DOI:
10.1039/C9FO00115H
(Paper)
Food Funct., 2019,
10, 3303-3311
GPETAFLR, an octapeptide isolated from Lupinus angustifolius L. protein hydrolysate, promotes the skewing to the M2 phenotype in human primary monocytes
Received
19th January 2019
, Accepted 16th April 2019
First published on 23rd April 2019
Abstract
The present study aimed to test the mechanisms by which GPETAFLR, released from the enzymatic hydrolysis of lupine protein, may modulate the inflammatory response and plasticity in human primary monocytes. Human circulating monocytes and mature macrophages were used to analyze the effects of GPETAFLR on plasticity and inflammatory response using biochemical, flow cytometry, quantitative real-time PCR, and ELISA assays. GPETAFLR skewed the monocyte plasticity towards the anti-inflammatory non-classical CD14+CD16++ monocyte subset and reduced the inflammatory competence of LPS-treated human monocytes diminishing IL-1β, IL-6, and TNF-α and increasing IL-10 production and gene expression. Results showed that GPETAFLR decreased the frequency of the LPS-induced activated monocyte population (CD14++CD16−), diminished monocyte activation involved down-regulation of CCR2 mRNA expression and protein expression, and decreased gene expression of the LPS-induced chemoattractant mediator CCL2. Our findings imply a new understanding of the mechanisms by which GPETAFLR favor a continuous and gradual plasticity process in the human monocyte/macrophage system and offer novel benefits derived from the consumption of Lupinus angustifolius L. in the prevention of inflammatory-related diseases.
Introduction
The word nutraceutical is the association of the terms nutrition and pharmaceutical and is considered as a food or food product that can offer medical and health benefits for the prevention and treatment of diseases. Most nutraceutical peptides are isolated from protein precursors by digestive enzymes, during food processing, storage, or by the in vitro hydrolysis of several proteolytic enzymes.1 Although milk proteins are the main source of bioactive peptides studied so far, there are many other peptides of animal and plant origin. In fact, more than 3000 peptides with 44 different biological activities have been identified and recently collected in the “Biopep” database.2 In this sense, the study of plant proteins has emerged as an alternative to produce bioactive peptides,3 small sequences of amino acids that are inactive within the intact protein but can be released by gastrointestinal enzymatic hydrolysis reaching the bloodstream as active molecules.4
The immune system is responsible for responding to any type of aggression in the body. It has its origin in the lymphoid organs (lymph nodes, spleen, thymus, and bone marrow) and in its action many cellular subtypes (immunocompetent cells), including monocytes and neutrophils, participate. Monocytes express several receptors that control and detect environmental changes, are highly plastic and heterogeneous, and change their functional phenotype in response to environmental stimulation.5,6 Inflammation is one of the complex biological responses of an organism's immune system. Evidence from murine and human studies has suggested that monocytosis may be an indicator of several inflammatory diseases.7 Therefore, the use of primary monocytes as a model of inflammation is widespread and is used in numerous studies to test the anti-inflammatory activity of various compounds.8,9 The secretion of pro-inflammatory mediators works as an inflammatory beacon for leukocytes, which contribute to all stages of several inflammatory disorders, therefore representing an important therapeutic target.10
Human monocytes are classified into three subsets: CD14++CD16– (classical monocytes), intermediate CD14++CD16+ (intermediate monocytes), and CD14+CD16++ (non-classical monocytes).11 So far, classical monocytes represent the major fraction (about 85% of total monocytes) and highly express CCR2; they are professional phagocytes giving rise to M1 macrophages, which secrete cytokines (TNF-α, IL-1β, and IL-6) in response to LPS during infection or inflammation.12 Intermediate monocytes display the highest levels of CCR5, TLR4, CD163, and HLA-DR during activation and also secrete pro-inflammatory cytokines such as IL-10.13 Non-classical monocytes are less granular and smaller in size, with lower expression of CCR2 than classical or intermediate subsets.14,15 These monocytes rich in CD16 are functionally involved in tissue repairing, patrolling, and wound healing, and have the tendency to be polarized into M2 macrophages with an anti-inflammatory phenotype in response to a variety of stimuli, including IL-4.16
We have previously shown the presence of a new peptide isolated from a protein hydrolysate of Lupinus angustifolius L.17 The aim of the present study was to investigate the effects of the GPETAFLR peptide on human primary monocytes and human mature macrophage activation as hallmarks of inflammatory disorders.
Materials and methods
Synthesis of the peptide
Seeds of lupine (Lupinus angustifolius L.) were a gift from Koipesol Semillas, S.A. (Sevilla, Spain). Alcalase 2.4 L was provided by Novozymes (Bagsvaerd, Denmark). Lupine protein isolate (LPI) was obtained through the method of Yust, Pedroche, Millán-Linares, Alcaide-Hidalgo, and Millán (2010)18 at the pilot plant scale (Plant Proteins Group, Instituto de la Grasa, Seville, Spain). The chemical composition of LPI in dry matter was: proteins 86.83%; dietary fibre 5.97%; oil 5.08%; ash 0.78%; and carbohydrates 1.34%. Hydrolysis was carried out according to Millan-Linares, Yust, Alcaide-Hidalgo, Millan, and Pedroche (2014).19 Lupine protein isolate (LPI) was suspended in distilled water (10% w/v), and hydrolysis with alcalase was performed under the following conditions: pH 8, 50 °C, E/S = 0.3 AU g−1 protein, and a hydrolysis time of 15 min. The mixture was heated at 85 °C for 15 min to inactivate the enzyme, centrifuged at 6500g for 15 min, and the supernatant constituted the lupine protein hydrolysate (LPH). LPH was purified by ultrafiltration and chromatographic techniques according to Millán-Linares et al. (2015).17 The sequence of the purified peptide was identified through sequence analysis by nanoHPLC coupled to a Polaris Q ion trap mass spectrometer (Mass Spectrometry Unit, Instituto de la Grasa). Finally, the peptide was synthesized by the Fmoc solid phase method by the Barcelona Scientific Park Foundation (Barcelona, Spain) at 95% purity, measured using HPLC-UV at 220 nm.
Blood collection and isolation of human monocytes
This study was conducted according to Good Clinical Practice Guidelines and is in line with the principles outlined in the Helsinki Declaration of the World Medical Association. Informed consent for the study was obtained from healthy male blood donors (age <35 years) at the University Hospital Virgen del Rocio (UHVR, Seville). Ethics approval was obtained from the Human Clinical Research and Ethics Committee of the UHVR (AGL2012-40247-C02-01). Participants declared that they were non-smokers and were not taking any medication. Peripheral blood samples were drawn from a large antecubital vein and collected into K3EDTA-containing tubes (Becton Dickinson, NJ, USA). Peripheral blood mononuclear cells (PBMCs) were isolated from peripheral blood samples by centrifugation over a Ficoll-Histopaque (Sigma-Aldrich, Madrid, Spain) gradient. Monocytes were isolated from PBMCs using CD14 microbeads and LS columns on a midiMACS system (Miltenyi Biotec, Madrid, Spain) according to the manufacturer's instructions. The purity for CD14 monocyte isolations was routinely >95% by flow cytometry (FACScanto II flow cytometer and FACSDiva software, BD). Following isolation, monocytes were suspended in an RPMI 1640 medium supplemented with L-glutamine, penicillin, streptomycin and 10% heat-inactivated foetal bovine serum. For treatments, 5 × 105 of purified monocytes, after in vitro stimulation with or without LPS (100 ng mL−1), were exposed to GPETAFLR at 50–100 μg mL−1 (P2 and P1, respectively) for 24 h.
Monocyte differentiation and polarization into M1 and M2 macrophages
Monocytes were induced to differentiate for 6 days in the presence of recombinant human M-CSF (25 ng mL−1) to obtain M0 macrophages. These cells were then cultured in RPMI 1640 supplemented with L-glutamine, penicillin, streptomycin, and 10% heat-inactivated FBS. For M1 and M2 polarization, M0 macrophages were exposed to LPS (100 ng mL−1) plus IFNγ (20 ng mL−1) and to IL-4 (20 ng mL−1), respectively, for additional 24 h. To evaluate the effect of the GPETAFLR peptide on macrophage polarization, M0 macrophages were exposed to GPETAFLR at 50–100 μg mL−1 for 24 h.
Cell viability assay (MTT)
Monocytes were incubated with different concentrations of GPETAFLR peptide in a 96-well plates (1 × 104 cells per well) during 24 h. Afterward, the MTT solution (Sigma) was incubated in the well until a purple precipitate was visible. MTT-formazan crystals were solubilized with DMSO (Sigma), and then measured with a microplate reader at 570 nm corrected to 650 nm.20 Cell survival was expressed as the percentage of absorbance compared with that obtained in control, non-treated cells.
Immunostaining of circulating monocytes by FACS
Circulating monocyte membrane expression of CD16 (PE anti-human CD16, Miltenyi), CD14 (APC-Cy7 anti-human CD14, Miltenyi), and CCR2 (APC anti-human CCR2, Vitro) on monocytes was analysed using flow cytometry. According to the manufacturer's instructions, 5 × 105 of purified monocytes, after in vitro stimulation with or without LPS (100 ng mL−1), were exposed to GPETAFLR at 50–100 μg mL−1 for 24 h. Thereafter, cells were incubated with antibodies at room temperature and in the dark for 15 min; erythrocytes were removed with FACS lysing solution (Becton Dickinson). Mean fluorescence intensity (MFI) was measured by using a FACSCanto II flow cytometer (Becton Dickinson) and calibrated using a FACSDiva software (Becton Dickinson). MFI of 104 cells was assessed for each sample. Monocytes were gated as forward scatterhigh (FSChigh)-side scatterhigh (SSChigh) cells. Expression levels are presented as MFI corrected for the nonspecific binding of isotope control antibodies.
RNA isolation and qRT-PCR analysis
Total RNA was extracted using the Trisure Reagent (Bioline), as instructed by the manufacturer. The A260/A280 ratio in a NanoDrop ND-1000 spectrophotometer (Thermo Scientific, Madrid, Spain) was used to determinate RNA quality. Momentarily, RNA (1 μg) was subjected to reverse transcription (iScript, Bio-Rad, Madrid, Spain). An amount of 10 ng of the resulting cDNA was used as a template for real-time PCR amplifications. The mRNA levels for specific genes were determined in a CFX96 system (Bio-Rad). For each PCR reaction, the cDNA template was added to Brilliant SYBR Green QPCR Supermix (Bio-Rad) containing the primer pairs for either gene or for glyceraldehyde 3-phosphate dehydrogenase (GAPDH) and hypoxanthine phosphoribosyltransferase 1 (HPRT) as housekeeping genes (Table 1). All amplification reactions were performed in triplicate and average threshold cycle (Ct) numbers of the triplicates were used to calculate the relative mRNA expression of candidate genes. The magnitude of change of mRNA expression for candidate genes was calculated using the standard 2−(ΔΔCt) method. All data were normalized to endogenous reference (GAPDH and HPRT) gene content and expressed as percentage of controls.
Table 1 Sequences of RT-PCR primers for gene expression analysis
Target |
GenBank accession number |
Direction |
Sequence (5′ → 3′) |
TNF-alpha
|
NM_000594 |
Forward |
TCCTTCAGACACCCTCAACC |
Reverse |
AGGCCCCAGTTTGAATTCTT |
IL1beta
|
NM_000576 |
Forward |
GGGCCTCAAGGAAAAGAATC |
Reverse |
TTCTGCTTGAGAGGTGCTGA |
IL6
|
NM_000600 |
Forward |
TACCCCCAGGAGAAGATTCC |
Reverse |
TTTTCTGCCAGTGCCTCTTT |
GAPDH
|
NM_001289746 |
Forward |
CACATGGCCTCCAAGGAGTAAG |
Reverse |
CCAGCAGTGAGGGTCTCTCT |
HPRT 1
|
NM_000194 |
Forward |
ACCCCACGAAGTGTTGGATA |
Reverse |
AAGCAGATGGCCACAGAACT |
CD80
|
NM_005191.3 |
Forward |
GGGAAAGTGTACGCCCTGTA |
Reverse |
GCTACTTCTGTGCCCACCAT |
CD200r
|
NM_138940.2 |
Forward |
GTTGCCCTCCTATCGCATTA |
Reverse |
TGGAAATTCCCATCAGGTGT |
CD64
|
NM_000566.3 |
Forward |
GTCCAAATCTCCAAGTGCGG |
Reverse |
CCCAAGTATGAGAGCAGCGT |
MRC-1
|
NM_138806 |
Forward |
GGCGGTGACCTCACAAGTAT |
Reverse |
ACGAAGCCATTTGGTAAACG |
Cytokine quantification
The levels of TNF-α, IL-1β, IL-6, and IL-10 in culture supernatants were determined by the enzyme-linked immunosorbent assay (ELISA), following the indications of the manufacturer (Diaclone, Besancon, France). The cytokine concentrations were expressed in pg per mL, as calculated from the calibration curves from serial dilution of human recombinant standards in each assay.
Statistical analysis
All values are expressed as arithmetic means ± standard deviations (SD). Data were evaluated with GraphPad Prism Version 5.01 software (San Diego, CA, USA). The statistical significance of any difference in each parameter among the groups was evaluated by one-way analysis of variance (ANOVA), following the Tukey multiple comparisons test as post hoc test. P values less than 0.05 were considered statistically significant.
Results
Effect of GPETAFLR on classical, non-classical, and intermediate monocyte subsets
FACS analysis of the monocyte surface markers CD14 and CD16 showed different results with the different treatments on monocyte subsets (Fig. 1A). Classical monocytes (CD14++CD16−) were increased in LPS-treated monocytes; however, the treatment with GPETAFLR decreased this population (Fig. 1B). Intermediate monocytes did not show significant differences when it was exposed with GPETAFLR (Fig. 1C). In contrast, non-classical monocytes (CD14+CD16++) were decreased with LPS. However, the treatment with GPETAFLR at 100 μg mL−1 increased the non-classical monocyte subset (Fig. 1D).
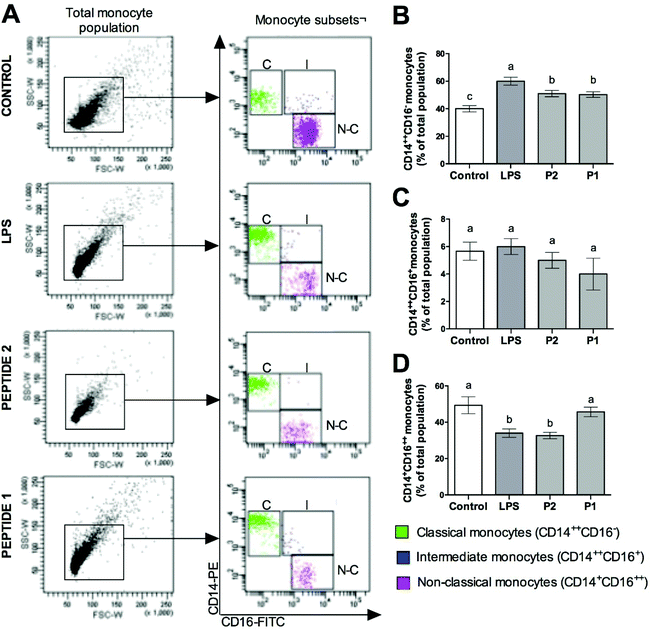 |
| Fig. 1 Effect of GPETAFLR on monocyte subsets. (A) FACS analysis (MFI) of monocyte surface markers CD14 and CD16 after 24 h incubation with or without LPS (100 ng mL−1) and peptide at 50–100 μg mL−1 (Peptide 2 and Peptide 1, respectively). (B) Classical CD14++CD16− monocytes, (C) intermediate CD14++CD16+ monocytes, and (D) non-classical CD14+CD16++ monocytes. Values are presented as means ± SD (n = 3) and those marked with different letters are significantly different (P < 0.05). | |
Effect of GPETAFLR on CCR2/CCL2 axis in monocyte subsets
The CCR2/CCL2 axis was studied (Fig. 2). CCR2 protein expression was analysed in monocyte subsets by FACS analysis (Fig. 2A). GPETAFLR decreased significantly CCR2 expression primarily on classical monocyte subsets compared to LPS (Fig. 2B), although no significantly differences were found on CCR2 MFI in non-classical or intermediate monocytes, there was a reduction tendency. In addition, mRNA expression of CCL2 and its receptor, CCR2 was analysed. LPS-treated monocytes showed a CCR2 up-regulation (Fig. 2C). However, those that were treated with GPETAFLR peptide had a lower increase in CCR2 mRNA levels than those that were treated with LPS. Regarding CCL2 mRNA levels, LPS treatment up-regulated its expression, whereas that GPETAFLR treatment down-regulated CCL2 expression compared to these treated with LPS (Fig. 2D).
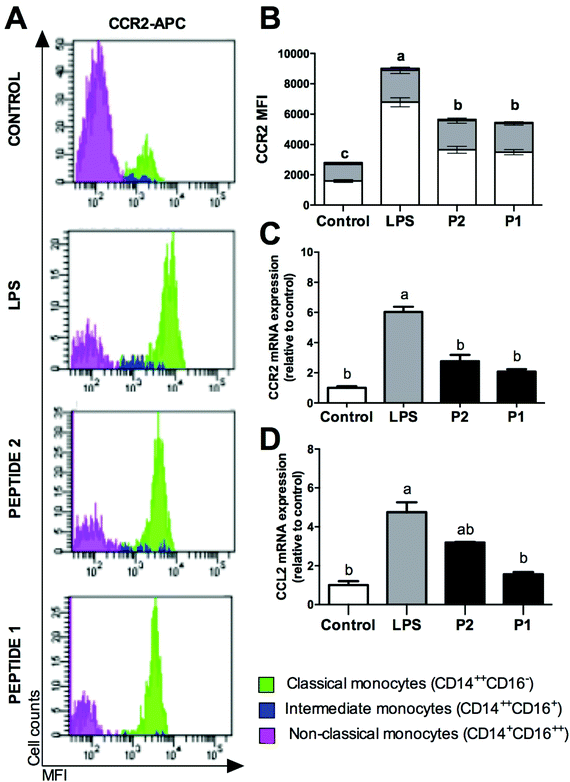 |
| Fig. 2 Effect of GPETAFLR on the CCR2/CCL2 axis. (A) Representative overlay of MFI as a marker for CCR2 in monocyte subsets after 24 h incubation with or without LPS (100 ng mL−1) and peptide at 50–100 μg mL−1 (Peptide 2 and Peptide 1, respectively). (B) FACS analysis of monocyte CCR2 expression in subsets by means of CD14 and CD16 surface marker expression. (C, D) Relative expression of CCR2 and CCL2 genes were measured using RT-qPCR. Values are presented as means ± SD (n = 3) and those marked with different letters are significantly different (P < 0.05). | |
Effect of GPETAFLR on cytokine expression and release in primary human monocytes
In addition to monocyte subsets, we observed that LPS-induced the release of pro-inflammatory cytokines (TNF-α, IL-1β, and IL-6) and expression of genes encoding these cytokines were diminished by GPETAFLR in LPS-treated human monocytes (Fig. 3A–F). In all these cases, GPETAFLR effects were dose-dependent. Secretion and gene expression of the anti-inflammatory IL-10 was also promoted by GPETAFLR (Fig. 3G and H).
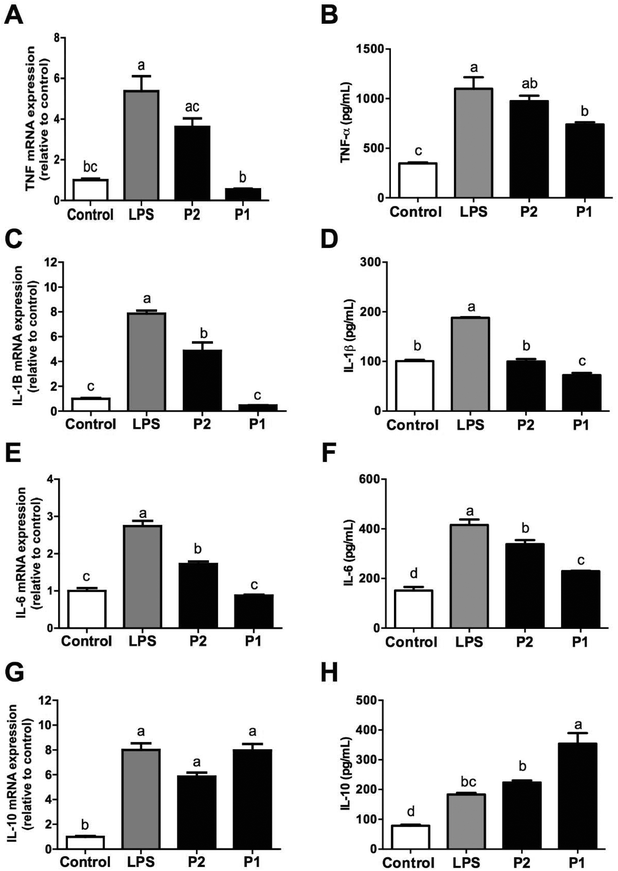 |
| Fig. 3 Effect of GPETAFLR on pro-inflammatory cytokine release in primary human monocytes. (A, C, E, G) Gene expression and (B, D, F, H) and secretion of TNFα, IL-1β, IL-6, and IL-10 in primary human monocytes after 24 h incubation with or without LPS (100 ng mL−1) and peptide at 50–100 μg mL−1 (Peptide 2 and Peptide 1, respectively). Values are presented as means ± SD (n = 3) and those marked with different letters are significantly different (P < 0.05). | |
Effect of GPETAFLR on modulation of M1/M2 macrophage polarisation
Macrophage polarization depends on different microenvironment and signals that promote specific phenotypes. These phenotypes correspond to classically (M1) and alternatively (M2) polarized macrophages. Relative expressions of CD80, CD64, CD200R and MRC-1 were measured using RT-qPCR (Fig. 4).
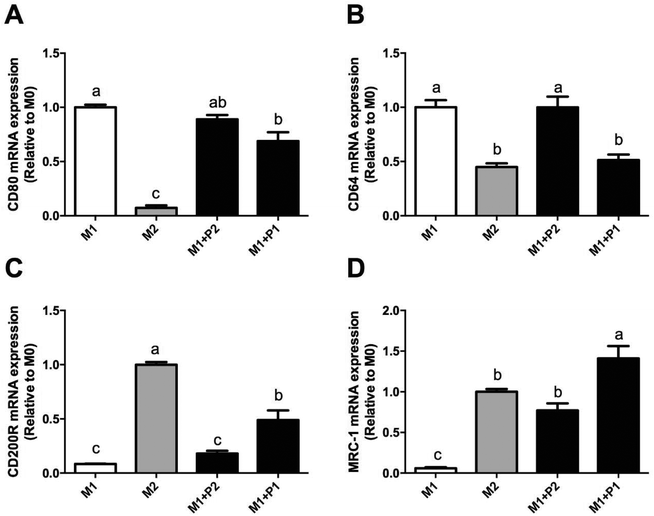 |
| Fig. 4 Effect of GPETAFLR on M1/M2 macrophage polarisation. M0 macrophages were incubated with LPS plus IFNγ (M1 control), IL-4 (M2 control), or with LPS plus IFNγ plus GPETAFLR (50–100 μg mL−1, Peptide 2 and Peptide 1, respectively) for additional 24 h. Relative expression of (A) CD80, (B) CD64, (C) CD200R, and (D) MRC-1 genes were measured by RT-qPCR. Values are presented as means ± SD (n = 3) and those marked with different letters are significantly different (P < 0.05). | |
M0 macrophages were incubated with LPS plus IFNγ (M1), IL-4 (M2) or LPS plus IFNγ plus GPETAFLR peptide (M1 + P) to study the phenotype plasticity. M0 macrophages treated with LPS plus IFNγ showed an increased in CD80 and CD64 mRNA levels (Fig. 4A and B, respectively) and a decrease in CD200R and MRC-1 mRNA levels (Fig. 4C and D, respectively). M0 macrophages treated with LPS plus IFNγ plus GPETAFLR peptide down-regulated CD80 and CD64 mRNA levels compared to M1 macrophages, whereas CD200R and MRC-1 mRNA levels were up-regulated compared to M1 macrophages. The results at 100 μg mL−1 were more remarkable than with 50 μg mL−1. In addition, cytokine production in the macrophage phenotype was also studied (Fig. 5). TNFα, IL-1β, and IL-6 cytokine levels were increased in M1 macrophages (Fig. 5A, B and C, respectively). M0 macrophages treated with LPS plus IFNγ plus GPETAFLR peptide decreased the pro-inflammatory activity of the M1 phenotype, whereas IL-10 cytokine levels were increased compared to M1 macrophages (Fig. 5D).
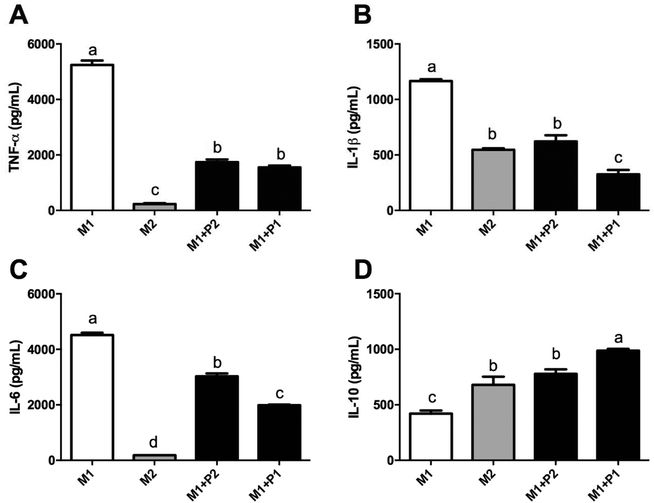 |
| Fig. 5 Effect of GPETAFLR on expression of cytokines in M1 macrophages. M0 macrophages were incubated with LPS plus IFNγ (M1 control), IL-4 (M2 control), or with LPS plus IFNγ plus GPETAFLR (50–100 μg mL−1, Peptide 2 and Peptide 1, respectively) for additional 24 h. Relative expression of (A) TNFα, (B) IL-1β, (C) IL-6, and (D) IL-10 genes were measured using RT-qPCR. Values are presented as means ± SD (n = 3) and those marked with different letters are significantly different (P < 0.05). | |
Discussion
In a world where resources are scarce, food plant processing residues are an alternative source to find proteins or peptides with bioactive properties.21 Plant-derived bioactive peptides are very interesting compounds that can be used with potential pharmaceutical and nutraceutical benefit.22
In the literature, it is possible to find a large number of examples where plant-derived biopeptides are used as anti-inflammatory or antioxidant compounds. One of them is 1,2,3,4,6 penta-O-galloyl-β-D-glucose, a naturally occurring polyphenolic compound present in some medicinal herbs as Rhuschinensis Mill.23Fagopyrum tataricum, commonly known as buckwheat, is another example of a bioactive plant. Researchers found that buckwheat extracts may inhibit adipogenesis and inflammatory response during adipocyte differentiation of 3T3–L1 cells.24 Brazilian red propolis (Apis mellifera), Copaifera oleoresins, flavonoid fraction of bergamot juice (Citrus bergamia), effusanin C (Isodon japonicus), oligomeric proanthocyanidins (Crataegus oxyacantha) are other isolated compounds with anti-inflammatory actions in activated monocytes and macrophages.25–29
Lupinus angustifolius L. is a legume that contents a high level of plant protein.17 The GPETAFLR peptide was isolated from a lupine protein hydrolysate. This biopeptide showed an anti-inflammatory effect in different line cells such as osteoclasts30 and THP-1-derived macrophages.17,31 However, it is the first time that the anti-inflammatory activity of GPETAFLR was shown on primary monocytes.
Macrophage polarisation depends on different microenvironments and signals to promote specific phenotypes. These phenotypes correspond to classically (M1) and alternatively (M2) polarized macrophages that exhibit pro-inflammatory and anti-inflammatory functions, respectively.32–34 In the present study, a modulation capacity of GPETAFLR on macrophage polarization was observed. This could be explained by the fact that the GPETAFLR peptide increase anti-inflammatory cytokines such as IL-10 while decreasing pro-inflammatory as IL-6 or IL-1β. These cytokines regulate and modulate the macrophage phenotype, stimulating the M1 phenotype in the case of IL-1β or M2 phenotype in the case of IL-10.35,36
GPETAFLR could also modulate monocyte subsets. Under LPS stimulation, classical monocytes (monocytes that promote inflammatory reactions) increased their population while non-classical monocytes suffer a decrease with respect to monocytes treated with the peptide. This mean that GPETAFLR can modulate the monocyte subset ratio, stimulating those monocytes that participate in the anti-inflammatory process and decreasing classical monocytes.37,38
In conclusion, the GPETAFLR peptide showed an anti-inflammatory effect in monocyte cells. Our findings imply a new understanding of the mechanisms by which GPETAFLR favors a continuous and gradual plasticity process in the human monocyte/macrophage system and offer novel benefits derived from the consumption of Lupinus angustifolius L. in the prevention of inflammatory-related diseases.
Abbreviations
CCL | CC chemokine ligand |
CCR | CC chemokine receptor |
CD | Cluster of differentiation |
GPETAFLR | Gly-Pro-Glu-Thr-Ala-Phe-Leu-Arg |
HLA-DR | Human leukocyte antigen–antigen D related |
M-CSF | Macrophage colony-stimulating factor |
TLR | Toll-like receptor |
TNF | Tumour necrosis factor |
Conflicts of interest
All the authors declare that they do not have conflicts of interest.
Acknowledgements
This work was supported by grant AGL2012-40247-C02-01 from the Spanish Ministry of Economy and Competitiveness. Authors thank the Cell Biology Unit of Instituto de la Grasa for its assistance during the fulfilment of this work. SMP acknowledges financial support from “V Own Research Plan” (University of Seville).
References
- A. Santini, S. M. Cammarata, G. Capone and A. Ianaro,
et al., Nutraceuticals: opening the debate for a regulatory framework, Br. J. Clin. Pharmacol., 2018, 84, 659–672 CrossRef PubMed.
- R. Khound, J. Shen, Y. Song and D. Santra,
et al., Phytoceuticals in Fenugreek Ameliorate VLDL Overproduction and Insulin Resistance via the Insig Signaling Pathway, Mol. Nutr. Food Res., 2017, 62, 1700541 CrossRef PubMed.
- S. Montserrat-de la Paz, M. A. Fernandez-Arche, M. Angel-Martin and M. D. Garcia-Gimenez, Phytochemical characterization of potential nutraceutical ingredients of Evening Primrose oil (Oenothera biennis L.), Phytochem. Lett., 2014, 8, 158–162 CrossRef CAS.
- S. Y. Lee and S. J. Hur, Antihypertensive peptides from animal products, marine organisms, and plants, Food Chem., 2017, 228, 506–517 CrossRef CAS PubMed.
- S. Montserrat-de la Paz, D. Rodriguez, M. P. Cardelo and M. C. Naranjo,
et al., The effects of exogenous fatty acids and niacin on human monocyte-macrophage plasticity, Mol. Nutr. Food Res., 2017, 61, 1600824 CrossRef PubMed.
- H. Xiong and E. G. Pamer, Monocytes and infection: Modulator, messenger and effector, Immunobiology, 2015, 220, 210–214 CrossRef CAS PubMed.
- M. C. Naranjo, I. Garcia, B. Bermudez and S. Lopez,
et al., Acute effects of dietary fatty acids on osteclastogenesis via RANKL/RANK/OPG system, Mol. Nutr. Food Res., 2016, 60, 2505–2513 CrossRef CAS PubMed.
- S. Montserrat-de la Paz, M. C. Naranjo, S. Lopez and R. Abia,
et al., Niacin and its metabolites as master regulators of macrophage activation, J. Nutr. Biochem., 2017, 39, 40–47 CrossRef CAS PubMed.
- M. C. Millán-Linares, B. Bermudez, M. E. Martin and E. Muñoz,
et al., Unsaponifiable fraction isolated from grape (Vitis vinifera L.) seed oil attenuates oxidative and inflammatory responses in human primary monocytes, Food Funct., 2018, 9, 2517–2523 RSC.
- C. Porta, E. Riboldi, A. Ippolito and A. Sica, Molecular and epigenetic basis of macrophage polarized activation, Semin. Immunol., 2015, 27, 237–248 CrossRef CAS PubMed.
- S. Montserrat-de la Paz, R. De la Puerta, A. Fernandez-Arche and A. M. Quilez,
et al., Pharmacological effects of mitraphylline from Uncaria tomentosa in primary human monocytes: Skew toward M2 macrophages, J. Ethnopharmacol., 2015, 170, 128–135 CrossRef CAS PubMed.
- P. Italiani and D. Boraschi, From Monocytes to M1/M2 Macrophages: Phenotypical vs. Functional Differentiation, Front. Immunol., 2014, 5, 514 Search PubMed.
- K. L. Wong, W. H. Yeap, J. J. Tai, S. M. Ong, T. M. Dang and S. C. Wong, The three human monocyte subsets: implications for health and disease, Immunol. Res., 2012, 53, 41–57 CrossRef CAS PubMed.
- G. Thomas, R. Tacke, C. C. Hedrick and R. N. Hanna, Nonclassical Patrolling Monocyte Function in the Vasculature, Arterioscler., Thromb., Vasc. Biol., 2015, 35, 1306–1316 CrossRef CAS PubMed.
- M. Hristov and G. H. Heine, Monocyte subsets in atherosclerosis, Hamostaseologie, 2015, 35, 105–112 CrossRef CAS PubMed.
- M. Benoit, B. Desnues and J. L. Mege, Macrophage polarization in bacterial Infections, J. Immunol., 2008, 181, 3733–3739 CrossRef CAS.
- M. C. Millán-Linares, F. Millán, J. Pedroche and M. M. Yust, GPETAFLR: A new anti-inflammatory peptide from Lupinus angustifolius L. protein hydrolysate, J. Funct. Foods, 2015, 18, 358–367 CrossRef.
- M. M. Yust, J. Pedroche, M. C. Millán-Linares, J. M. Alcaide-Hidalgo and F. Millán, Improvement of functional properties of chickpea proteins by hydrolysis with immobilized Alcalase, Food Chem., 2010, 122, 1212–1217 CrossRef CAS.
- M. C. Millán-Linares, M. M. Yust, J. M. Alcaide-Hidalgo, F. Millán and J. Pedroche, Lupine protein hydrolysates inhibit enzymes involved in the inflammatory pathway, Food Chem., 2014, 151, 141–147 CrossRef PubMed.
- S. Montserrat-de la Paz, A. Fernández-Arche, M. Angel-Martín and M. D. García-Giménez, The sterols isolated from Evening Primrose oil modulate the release of proinflammatory mediators, Phytomedicine, 2012, 19, 1072–1076 CrossRef CAS PubMed.
- H. Hou, Y. Fan, B. Li and C. Xue,
et al., Purification and identification of immunomodulating peptides from enzymatic hydrolysates of Alaska pollock frame, Food Chem., 2012, 134, 821–828 CrossRef CAS PubMed.
- E. Maestri, M. Marmiroli and N. Marmiroli, Bioactive peptides in plant-derived foodstuffs, J. Proteomics, 2016, 147, 140–155 CrossRef CAS PubMed.
- P. Mendonca, E. Taka, D. Bauer and M. Cobourne-Duval,
et al., The attenuating effects of 1,2,3,4,6 penta-O-galloyl-β-D-glucose on inflammatory cytokines release from activated BV-2 microglial cells, J. Neuroimmunol., 2017, 305, 9–15 CrossRef CAS PubMed.
- M. K. Lee, Y. Shin, S. Jung and S. Y. Kim,
et al., The Inhibitory Effect of Tartary Buckwheat Extractson Adipogenesis and Inflammatory Response, Molecules, 2017, 22, 1160 CrossRef PubMed.
- B. Bueno-Silva, D. Kawamoto, E. S. Ando-Suguimoto and S. M. Alencar,
et al., Brazilian Red Propolis Attenuates Inflammatory Signaling Cascade in LPS Activated, PLoS One, 2015, 10, e0144954 CrossRef PubMed.
- K. B. Santiago, B. J. Conti, B. F. M. Teles and J. J. Mangabeira da Silva,
et al., Immunomodulatory action of Copaifera spp oleoresins on cytokine production by human monocytes, Biomed. Pharmacother., 2015, 70, 12–18 CrossRef CAS PubMed.
- R. Risitano, M. Curro, S. Cirmi and N. Ferlazzo,
et al., Flavonoid Fraction of Bergamot Juice Reduces LPS Induced inflammatory Response through SIRT1-Mediated NF-kB Inhibition in THP-1 Monocytes, PLoS One, 2014, 9, e107431 CrossRef PubMed.
- J. Y. Kim, H. S. Kim, Y. J. Kim and H. K. Lee,
et al., Effusanin C inhibits inflammatory responses via blocking NF-κB and MAPK signalingin monocytes, Int. Immunopharmacol., 2013, 15, 84–88 CrossRef CAS PubMed.
- T. Mohana, A. V. Navin, S. Jamuna and M. S. Sakeena,
et al., Inhibition of differentiation of monocyte to macrophages in atherosclerosis by oligomeric proanthocyanidins –In vivo and in vitro study, Food Chem. Toxicol., 2015, 82, 96–105 CrossRef CAS PubMed.
- M. C. Millán-Linares, A. Lemus-Conejo, M. M. Yust and J. Pedroche,
et al., GPETAFLR, a novel bioactive peptide from Lupinus angustifolius L. protein hydrolysate, reduces osteoclastogenesis, J. Funct. Foods, 2018, 47, 299–303 CrossRef.
- M. C. Millán-Linares, B. Bermúdez, M. M. Yust and F. Millán,
et al., Anti-inflammatory activity of lupine (Lupinus angustifolius L.) protein hydrolysates in THP-1-derived macrophages, J. Funct. Foods, 2014, 8, 224–233 CrossRef.
- Y. C. Liu, X. B. Zou, Y. F. Chai and Y. M. Yao, Macrophage Polarization in Inflammatory Diseases, Int. J. Biol. Sci., 2014, 10, 520–529 CrossRef PubMed.
- A. Sica, M. Erreni, P. Allavena and C. Porta, Macrophage polarization in pathology, Cell. Mol. Life Sci., 2015, 72, 4111–4126 CrossRef CAS PubMed.
- F. Chu, M. Shi, C. Zheng and D. Shen,
et al., The roles of macrophages and microglia in multiple sclerosis and experimental autoimmune encephalomyelitis, J. Neuroimmunol., 2018, 318, 1–7 CrossRef CAS PubMed.
- L. Zhu, Q. Zhao, T. Yang and W. Ding,
et al., Cellular Metabolism and Macrophage Functional Polarization, Int. Rev. Immunol., 2014, 34, 82–100 CrossRef PubMed.
- M. Locati, A. Mantovani and A. Sica, Macrophage Activation and Polarization as an Adaptive Component of Innate Immunity, Adv. Immunol., 2013, 120, 163–184 CAS.
- L. Ziegler-Heitbrock, Monocyte subsets in man and other species, Cell. Immunol., 2014, 289, 135–139 CrossRef CAS PubMed.
- K. L. Wong, W. H. Yeap, J. J. Yi Tai and S. M. Ong,
et al., The three human monocyte subsets: implications for health and disease, Immunol. Res., 2012, 53, 41–57 CrossRef CAS PubMed.
|
This journal is © The Royal Society of Chemistry 2019 |