed, apricot consumption is associated with several health effects, including hepatoprotective, anti-inflammatory and anti-hypertensive effects, among others.23 The phenolic content in apricots can vary between varieties24–27 and depends on maturity28 and the region and system of cultivation.27,29 Nevertheless, apricots have been widely reported to be rich in flavonoids, specifically flavanols and flavonols, and non-flavonoid phenolics, specifically hydroxycinnamic derivatives.26,28 In apricots, flavonols occur largely as quercetin and kaempferol glucosides, quercetin-3-O-rutinoside (rutin) being their main component.23,28 Flavanols occur mainly as (+)-catechin and (−)-epicatechin, one or the other being the predominant form depending on the apricot variety.26 In addition, several dimeric flavanol forms can accumulate up to significant levels.25,28 Among the hydroxycinnamic derivatives, chlorogenic acid is widely reported as the main compound.28 Neochlorogenic acid, p-coumaric acid, caffeic acid and gallic acid can also be present in different apricot cultivars.26,28 In addition, other phenolics from different families, such as resveratrol, can also occur in small quantities.25,29
The phenolic composition of apricots has been widely determined by using different extraction methods,24,25,30 non-specific for apricots. For instance, many of the studies that evaluate phenolic compounds in apricots use adaptations of the method described by Bengoechea et al.,26–29,31,32 which was developed for the extraction of phenolic compounds from peach and apple purees and concentrates.6 Importantly, the selection of one extraction method over another has crucial effects on the extraction of phenolic compounds and thus the characterisation of the food matrix.10,33 However, so far, specific methods that aim to focus on the full extraction of all relevant phenolic families from apricots are lacking. In this sense, Zitka et al.10 evaluated the effect of some extraction factors (i.e. solvent and temperature) on polyphenol extraction from apricots, but this study was centred on the extraction of particular apricot phenolic compounds. Similarly, other authors have developed optimized methods to extract apricot polyphenols, not from the fresh apricot matrix, but from processed apricots,25 sun dried apricots,5 and apricot waste.34 For example, Erdoan et al. evaluated the effect of some extraction variables (i.e. solvent, pressure, time and temperature) by the one-variable-at-a-time approach in an accelerated solvent extraction system for the total polyphenols in processed apricots.25 In the same line, Cheaib et al. studied the effect of certain extraction variables (i.e. temperature and solvent) on the extraction of the total phenolic, flavonoid and tannin contents from apricot pomace (pressed skins and pulp residues).34 As for sun-dried apricots, Wani et al. optimised several parameters (i.e. temperature and solvent) to extract the highest polyphenol content with the highest anti-oxidant activity by an RSM approach.5 However, none of the mentioned studies developed a specific method to extract the maximum content of the most representative families in apricots by an RSM approach. Importantly, beneficial health effects of apricots are mainly associated with the consumption of fresh fruit, and thus full characterization of the phenolic composition of apricot fruits is required to identify the involved bioactive compounds. Hence, the primary aim of this study is to develop an apricot-specific phenolic compound extraction methodology that allows accurate characterisation of the apricot fruit phenolic profile. To do so, an RSM was applied, and extracts were analysed by HPLC-ESI-MS/M. The development of this methodology is novel as it is the first approach to develop a specific phenolic compound extraction methodology that aims to extract all the phenolic families from apricots, and this will be useful to completely characterise the phenolic profile in detail for bioactivity, comparison or any other studies.
2. Experimental
2.1. Plant material
Apricots (Prunus armeniaca, Charisma variety) were purchased from Mercabarna (Barcelona, Spain). Apricot stones were manually removed and discarded. Apricots (peel and flesh) were first frozen in liquid nitrogen and ground. Next, homogenates were lyophilised for a week in a Telstar LyoQuest lyophilizer (Thermo Fisher Scientific, Madrid, Spain) at −85 °C and further ground to obtain a fine powder. The apricot powder was kept dry and protected from humidity and light exposure until extraction.
2.2. Chemicals and reagents
Acetonitrile, methanol, ethanol (HPLC analytical grade) and glacial acetic acid were purchased from Panreac (Barcelona, Spain). Ultrapure water was obtained from a Milli-Q Advantage A10 system (Madrid, Spain). Formic acid was purchased from Scharlab (Barcelona, Spain). The Folin–Ciocalteu and p-dimethylaminocinnamaldehyde (DMACA) reagents were purchased from Fluka/Sigma-Aldrich (Madrid, Spain). Chlorogenic acid, eriodictyol, eriodyctiol-7-O-glucoside, hyperoside (quercetin-3-O-galactoside), isorhamnetin-3-O-glucoside, kaempferol, kaempferol-3-O-glucoside, and kaempferol-3-O-rutinoside were purchased from Extrasynthese (Lyon, France), and benzoic acid, caffeic acid, (+)-catechin, epigallocatechin gallate (EGCG), p-coumaric acid, (−)-epicatechin, ferulic acid, gallic acid, procyanidin dimer B2, protocatechuic acid and quercetin were purchased from Fluka/Sigma-Aldrich (Madrid, Spain). Resveratrol was purchased from Quimivita (Barcelona, Spain) and rutin was kindly provided by Nutrafur (Murcia, Spain).
All standard compounds were individually dissolved in methanol at 2000 mg L−1, with the exception of isorhamnetin-3-O-glucoside, dissolved at 1000 mg L−1, and hyperoside, dissolved at 500 mg L−1. All standard stock solutions were newly prepared every 3 months and stored in amber-glass flasks at −20 °C. Mixed standard stock solutions were prepared with Milli-Q water to obtain the concentration needed to construct the calibration curves.
2.3. Extraction procedure
Apricot powder was weighed to obtain the desired LSR and mixed with 1 mL of pre-heated extraction solvent (methanol
:
water, v
:
v). Different methanol proportions, temperatures, times and extraction steps were used throughout the experiment. In addition, methanol was prepared in all cases including 1% formic acid. Extractions were performed at 500 rpm agitation under protection from light exposure. Samples were centrifuged at 9500g for 10 min at 4 °C, and supernatants were stored at −20 °C until further analysis.
2.4. Single factor studies
Prior to RSM, a first set of tests were performed to select experimental ranges for independent variables. Individual effects of the LSR, methanol concentration and temperature were evaluated based on the total phenolic content (TPC), total flavonol content (TFoC), tartaric ester content (TEC) and total flavanol content (TFaC) as the major phenolic families present in apricots.26,28,29 To select the RSM ranges of the extraction variables different sets of extractions were performed: as for the LSR, this extraction variable was tested at 10, 20, 40, 60 and 80 mL g−1; as for temperature, this variable was tested at 25, 40, 55, 70, and 85°; and as for methanol percentage, this variable was tested at 30, 50, 60, 70 and 90%. The LSR, temperature and methanol percentage were kept constant at 20 mL g−1, 55 °C, and 50% when not under study. All extractions lasted for 30 min as this extraction time is considered to be neither too long to promote phenolic degradation nor too short to impede phenolic solubilisation into the solvent as reported in other studies.4,5,11–13,15
2.5. Surface response design
The extraction was optimised by using an experimental design by RSM. A face-centered central composite design with two factors and three levels, consisting of 11 randomised runs with 3 centre points, was selected. Independent variables used in the RSM were temperature (25–55 °C, Xi) and methanol proportion (60–100%, Xj). The LSR (20 mL g−1) and extraction time (30 min) were fixed as constant variables during the RSM experiment. Experimental data were fitted to a second polynomial response surface, which follows the equation |  | (1) |
where Y is a dependent variable, β0 is the constant coefficient, and βi, βii and βij are the linear, quadratic and interaction regression coefficients, respectively. Xi, Xii and Xji represent independent variables.
Individual phenolic compounds were quantified by the HPLC-DAD method and used in the RSM optimisation study. The results of the RSM design were analysed with Design-Expert 9.0.6 software (Trial version, Stat-Ease Inc., Minneapolis, MN, USA). Single parameters that were not influenced by the extraction factors were omitted in the model.
2.6. Kinetic study
A kinetic study was performed to evaluate the effect of time on the polyphenol extraction yield. Seven extraction times, ranging from 0 to 120 min, were selected. The LSR was fixed at 20 mL g−1, methanol percentage at 72% and temperature at 38 °C. TPC, TFoC, TEC and TFaC were determined to evaluate the effect of time on the polyphenol extraction.
2.7. Sequential extractions
Three consecutive extractions were performed to evaluate the influence of multiple extractions on the polyphenol extraction yield. The LSR was fixed at 20 mL g−1, methanol percentage at 72% and temperature at 38 °C. Samples were mixed with the solvent, vortexed and centrifuged (9500g, 10 min, 4 °C). TPC, TFoC, TEC and TFaC were determined to evaluate the effect of sequential extractions on the polyphenol extraction yield.
2.8. Methanol–ethanol comparison
To evaluate the efficiency of ethanol in polyphenol extraction, apricot powder was extracted twice under the following conditions: LSR of 20 mL g−1, methanol or ethanol (EtOH) proportion of 72% (1% formic acid), temperature of 38 °C. Samples were mixed with the solvent, vortexed and centrifuged (9500g, 10 min, 4 °C). The TPC, TFaC, TEC and TFoC were determined to evaluate the efficiency of ethanol in polyphenol extraction.
2.9. Analysis of response variables
2.9.1. Total phenolic content.
The TPC of extracts was determined by the Folin–Ciocalteu method adapted from Iglesias-Carres et al.11 Briefly, 50 μL of the extract and 50 μL of the Folin–Ciocalteu reagent were successively added to an Eppendorf tube containing 500 μl of Milli-Q water and mixed. After standing for 3 minutes in the dark, the samples received 100 μL of Na2CO3 (25%) and were brought to a final volume of 1 mL with Milli-Q water. Absorbance was read at 725 nm using an Eon BioTek spectrophotometer (Izasa, Barcelona, Spain) against a water sample (blank) that underwent equal treatment after 1 hour of incubation in the dark. Gallic acid was used to construct the calibration curve between 40 mg L−1 and 400 mg L−1. The results were expressed as milligrams of gallic acid equivalents per gram of dry weight (mg GAE per g dw).
2.9.2. Total flavonol and tartaric ester content.
The TFoC and TEC were determined with the method described by Cacace et al.3 Briefly, 250 μL of extracts were mixed with 250 μL of 0.1% HCl in ethanol 95% and 4.55 mL of 2% HCl and were allowed to react for 15 minutes. Absorbance was then read at 360 and 320 nm for flavanol and tartaric ester quantification, respectively, using an Eon BioTek spectrophotometer (Izasa, Barcelona, Spain). Quercetin and caffeic acid were used to construct calibration curves, and the results were expressed as milligrams of quercetin or caffeic acid equivalents per gram of dry weight (mg quercetin per g dw; mg caffeic acid per g dw).
2.9.3. Total flavanol content.
The TFaC of extracts was estimated by the DMACA method.35 Briefly, 100 μL of extract samples were mixed with 500 μL of DMACA solution (0.1% 1 N HCl in methanol) and allowed to react at room temperature for 10 min while protected from light exposure. Next, absorbance was read at 640 nm using an Eon BioTek spectrophotometer (Izasa, Barcelona, Spain). Catechin concentrations between 5 mg L−1 and 100 mg L−1 were used to construct a calibration curve. The TFaC was expressed as milligrams of catechin equivalents per gram of dry weight (mg catechin per g dw).
2.9.4. HPLC-DAD method.
Polyphenol separation was achieved using a ZORBAX Eclipse XDB-C18 (150 mm × 2.1 mm i.d., 5 μm particle size) as the chromatographic column (Agilent Technologies, Palo Alto, CA, USA) equipped with a Narrow-Bore guard column (2.1 mm × 12.5 mm, 5 μm particle size). The mobile phase was water
:
acetic acid (99.8
:
0.2, v
:
v) (A) and acetonitrile (B) in a gradient mode as follows: initial conditions 0% B; 0–30% B, 0–18 min; 30–100% B, 18–19 min; 100% B isocratic, 19–20 min; 100–0% B, 20–21 min. A post-run of 6 min was required for column re-equilibration. The flow rate was set at 0.5 mL min−1, and the injection volume was 10 μL for all runs.
Identification and quantification of phenolic compounds of interest was achieved with a UV/ViS photodiode array detector (1260 Infinity, Agilent Technologies, Palo Alto, CA, USA). Chromatograms were recorded from 200 to 600 nm. Catechin was detected at 280 nm, chlorogenic acid at 320 nm, and rutin at 340 nm. The results were expressed as micrograms of equivalents per gram of dry weight (mg per g dw).
Calibration curves, linearity, intraday variability (precision), interday variability (reproducibility), detection and quantification limits were calculated in mobile phase A spiked with polyphenol standards (ESI Table 1†). The peak areas of various concentrations of standards were used to construct calibration curves. The method precision was calculated as the relative standard deviation (% RSD) of the concentration in a triplicate analysis of three different spiked samples (100, 50 and 1 μg mL−1). Method reproducibility was calculated as the relative standard deviations (% RSD) of three different standard compound concentrations (100, 50 and 1 μg mL−1) analysed in triplicate over three consecutive days. Sensitivity was evaluated by determining the limits of detection (LOD) and quantification (LOQ), which were respectively defined as the concentration corresponding to 3-fold and 10-fold of the signal-to-noise ratio.
2.9.5. HPLC-ESI-MS/MS method.
The extracts were directly analysed using a 1200 LC Series coupled to a 6410 MS/MS (Agilent Technologies, Palo Alto, CA, USA). The column and mobile phases used were the same as in the HPLC-DAD method (see section 2.9.4). The gradient mode was as follows: initial conditions 0% B; 0–0.5 min, 0% B; 0.5–2 min, 0–10% B; 2–12 min, 10–30% B; 12–16 min, 30–60% B; 16–17 min, 60–100% B, 17–20 min, 100% B; 20–21 min, 100–0% B. A post-run of 6 min was required for column re-equilibration. The flow rate was set at 0.4 mL min−1, and the injection volume was 2.5 μL for all runs. Electrospray ionization (ESI) was conducted at 200 °C and 14 l min−1 with 20 psi nebulizer gas pressure and 3000 V capillary voltage. The mass spectrometer was operated in the negative mode, and MS/MS data were acquired in dynamic mode. The optimized conditions for the analysis of the phenolic compounds studied using HPLC-ESI-MS/MS are summarized in ESI Table 2.† Data acquisition was carried out using MassHunter Software (Agilent Technologies, Palo Alto, CA, USA). The calibration curves, coefficient of determination, linearity and detection and quantification limits of the HPLC-ESI-MS/MS method can be found in ESI Table 3† and were evaluated following the same principles as in the HPLC-DAD method (see section 2.9.4).
2.10. Statistics
The results of the RSM design were analysed using Design-Expert 9.0.6 software (Trial version, Stat-Ease Inc., Minneapolis, MN, USA). SPSS 19 software (SPSS Inc., Chicago, IL, USA) was used for any other statistical analyses. All experiments were performed in triplicate; the statistics’ significance was evaluated using One-way ANOVA or Student's t-test; and p-values less than 0.05 were considered to be statistically significant.
3. Results and discussion
The phenolic content of apricots26–29,31,32 as well as the beneficial health effects23 has been largely studied. However, when apricot phenolics are analysed in the literature unspecific extraction methodologies are usually used. Importantly, extraction conditions can greatly affect the extraction yields of phenolic compounds5,8,11–13 and thus the characterization of a given matrix. For correct characterization of apricot phenolic composition, which is essential to link a specific polyphenol or a group of polyphenols with a particular health effect, an optimised method specific to extract apricot phenolics should be used. To develop a methodology as such, the extraction parameters affecting the extraction of phenolic compounds from apricots were evaluated and optimised.
3.1. Single factor studies
The effects of the LSR, temperature and methanol proportion on phenolic extraction were first evaluated individually (Fig. 1) to select a relevant variable range for the RSM study. This one-variable-at-a-time approach is useful to identify whether an extraction parameter affects the extraction of phenolic compounds from a given fruit matrix and its relevance.13 To evaluate the effect of these extraction parameters on the extraction of apricot phenolics, the TPC, TFoC, TEC and TFaC were chosen as dependent variables to give a global view of the extraction of the most representative phenolic families present in apricots.26,28,29 Extraction time is a relevant parameter in the extraction of phenolic compounds. The exposure to the optimal extraction solvent and temperature during a certain period of time results in a higher extraction rate.16,17 However, too long extraction times are not economically feasible and might also promote the degradation of extracted phenolic compounds.36 Thus, the extraction time was fixed at 30 min as this time is not too long or too short and has provided good results in the extraction of phenolic compounds from other food matrixes.4,5,11–13,15 Choosing an optimal LSR is key to the extraction of phenolics, since working at a low LSR may lead to solvent saturation7 and working at a high LSR is economically counterproductive. The TPC did not increase significantly at values above 20 mL g−1, whereas the TFoC, TEC and TFaC did not show any changes due to the LSR (Fig. 1a). Therefore, the LSR was fixed at 20 mL g−1 throughout the rest of the experiment. This LSR is very similar to the optimum in other plant matrixes,13,16,21 including sun-dried apricots.5
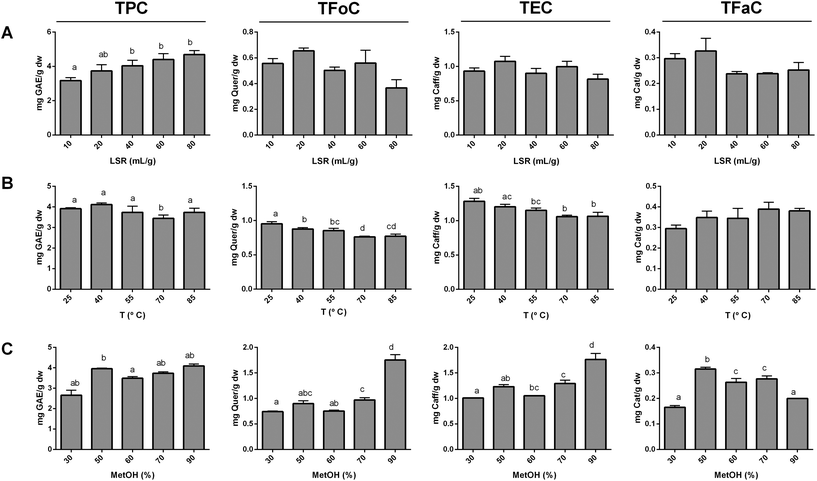 |
| Fig. 1 Individual effects of the liquid-to-solid ratio (LSR) (a), temperature (T) (b) and methanol (MeOH) percentage (c) on the extraction of apricot total phenolic content (TPC); total flavonol content (TFoC); tartaric ester content (TEC) and total flavanol content (TFaC). The results are expressed as mg of phenolic component per gram of dry weight (mg per g dw) ± SD (n = 3). a, b, c, d Mean values with different letters were significantly different between extraction conditions (one-way ANOVA with Tukey's post hoc test, p < 0.05). Abbreviations: caffeic acid (Caff), catechin (Cat), gallic acid equivalents (GAE), and quercetin (Quer). The figure was created with Graph-Pad PriSM 6.01 software (GraphPad, Sand Diego, CA, USA). | |
Temperature is a well-known factor that affects the extraction of phenolic compounds.4,11,13,37 In this sense, extraction temperature modulates several extraction parameters (i.e. solvent's viscosity and compound diffusion coefficient) which, in turn, modulate the extraction process.8,38 Moreover, temperature can also modulate phenolic extraction by promoting their chemical and enzymatic degradation.15 Indeed the degradation of phenolic compounds during extraction has been reported for several phenolic families at high extraction temperatures.3,8 Nevertheless, in this study, the TPC was poorly influenced by an increase of temperature whilst no effect was reported for TFaC extraction (Fig. 1b). Consistent with these findings, Gan et al. and Cacace et al. did not report a significant effect of temperature on the TPC extractability in Parkia speciosa pods and black currants, respectively.3,21 In our study, the TFoC and TEC decreased as temperature increased, and these decreases were higher above 55 °C. This contradicts the findings of the study of Van Der Sluis et al. on apple juice that demonstrated epicatechin to be more sensitive to temperature than chlorogenic acid.39 However, factors such as phenolic localisation in fruit tissues and interaction with the plant matrix play an important role in extraction,37 which could explain the effect observed for this specific fruit and phenolic families. Given our results, a range of temperatures between 25 and 55 °C was selected for the RSM study.
Throughout all experiments, methanol was always prepared at a formic acid concentration of 1%. Low concentrations of organic acids, such as formic acid, promote the degradation of the plant matrix, which enhances the extraction rate of phenolic compounds.40 The effect of methanol proportion on the TPC was not clear, which could be due to the wide variety of phenolic families present in apricots.28 However, the TFoC and TEC were found to be higher at 90%. Consistent with this finding, extractions with high proportions (80 and 100%) of acidified methanol are reported to be capable of extracting tartaric esters and flavonols from apricot fruits, jams and nectars.26,28,29 The TFaC was found to achieve the greatest extraction yield at 50% methanol, which is in line with the results obtained for flavonoids in Morinda citrifolia fruit.41 Given the few quantitative differences reported between 50 and 60% MeOH in the extraction of flavanols and the important differences in the extraction of flavonols and tartaric esters at higher proportions of methanol, a range of methanol proportions between 60 and 100% was selected for the RSM study.
3.2. Analysis of response surfaces
The extraction of apricot phenolics was optimised using an RSM approach. RSM approaches, unlike single factor studies, allow the evaluation of interaction effects between extraction parameters and can also predict an optimal extraction point that has to be verified experimentally.42 In this specific study, a face-centered central composite design with two factors and three levels was selected, consisting of 11 randomised runs with 3 centre points. The previously optimised LSR (20 mL g−1) and extraction time (30 min) were fixed as constant variables during the RSM experiment. The independent variables used in the RSM were methanol proportion (60–100%, Xj) and temperature (25–55 °C, Xi). The compounds catechin, chlorogenic acid and rutin were analysed individually by the HPLC-DAD method and were included in the RSM as relevant representatives of the phenolic families present in apricots.26,28,29 The obtained experimental extraction of catechin, chlorogenic acid and rutin for all runs is reported in Table 1.
Table 1 Face-centred settings of independent variables and experimental results of (+)-catechin, chlorogenic acid and rutin
Run |
T (°C) |
MeOH (%) |
Cat |
Chl |
Rut |
Abbreviations: Temperature (T); methanol (MeOH); (+)-catechin (Cat); chlorogenic acid (Chl); and rutin (Rut). The results are expressed as mg of phenolic component per gram of dry weight (mg per g dw) ± SD (n = 3). |
1 |
25 |
60 |
1.21 |
1.51 |
0.54 |
2 |
55 |
60 |
1.08 |
1.61 |
0.58 |
3 |
25 |
100 |
1.14 |
1.23 |
0.31 |
4 |
55 |
100 |
1.10 |
1.16 |
0.34 |
5 |
25 |
80 |
1.27 |
1.47 |
0.50 |
6 |
55 |
80 |
1.24 |
1.51 |
0.56 |
7 |
40 |
60 |
1.18 |
1.55 |
0.61 |
8 |
40 |
100 |
1.17 |
1.29 |
0.36 |
9 |
40 |
80 |
1.29 |
1.52 |
0.56 |
10 |
40 |
80 |
1.24 |
1.48 |
0.54 |
11 |
40 |
80 |
1.33 |
1.52 |
0.53 |
3.2.1. Multiple linear regressions and the model's adequacy.
The experimental data (Table 1) were used to determine the regression coefficients of eqn (1). All compounds analysed generated a significant model, implying that at least one of the extraction variables could explain the variation in the response variables (Table 2). Indeed, the coefficients of determination (R2) were above 0.9, which means that the model represented the data accurately. In addition, the lack of fit values was not significant (p > 0.05), thus further validating the model. Indeed, these results confirm that methanol percentage and extraction temperature were identified as relevant extraction parameters in the single factor study analyses.
Table 2 Analysis of variance and regression coefficients of the predicted model for response variables in apricots
Model parameters |
Regression coefficient |
Cat |
Chl |
Rut |
Abbreviations: Temperature (T); methanol (MeOH); determination coefficient (R2); (+)-catechin (Cat); Chlorogenic acid (Chl); rutin (Rut). Differences between groups determined by ANOVA. *p < 0.05. p-Value of lack of the fit test. |
Intercept |
β0 |
1.29 |
1.51 |
5.49 × 10−1 |
Linear
|
T × T |
β1 |
−3.33 × 10−2* |
1.17 × 10−2 |
2.17 × 10−2* |
MeOH |
β2 |
−1.00 × 10−2 |
−1.65 × 10−1* |
−1.18 × 10−1* |
|
Interaction
|
T × MeOH |
β12 |
2.25 × 10−2 |
−4.25 × 10−2* |
−2.50 × 10−3 |
|
Quadratic
|
T × T |
β11 |
−3.73 × 10−2 |
−3.03 × 10−2 |
−2.87 × 10−2* |
MeOH × MeOH |
β22 |
−1.17 × 10−1* |
−1.00 × 10−1* |
−7.37 × 10−2* |
R
2
|
|
0.9161 |
0.9746 |
0.9884 |
Adjusted R2 |
|
0.8322 |
0.9491 |
0.9767 |
p-Value |
|
0.0101 |
0.0005 |
0.0001 |
F-Value |
|
10.92 |
38.30 |
84.96 |
Lack of fita |
|
0.8957 |
0.2826 |
0.4910 |
3.2.2. Analysis of regression coefficients and response surface plots.
The regression coefficients of the model for catechin, chlorogenic acid and rutin obtained from the multiple linear regressions are reported in Table 2. Dependent variables (i.e., catechin, chlorogenic acid and rutin) allowed direct interpretation of the effect of the independent variables (i.e., methanol proportion and extraction temperature). The visualisation of the statistical significance of the independent variables on the dependent variables was facilitated by the generated surface contour plots (Fig. 2). Precisely, these figures facilitate a more visual effect of the extraction variables on the extraction of the studied phenolic compounds.
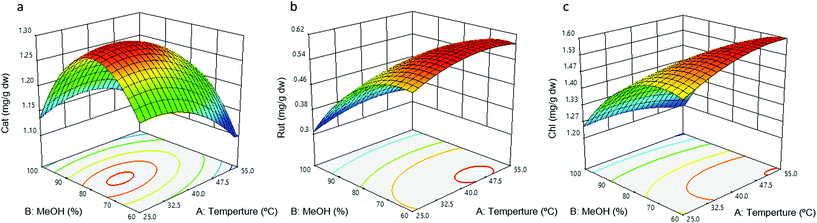 |
| Fig. 2 Response surface plots for catechin (a), rutin (b) and chlorogenic acid (c) as a function of extraction temperature and methanol proportion. Extractions were performed at a liquid-to-solid ratio of 20 mL g−1 under 500 rpm agitation for 30 minutes. Abbreviations: methanol (MeOH), catechin (Cat), rutin (Rut) and chlorogenic acid (Chl). The figure was created with Design-expert 9.0.6 software (Trial version, Stat-Ease Inc., Minneapolis, MN, USA). | |
Regarding the effect of temperature, chlorogenic acid was not influenced by this factor. However, a significant positive linear effect and a negative quadratic effect were observed for rutin, indicating that rutin extraction increases with temperature to a point after which it begins to decrease (Table 2). This behaviour was previously described for flavanols13 and total phenolics4,8 in other plant matrixes. For catechin, a linear negative effect was found to be statistically significant, possibly due to the thermosensitivity of flavanols.13 Indeed, the optimal extraction temperature for catechin according to our optimised results was 32 °C (Fig. 2a), in agreement with the optimal extraction temperature of 30 °C reported by Wani et al. for sun-dried apricots.5 The optimal extraction temperatures reported for chlorogenic acid and rutin were 53 and 46 °C, respectively (Fig. 2b and c), which are very similar to those reported for several phenolics in other plant materials.8,16 Cellular localisation and interaction with cell structural components could explain the difference in the optimal extraction temperatures between the studied compounds.37
When studying methanol percentage, a negative linear effect of chlorogenic acid and rutin and a negative quadratic effect for catechin, chlorogenic acid and rutin were observed and were statistically significant. This implies that an increase in methanol proportion will result in a reduction of the extraction of rutin and chlorogenic acid, as evidenced in Fig. 2. In this sense, Yang et al. also reported a negative linear and quadratic effect of the extraction solvent for total phenolics in Phyllanthus emblica L17 and Liyana-Pathirana et al. in the antioxidant activity of soft wheat bran.14 In addition, in this study, catechin was reported to have an optimal methanol proportion of 78% (Fig. 2a), which is highly similar to the optimal EtOH proportion (84%) reported by Liu et al. for epicatechin in hawthorn fruit.43 Indeed, a methanol proportion of 80% has been shown to be efficient in extracting several phenolics, including epicatechin and procyanidin B3.44 In agreement with the optimal reported methanol proportion of 63% for rutin (Fig. 2b), Wijngaard et al. found an ethanol concentration of 64% to be optimal for the extraction of total flavonols.18 In this sense, solvents of approximately 50 and 60% have been reported to be optimal in the extraction of total phenolics.3,4 The optimal methanol proportion obtained in this study for chlorogenic acid extraction was 60% (Fig. 2c), in agreement with Yilmaz et al., who reported an optimal ethanol concentration for neochlorogenic acid at 56% (ref. 4) and Wijngaard et al. at 58% for chlorogenic acid.18 Notably, aqueous mixtures of organic solvents usually yield better extraction rates.41,45 In fact, the addition of water in extraction solvents promotes fruit particle swelling. This increases the contact area between fruit particles and the extraction solvent, which allows the solvent to penetrate more easily into the food matrix, leading to increased phenolic extraction yields.45
A negative interaction (crossover effect) between temperature and methanol proportion was found to be statistically significant for chlorogenic acid. Silva et al. reported a significant interaction between ethanol concentration and temperature for the total flavonols in Inga edulis leaves.13 Similarly, Pompeu et al. reported this type of interaction for antioxidant activity in Euterpe oleracea fruit,8 and Karacabey et al. reported a significant negative interaction between ethanol concentration and temperature in the antioxidant capacity of grape crane extracts.20
3.2.3. Validation of the model.
The optimised combination of extraction variables at the highest desirability (0.861) was a temperature of 38 °C and methanol at 72%. To validate the veracity of the model, 3 extractions were performed under the optimised conditions. No significant differences were reported between the experimental and predicted extraction rates for catechin, chlorogenic acid and rutin (Table 3). Therefore, the model can accurately predict the behaviour of the response variables within the range of extraction variables studied. Thus, the extraction temperature and methanol proportion were fixed at 38 °C and 72% throughout the rest of the study. Similar to our results, Wani et al. reported a temperature of 30 °C to be optimal in the extraction of total phenolics from sun-dried apricots,5 and Tabaraki et al. reported an optimal ethanol concentration between 60 and 70% for rice brans.16
Table 3 Overall optimal extraction parameters for phenolics in apricots
Extraction variables |
Response variable |
Predicted |
Experimental |
T (°C) |
MeOH (%) |
Desirability |
Abbreviations: Temperature (T); methanol (MeOH); (+)-catechin (Cat); chlorogenic acid (Chl); rutin (Rut). The results are expressed as mg of phenolic components per gram of dry weight (mg per g dw) ± SD (n = 3). |
38 |
72 |
0.861 |
Cat |
1.28 |
1.32 ± 0.03 |
|
|
|
Chl |
1.56 |
1.56 ± 0.14 |
|
|
|
Rut |
0.59 |
0.61 ± 0.01 |
3.3. Effect of time on phenolic extraction
There are many literature examples in which time exerts a significant influence on the extraction of several phenolics.4,9,11,17,25 It has been postulated that time can promote the solubilisation of polyphenols in a solvent due to the cell-wall weakening effects of optimised methanol and temperature conditions.16,17 Therefore, we further evaluate the effect of time on the phenolic extraction of apricots under the optimised conditions of 20 mL g−1, 72% of methanol (1% formic acid), 38 °C within a range of 0 to 120 minutes (500 rpm) (Fig. 3). Variables that encompass multiple representatives of the same phenolic family provide a general view of the effect of a particular extraction parameter. The TPC, TFoC, TEC and TFaC represent the major phenolic families found in apricots26,28,29 and were used to study the effect of extraction time in apricots. However, no significant changes were reported due to time in any of the studied parameters. Therefore, from practical and economic aspects, centrifugation immediately after vortexing was set as the optimal procedure (i.e., 0 min as the extraction time). Consistent with this finding, Thoo et al. found no effect of time on the extraction of flavonoids in Morinda citrifolia.41 In addition, Yang et al. reported a time of 23.16 min and Wani et al. a time of 30 min as optimal in Phyllanthus emblica L. bark and sun-dried apricots, respectively,5,17 which are relatively short extraction times.
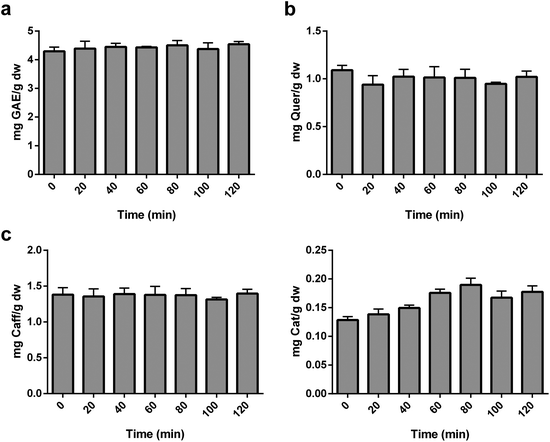 |
| Fig. 3 Effect of time on the extraction of total phenolic (a), total flavonol (b), tartaric ester (c), and total flavanol (d) contents. Extractions were performed at 20 mL g−1, 38 °C, 72% methanol (1% formic acid), and agitation at 500 rpm. The results are expressed as mg of phenolic component per gram of dry weight (mg per g dw) ± SD (n = 3). The figure was created with Graph-Pad PriSM 6.01 software (GraphPad, Sand Diego, CA, USA). | |
3.4. Multi-step extractions
To evaluate whether better extraction could be attained with this optimised method, three consecutive extractions were performed under the optimised conditions of 20 mL g−1, 38 °C, 72% of methanol (1% formic acid). Samples were vortexed with pre-heated extraction solvents and immediately centrifuged. Values above 70% were observed for the TPC, TFoC and TEC in the first extraction step (Fig. 4). Remarkably, few studies evaluate the effect of consecutive extractions in the literature,7,9,17,46 and by applying this approach the extraction of phenolic compounds from food matrixes can increase considerably.17,46 Values between 89% and 94% were reached in the second extraction step. Similarly, Mané et al. reported that flavanols, phenolic acids and anthocyanins in grape skins, seeds and pulp were primarily extracted in the first extraction step.7 Chirinos et al. also reported a yield of 60% in a single step for Tropaeolum tuberosum phenolic extraction.9 However, since our aim was to extract a maximum content of phenolics for accurate quantification, we set two extraction steps as the optimal number of extraction steps in the rest of the experiment. Remarkably, for all the parameters studied, no significant differences were reported between the second and third extraction steps, while the first and second steps were significantly different.
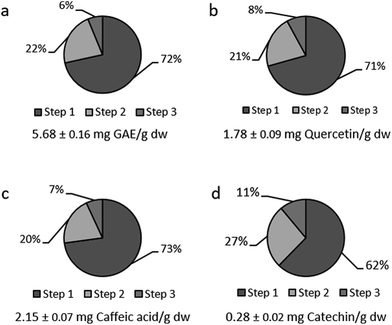 |
| Fig. 4 Effect of multi-step extraction on total phenolic (a), total flavonol (b), tartaric ester (c), and total flavanol (d) contents. Extractions were performed at 20 mL g−1, 38 °C and 72% methanol (1% formic acid). The total quantity of phenolic component extracted after the third step is expressed as mg of phenolic component per gram of dry weight (mg per g dw) ± SD (n = 3). The figure was created with Graph-Pad PriSM 6.01 software (GraphPad, Sand Diego, CA, USA). | |
3.5. Characterisation of apricot phenolics and the effect of the extraction solvent
Considering that apricot characterisation has been mainly realised using unspecific phenolic extraction methodologies,26–29,31,32 we used our specific optimised method to precisely and fully characterize the phenolic profile of Charisma apricots. Moreover, methanolic and ethanolic extractions were performed under the optimised conditions to evaluate the effect of these solvents on the phenolic extraction and characterisation. Our results demonstrate that the methanol and the ethanol-base extractions reported different extraction yields for the TFoC (Table 4) and for individual compounds determined by HPLC-ESI-MS/MS (Table 5). In most individual phenolics, methanol achieved higher extractions than ethanol, which is consistent with the literature,9,25 but, in most cases, the total extracted values in the methanolic and ethanolic extraction were of a similar range. Paradoxically, the TFoC was significantly higher in the ethanol-based extraction. However, in the specific cases of protocatechuic acid and dihydroxybenzoic acid, the methanolic extraction clearly achieved higher extraction rates than the ethanolic one. Ethanol showed some higher extraction rates in individual compounds than methanol. For instance, the ethanol-based extraction achieved significantly higher extractions of chlorogenic acid. Remarkably, chlorogenic acid and related compounds such as caffeic acid have shown important health promoting activities.2 Moreover, there are no differences in the TPC, TEC and TFaC of both extraction solvents. Therefore, considering that ethanol is used in the food industry due to methanol's toxicity,47 our results suggest that the adaptation of this methodology on a larger scale could be used to produce phenolic rich extracts with potential bioactive functions.
Table 4 Quantification of apricot (Prunus armeniaca) phenolics by HPLC-DAD using methanol or ethanol as the extraction solvent
|
MeOH |
EtOH |
p-Value |
Abbreviations: Total phenolic content (TPC); total flavonol content (TFoC); tartaric ester content (TEC); and total flavanol content (TFaC). Extractions were performed twice under optimised conditions: organic solvent 72% and formic acid 1%, at 20 mL g−1 and 38 °C. The results are expressed as mg of phenolic component per gram of dry weight (mg per g dw) ± SD (n = 3). Statistics by Student's t-test. |
TPC |
5.33 ± 0.16 |
5.24 ± 0.14 |
0.232 |
TFoC |
1.21 ± 0.10 |
1.47 ± 0.09 |
0.002 |
TEC |
1.51 ± 0.10 |
1.55 ± 0.07 |
0.451 |
TFaC |
0.252 ± 0.02 |
0.257 ± 0.01 |
0.556 |
Table 5 Quantification of apricot (Prunus armeniaca) phenolics by HPLC-ESI-MS/MS
Compound |
MeOH |
EtOH |
p-Value |
Extractions were performed twice under optimised conditions: organic solvent 72% and formic acid 1%, at 20 mL g−1 and 38 °C. The results are expressed in mg per kg dw ± SD (n = 3) (statistics by Student's t-test). d1, d2, d3, d4 and d5 indicate different isomeric compounds. Quantified using the calibration curve of benzoic acid. Quantified using the calibration curve of protocatechuic acid. Quantified using the calibration curve of gallic acid. Quantified using the calibration curve of caffeic acid. Quantified using the calibration curve of chlorogenic acid. Quantified using the calibration curve of ferulic acid. Quantified using the calibration curve of resveratrol. Quantified using the calibration curve of catechin. Quantified using the calibration curve of procyanidin dimer B2. Abbreviations: n.d., not detected; n.q., not quantified. |
Benzoic acid |
5.88 ± 0.30 |
6.21 ± 0.16 |
0.16 |
Hydroxybenzoic acida |
2.34 ± 0.05 |
2.45 ± 0.10 |
0.17 |
Protocatechuic acid |
185.11 ± 5.10 |
6.63 ± 0.21 |
<0.01 |
Dihydroxybenzoic acidb |
56.52 ± 1.30 |
n.q. |
|
p-Coumaric acid |
n.q. |
n.q. |
|
Gallic acid |
n.q. |
n.q. |
|
Ferulic acid |
n.q. |
n.q. |
|
Resveratrol |
0.16 ± 0.00 |
0.15 ± 0.00 |
<0.01 |
Kaempferol |
0.05 ± 0.03 |
0.03 ± 0.00 |
0.31 |
Catechin |
20.00 ± 0.93 |
18.45 ± 0.83 |
0.10 |
Epicatechin |
16.44 ± 0.90 |
15.31 ± 0.34 |
0.11 |
Quercetin |
n.q. |
n.q. |
|
Protocatechuic acid glucosideb |
1.54 ± 0.05 |
1.59 ± 0.03 |
0.19 |
Gallic acid O-glucoside d1c |
0.01 ± 0.00 |
0.02 ± 0.00 |
0.03 |
Gallic acid O-glucoside d2c |
0.18 ± 0.01 |
0.20 ± 0.01 |
0.01 |
Caffeic acid O-glucoside d1d |
2.79 ± 0.04 |
3.25 ± 0.26 |
0.04 |
Caffeic acid O-glucoside d2d |
4.77 ± 0.21 |
5.16 ± 0.25 |
0.11 |
Caffeic acid O-glucoside d3d |
20.43 ± 0.60 |
21.69 ± 0.68 |
0.07 |
Neochlorogenic acide |
565.79 ± 16.82 |
586.19 ± 13.60 |
0.18 |
Chlorogenic acid |
178.60 ± 13.11 |
216.36 ± 4.81 |
0.01 |
Cryptogenic acide |
41.55 ± 3.50 |
36.60 ± 2.61 |
0.12 |
Feruloylquinic acidf |
3.32 ± 0.04 |
3.45 ± 0.05 |
0.02 |
Resveratrol O-glucoside d1g |
0.16 ± 0.00 |
0.15 ± 0.00 |
0.01 |
Resveratrol O-glucoside d2g |
0.13 ± 0.01 |
0.15 ± 0.01 |
0.06 |
Catechin gallateh |
0.70 ± 0.01 |
0.71 ± 0.02 |
0.24 |
Kaempferol-3-O-glucoside |
0.47 ± 0.04 |
0.46 ± 0.01 |
0.75 |
Eriodictyol-7-O-glucoside |
0.32 ± 0.01 |
0.32 ± 0.00 |
0.32 |
Catechin O-glucosideh |
0.51 ± 0.04 |
0.46 ± 0.01 |
0.10 |
Hyperoside |
13.20 ± 0.84 |
12.87 ± 0.40 |
0.57 |
Isorhamnetin-3-O-glucoside |
0.03 ± 0.00 |
0.03 ± 0.00 |
0.72 |
Procyanidin dimer d1i |
14.39 ± 0.73 |
11.44 ± 0.24 |
<0.01 |
Procyanidin dimer d2i |
6.63 ± 0.22 |
5.99 ± 0.34 |
0.05 |
Procyanidin dimer B2 |
6.69 ± 0.21 |
5.80 ± 0.26 |
0.01 |
Procyanidin dimer d3i |
1.00 ± 0.08 |
0.85 ± 0.04 |
0.04 |
Procyanidin dimer d4i |
2.80 ± 0.20 |
2.50 ± 0.06 |
0.07 |
Procyanidin dimer d5i |
0.68 ± 0.01 |
0.62 ± 0.01 |
<0.01 |
Kaempferol-3-O-rutinoside |
14.51 ± 1.05 |
14.27 ± 0.21 |
0.72 |
Rutin |
278.94 ± 9.71 |
238.33 ± 7.81 |
<0.01 |
Procyanidin trimeri |
0.38 ± 0.01 |
0.30 ± 0.03 |
0.01 |
Methanol, which generally achieves better extraction rates, was demonstrated to be the optimal solvent to accurately profile the phenolic content of apricots. It is worth considering that, since apricot phenolics vary due to their variety, maturity stage and region of cultivation,26–29 comparing the extraction rate of different methods is controversial. Our optimised method achieved 5.33 ± 0.16 mg GAE per g dw, within the range of the TPC (8.18–4.23 mg GAE per g dw) reported for several apricot varieties.24 However, our method extracted higher TPC than apricots from different varieties in different studies.30 In agreement with the phenolic profile of apricots, we found rutin and hydroxycinnamic acids (Table 5) to be the most abundant compounds in apricots.25,27,30 In the case of the most representative phenolic compounds in apricots, higher quantities were extracted with this optimized method than the values reported in the literature. For example, rutin, which was reported to range between 15.50 and 69.52 mg per kg dw in several apricot varieties, was found at 278.94 ± 9.71 mg per kg dw in our study.27 Similar concentrations of chlorogenic acid were found in the study of Karabulut et al. compared to the one we found.30 However, our method extracted 178.60 ± 13.11 mg chlorogenic acid per kg dw, which is greater than the concentrations found in Ordubat (42.41 ± 0.01 mg per kg dw), Stark early orange (93.86 ± 0.01 mg per kg dw) and Wilson delicious (147.31 ± 0.00 mg per kg dw), among other varieties.26 Similarly, lower concentrations of chlorogenic acid were found in the study of Kan et al.27 Karabulut et al. reported neochlorogenic acid at concentrations between 103.72 and 285.29 mg per kg dw,30 and, in this study, the observed neochlorogenic acid's concentration was 565.79 ± 16.82 mg per kg dw.
All in all, better extraction rates than reported in the literature using unspecific methods for apricots were achieved for most of the representative phenolic compounds. In addition to the good extraction rates achieved, this method is faster than the one developed for sun-dried apricots5 and other plant matrixes.4,13 Moreover, the required temperatures4,11,13,15 and LSR11,15,19 are lower than those of other methods for different plant matrixes, making our method more economically feasible.
4. Conclusions
In this study, we developed a fast and economically feasible method for the specific extraction of apricot phenolics. The optimised conditions were as follows: 20 g mL−1, 38 °C and 72% methanol (1% formic acid). The phenolic extraction using this method allowed accurate characterisation of the phenolic profile of apricots, which is essential in the study of their bioactivity. Additionally, the ethanol-based extraction has potential to be adapted to the food industry for the production of phenolic-rich extracts with potential bioactive effects.
Abbreviations
Dw | Dry weight |
GAE | Gallic acid equivalents |
LSR | Liquid-to-solid ratio |
RSM | Response surface methodology |
TEC | Tartaric ester content |
TFaC | Total flavanol content |
TFoC | Total flavonol content |
TPC | Total phenolic content |
Conflicts of interest
There are no conflicts to declare.
Acknowledgements
This work was supported by the Spanish Ministry of Economy and Competitiveness (grant numbers AGL2013-49500-EXP and AGL2016-77105-R) and by European Regional Development Funds of the European Union within the Operative Program FEDER of Catalunya 2014-2020 (PECT-NUTRISALT). L. I.-C. is a recipient of a predoctoral fellowship from Universitat Rovira i Virgili – Martí i Franquès. Grant number: 2015PMF-PIPF-50. A. M.-C. is a recipient of a predoctoral fellowship from Universitat Rovira i Virgili – Martí i Franquès. Grant number: 2015PMF-PIPF-51. A. A.-A. is a Serra Húnter fellow. We express deep thanks to Dr Pol Herrero, Dr Antoni Del Pino, Niurka Llópiz and Rosa M. Pastor for their technical help and advice.
References
- L. Hooper, P. A. Kroon, E. B. Rimm, J. S. Cohn, I. Harvey, K. A. Le Cornu, J. J. Ryder, W. L. Hall and A. Cassidy, Am. J. Clin. Nutr., 2008, 88, 38–50 CrossRef CAS PubMed.
- M. N. Clifford, I. B. Jaganath, I. A. Ludwig and A. Crozier, Nat. Prod. Rep., 2017, 34, 1391–1421 RSC.
- J. E. Cacace and G. Mazza, J. Food Sci., 2003, 68, 240–248 CrossRef CAS.
- F. M. Yılmaz, M. Karaaslan and H. Vardin, J. Food Sci. Technol., 2015, 52, 2851–2859 CrossRef PubMed.
- S. M. Wani, N. Jan, T. A. Wani, M. Ahmad, F. A. Masoodi and A. Gani, J. Saudi Soc. Agric. Sci., 2017, 16, 119–126 Search PubMed.
- M. L. Bengoechea, A. I. Sancho, B. Bartolomé, I. Estrella, C. Gómez-Cordovés and M. T. Hernández, J. Agric. Food Chem., 1997, 45, 4071–4075 CrossRef CAS.
- C. Mané, J. M. Souquet, D. Ollé, C. Verriés, F. Véran, G. Mazerolles, V. Cheynier and H. Fulcrand, J. Agric. Food Chem., 2007, 55, 7224–7233 CrossRef PubMed.
- D. R. Pompeu, E. M. Silva and H. Rogez, Bioresour. Technol., 2009, 100, 6076–6082 CrossRef CAS PubMed.
- R. Chirinos, H. Rogez, D. Campos, R. Pedreschi and Y. Larondelle, Sep. Purif. Technol., 2007, 55, 217–225 CrossRef CAS.
- O. Zitka, J. Sochor, O. Rop, S. Skalickova, P. Sobrova, J. Zehnalek, M. Beklova, B. Krska, V. Adam and R. Kizek, Molecules, 2011, 16, 2914–2936 CrossRef CAS PubMed.
- L. Iglesias-Carres, A. Mas-Capdevila, L. Sancho-Pardo, F. I. Bravo, M. Mulero, B. Muguerza and A. Arola-Arnal, Nutrients, 2018, 10, 1931 CrossRef PubMed.
- L. Iglesias-Carres, A. Mas-Capdevila, F. I. Bravo, G. Aragonès, B. Muguerza and A. Arola-Arnal, PLoS One, 2019, 14, e0211267 CrossRef CAS PubMed.
- E. M. Silva, H. Rogez and Y. Larondelle, Sep. Purif. Technol., 2007, 55, 381–387 CrossRef CAS.
- C. Liyana-Pathirana and F. Shahidi, Food Chem., 2005, 93, 47–56 CrossRef CAS.
- K. N. Prasad, F. A. Hassan, B. Yang, K. W. Kong, R. N. Ramanan, A. Azlan and A. Ismail, Food Chem., 2011, 128, 1121–1127 CrossRef CAS.
- R. Tabaraki and A. Nateghi, Ultrason. Sonochem., 2011, 18, 1279–1286 CrossRef CAS PubMed.
- L. Yang, J. G. Jiang, W. F. Li, J. Chen, D. Y. Wang and L. Zhu, J. Sep. Sci., 2009, 32, 1437–1444 CrossRef CAS PubMed.
- H. H. Wijngaard and N. Brunton, J. Food Eng., 2010, 96, 134–140 CrossRef CAS.
- Y. Liu, S. Wei and M. Liao, Ind. Crops Prod., 2013, 49, 837–843 CrossRef CAS.
- E. Karacabey and G. Mazza, Food Chem., 2010, 119, 343–348 CrossRef CAS.
- C. Y. Gan and A. A. Latiff, Food Chem., 2011, 124, 1277–1283 CrossRef CAS.
- J. Pérez-Jiménez, V. Neveu, F. Vos and A. Scalbert, Eur. J. Clin. Nutr., 2010, 64(Suppl 3), S112–S120 CrossRef PubMed.
- F. Fratianni, M. N. Ombra, A. D'Acierno, L. Cipriano and F. Nazzaro, Curr. Opin. Food Sci., 2018, 19, 23–29 CrossRef.
- E. B. Akin, I. Karabulut and A. Topcu, Food Chem., 2008, 107, 939–948 CrossRef CAS.
- S. Erdoan and S. Erdemoğlu, Int. J. Food Sci. Nutr., 2011, 62, 729–739 CrossRef PubMed.
- M. Gundogdu, S. Ercisli, S. Berk, T. Kan, I. Canan and M. K. Gecer, J. Food Meas. Charact., 2017, 11, 2087–2093 CrossRef.
- T. Kan, M. Gundogdu, S. Ercisli, F. Muradoglu, F. Celik, M. K. Gecer, O. Kodad and M. Zia-Ul-Haq, Biol. Res., 2014, 47, 1–6 Search PubMed.
- V. Dragovic-Uzelac, B. Levaj, V. Mrkic, D. Bursac and M. Boras, Food Chem., 2007, 102, 966–975 CrossRef CAS.
- T. Kan and S. Z. Bostan, J. Food Process. Preserv., 2013, 37, 163–170 CrossRef CAS.
- I. Karabulut, T. Bilenler, K. Sislioglu, I. Gokbulut, F. Seyhan, I. S. Ozdemir and B. Ozturk, J. Food Biochem., 2018, 42, e12458–e12470 CrossRef.
- V. Dragovic-Uzelac, K. Delonga, B. Levaj, S. Djakovic and J. Pospisil, J. Agric. Food Chem., 2005, 53, 4836–4842 CrossRef CAS PubMed.
- S. M. Wani, P. R. Hussain, F. A. Masoodi, M. Ahmad, T. A. Wani, A. Gani, S. A. Rather and P. Suradkar, J. Agric. Sci., 2017, 9, 66–82 Search PubMed.
- V. M. Burin, N. E. Ferreira-Lima, C. P. Panceri and M. T. Bordignon-Luiz, Microchem. J., 2014, 114, 155–163 CrossRef CAS.
-
Di. Cheaib, N. El Darra, H. N. Rajha, R. G. Maroun and N. Louka, Sci. World J Search PubMed.
- A. Arnous, D. P. Makris and P. Kefalas, J. Food Compos. Anal., 2002, 15, 655–665 CrossRef CAS.
- Y. Sun, W. Xu, W. Zhang, Q. Hu and X. Zeng, Sep. Purif. Technol., 2011, 78, 311–320 CrossRef CAS.
- M. Pinelo, A. Arnous and A. S. Meyer, Trends Food Sci. Technol., 2006, 17, 579–590 CrossRef CAS.
- J. E. Cacace and G. Mazza, J. Food Eng., 2003, 59, 379–389 CrossRef.
- A. A. Van Der Sluis, M. Dekker and M. A. J. S. Van Boekel, J. Agric. Food Chem., 2005, 53, 1073–1080 CrossRef CAS PubMed.
- G. D. S. C. Borges, F. G. K. Vieira, C. Copetti, L. V. Gonzaga and R. Fett, Food Res. Int., 2011, 44, 708–715 CrossRef CAS.
- Y. Y. Thoo, S. K. Ho, J. Y. Liang, C. W. Ho and C. P. Tan, Food Chem., 2010, 120, 290–295 CrossRef CAS.
- D. Baş and İ. H. Boyaci, J. Food Eng., 2007, 78, 846–854 CrossRef.
- J. L. Liu, J. F. Yuan and Z. Q. Zhang, Int. J. Food Sci. Technol., 2010, 45, 2400–2406 CrossRef CAS.
- J. Oszmianski and C. Y. Lee, Am. J. Enol. Vitic., 1990, 41, 204–206 CAS.
- W. Li, Z. Wang, Y. P. Wang, C. Jiang, Q. Liu, Y. S. Sun and Y. N. Zheng, Food Chem., 2012, 130, 1044–1049 CrossRef CAS.
- N. E. Durling, O. J. Catchpole, J. B. Grey, R. F. Webby, K. A. Mitchell, L. Y. Foo and N. B. Perry, Food Chem., 2007, 101, 1417–1424 CrossRef CAS.
- R. Kushwaha and S. Karanjekar, Int. J. ChemTech Res., 2011, 3, 1033–1036 Search PubMed.
Footnotes |
† Electronic supplementary information (ESI) available. See DOI: 10.1039/c9fo00353c |
‡ Deceased. |
|
This journal is © The Royal Society of Chemistry 2019 |
Click here to see how this site uses Cookies. View our privacy policy here.