DOI:
10.1039/C8FO02091D
(Paper)
Food Funct., 2019,
10, 344-354
Resveratrol improves intestinal barrier function, alleviates mitochondrial dysfunction and induces mitophagy in diquat challenged piglets1
Received
25th October 2018
, Accepted 13th December 2018
First published on 17th December 2018
Abstract
This study evaluated whether resveratrol can alleviate intestinal injury and enhance the mitochondrial function and the mitophagy level in diquat induced oxidative stress of piglets. Twenty-four 35 day old piglets were randomly allocated to four groups: (1) nonchallenged control; (2) control + 100 mg kg−1 resveratrol diet; (3) diquat-treated control and (4) diquat + 100 mg kg−1 resveratrol diet. At the beginning of the experiment, the piglets were injected with diquat or saline (10 mg per kg bodyweight). On day 14, the piglets were killed to obtain the jejuna segments. The results showed that resveratrol increased (P < 0.05) the total antioxidant capacity (T-AOC) and decreased (P < 0.05) the hydrogen peroxide (H2O2) and malondialdehyde (MDA) levels in the jejunal mucosa, in comparison with the diquat group. Resveratrol improved (P < 0.05) the intestinal barrier function, as indicated by the increased transepithelial electrical resistance and the decreased paracellular permeability of fluorescein isothiocyanate dextran 4 kDa in the jejunum. Moreover, resveratrol prevented (P < 0.05) the diquat induced decline of occludin, claudin-1 and ZO-1 levels in the jejunal mucosa. Resveratrol ameliorated mitochondria swelling, vacuolation and cracked cristae induced by diquat. Resveratrol decreased (P < 0.05) the ROS generation and increased (P < 0.05) the membrane potential of intestinal mitochondria, the content of mitochondrial DNA and the activity of mitochondrial complexes I–IV in the jejunum. Finally, resveratrol enhanced (P < 0.05) the level of PTEN induced putative kinase 1 (PINK1) and Parkin in intestinal mitochondria; meanwhile it increased (P < 0.05) the LC3-II/LC3-I ratio in the jejunum. These data indicate that resveratrol is effective in protecting the intestinal barrier, improving the redox status, alleviating mitochondrial damage and inducing mitophagy in piglets challenged with diquat.
1. Introduction
Oxidative stress plays a vital role in human disease, particularly in inflammatory bowel disease and gastrointestinal mucosa diseases.1,2 The disharmony among the generation of the reactive oxygen species (ROS) and the antioxidative system would lead to serious oxidative stress.3 Superfluous ROS may destroy the redox balance, and then damage the proteins, lipids and DNA, leading to gut injury.4 Therefore, effective and safe therapeutic approaches are urgently needed to maintain the intestinal redox balance.
Resveratrol (trans-3,4,5-trihydroxystilbene) is a polyphenol isolated from peanuts, red wine, grapes, pistachios, and chocolate.5–7 Numerous studies have reported that resveratrol has efficient antioxidant activities in vitro8,9 and in vivo.10,11 However, the mechanism area is infrequently studied, except a few on free radical clearances.12 Generally, under an oxidative stress situation, ROS are overproduced, particularly in oxidative stress damaged mitochondria. Therefore, the powerful antioxidant effect of resveratrol may be due to the targeting of mitochondria, the primary source of ROS.
The intestine needs a great amount of energy to support its rapid self-renewal.13 Mitochondria are the principal ATP sources of the cells.14 However, mitochondria are a major source of ROS, as well as important targets of ROS action, which may generate the superfluous ROS.15 In turn, ROS overproduction leads to mitochondrial damage and disruption of the oxidation respiratory chain, eventually leading to the activation of cell death pathways.16 Recent research has demonstrated that cells develop a defence mechanism called mitophagy which can mediate the clearance of damaged mitochondria before they activate cell death.17 Numerous studies have illustrated that PINK1 and Parkin are involved in the regulation of mitophagy.17–19 PINK1 is located in healthy and polarized mitochondria and maintain a low level. Meanwhile, the accumulation of PINK1 on dysfunctional mitochondria is necessary to recruit Parkin to induce mitophagy.19 A latest study had reported that resveratrol exerts neuroprotective effects through activating mitophagy in β-amyloid challenged PC12 cells.20 Nevertheless, until now, no information is available that is relevant to the influence of resveratrol on mitochondrial function and mitophagy in oxidative stress induced intestinal injury of piglets. It would be of great interest to focus on whether resveratrol prevents intestinal injury induced by oxidative stress via advancing the mitochondrial function and the mitophagy level of piglets.
Hence, the purposes of this study were to determine whether resveratrol exerts its intestinal protection effect by modulating mitochondrial dysfunctions and associated mitophagy during oxidative stress induced intestinal damage. In the present study, diquat was injected to establish our model of intestinal oxidative stress.21,22 According to our previous report,23 we took advantage of a well-characterized model of intestinal injury induced by oxidative stress in piglets, to elaborate whether resveratrol could relieve intestinal damage, improve mitochondrial dysfunction and induce mitophagy.
2. Materials and methods
2.1 Animals and experiment design
All animal procedures were performed in accordance with the Guidelines for Care and Use of Laboratory Animals of Zhejiang University and the experiments were approved by the Animal Ethics Committee of Zhejiang University. Twenty-four piglets (35 d, weaned at 21 d, Duroc × Landrace × Yorkshire) of the same average initial body weight of 9.35 ± 0.26 kg were randomly allotted to four treatments: (1) the non-challenged control group: piglets receiving a control diet and injected with 0.9% NaCl solution; (2) control + resveratrol: piglets fed the control diet with supplementation of 100 mg kg−1 resveratrol (Sigma-Aldrich, St Louis, USA); (3) diquat-challenged group: piglets receiving the control diet and injected with diquat (Sigma-Aldrich, St Louis, USA); (4) diquat + resveratrol: piglets fed the control diet with supplementation of 100 mg kg−1 resveratrol and administration of diquat. The diets were formulated in accordance with NRC (2012) (Table 1). At the beginning of the trial, the piglets were administered diquat or saline (10 mg kg−1 of BW) by intraperitoneal injection which was in accordance with previous studies.22,23 The piglets were individually placed in cages (1.8–1.2 m2). Feed and water were freely available.
Table 1 Ingredient and chemical composition of the basal diet based on feed
Item, g kg−1 |
|
Provided per kilogram of diet: vitamin A, 8000 IU; vitamin D, 2000 IU; vitamin E, 40 IU; vitamin K3, 1.5 mg; vitamin B1, 1.5 mg; vitamin B6, 1.5 mg; biotin, 0.10 mg; niacin, 30 mg; pantothenic acid, 25 mg; Zn, 80 mg; Fe, 100 mg; Cu, 20 mg; Mn, 20 mg; I, 0.14 mg; Se, 0.3 mg.
Digestible energy was calculated from the data provided by Feed Database in China (2012).
|
Maize |
530 |
Extruded maize |
140 |
Soybean meal |
130 |
Extruded soybean |
110.3 |
Fish meal |
40.5 |
Soybean oil |
19 |
Limestone |
6 |
Dicalcium phosphate |
9 |
Sodium chloride |
3 |
L-Lysine hydrochloride |
1.5 |
DL-Methionine |
0.7 |
Vitamin–mineral premixa |
10 |
Analysed composition, g kg−1 |
|
Digestible energyb, MJ kg−1 (calculated) |
14.59 |
Crude protein |
205.53 |
Lysine |
13.88 |
Methionine |
4.40 |
Calcium |
8.51 |
2.2 Sample collection
After overnight fasting, on day 14, the piglets were euthanized with sodium pentobarbital (200 mg per kg BW) (Sigma-Aldrich, St Louis, USA) in accordance with previous research studies24,25 and the jejunum was rapidly removed. The proximal jejunum was used for the separation of mitochondria and the Ussing chamber essay. About 10 cm portions of the adjacent jejunum were opened longitudinally and cleaned with PBS. Mucosal samples were collected by scratching with a glass slide from the connective tissue, snap-frozen in liquid nitrogen and then stored at −80 °C.
2.3 Measurement of the redox status in the jejunum
In accordance with the manufacturer's guidelines, the total antioxidant capacity (T-AOC) and the level of hydrogen peroxide (H2O2) and malondialdehyde (MDA) in the jejunal mucosa were determined using ELISA kits from Nanjing Jiancheng Bioengineering Institute (Nanjing, China) using a fluorescence microplate reader (Bio-Tek Instruments, Inc.).
2.4 Intestinal barrier function measurements
The intestinal barrier function was measured using an Ussing chamber (model VCC MC6; Physiologic Instruments, San Diego, CA, USA) and all procedures were in accordance with those previously reported.26–28
2.5 Intestinal mitochondrial isolation
Fresh jejunum was used for mitochondrial preparation. All procedures followed the guidance of mitochondria isolating kits (Beyotime Institute of Biotechnology, China).
2.6 Ultrastructure of intestinal mitochondria
Fresh jejunal segments were fixed in 2.5% glutaraldehyde in PBS for 4 h. After dehydrating in different concentrations of ethanol and alcohol and embedding in the Spurr resin, ultrathin sections were stained with uranyl acetate and alkaline lead citrate for 5 to 10 min, and the specimen was placed in Eppendorf tubes containing the Spurr resin and heated at 70 °C for more than 9 h. The specimen was sectioned using a LEICA EM UC7 ultratome (Leica UC7) and the sections were stained with uranyl acetate and alkaline lead citrate for 5 to 10 min, respectively, and observed using a Hitachi Model H-7650 TEM (Hitachi, Tokyo, Japan). Glutaraldehyde, PBS, ethanol, acetone, lead citrate, and methylene blue were purchased from Sinopharm Chemical Reagent Co., Ltd.
2.7 Intestinal mitochondrion ROS production measurement
The intestinal mitochondria were treated with 2 μmol L−1 2′,7′-dichlorohydro-fluorescein diacetate and incubated at 24 °C for 20 min, and then the fluorescence intensity was determined using a fluorescence microplate reader as described.23,29
2.8 Measurement of the intestinal mitochondrial membrane potential (ΔΨm)
The ΔΨm was determined using ΔΨm assay kits (Beyotime Institute of Biotechnology, China) and all methods were in accordance with the guidelines. When the mitochondrial membrane potential is high, JC-1 aggregates into the matrix of the mitochondria, forming a polymer (J-aggregates), which can produce red fluorescence; when the mitochondrial membrane potential is low, JC-1 cannot aggregate in the mitochondria. In the matrix, JC-1 is a monomer at this time, and green fluorescence can be produced. The ΔΨm of intestinal mitochondria was measured by the fluorescence ratio of aggregates to monomers using a fluorescence microplate reader (Bio-Tek Instruments, Inc.).30–32
2.9 Determination of the mtDNA content in the jejunum
The mtDNA content present per nuclear genome was measured by amplifying the mitochondrial DNA-loop and β-actin using real-time PCR measurements, analyzing with a standard curve as mentioned in a previous study.33 The primers are presented in Table 2.
Table 2 Primer sequences used for real-time PCR
Gene |
5′-Primer (F) |
3′-Primer (R) |
Accession number |
Length |
mt D-loop, mitochondrial DNA-loop. |
β-Actin |
CTGCGGCATCCACGAAACT |
AGGGCCGTGATCTCCTTCTG |
DQ845171.1 |
147 |
mt D-loop |
GATCGTACATAGCACATATCATGTC |
GGTCCTGAAGTAAGAACCAGATG |
AF276923 |
198 |
2.10 The activities of respiratory chain complexes I–IV in intestinal mitochondria
The activities of mitochondrial respiratory chain complexes I (NADH-CoQ reductase), II (succinate-CoQ reductase), III (CoQ-cytochrome C reductase), and IV (cytochrome C oxidase) were measured by using quantitative determination kits in accordance with the guidance (GenMed Scientifics, China) from a previous study.34
2.11 Western blot analysis
The method of performing western blot was outlined by Xiao et al. (2016).28 After the proteins were separated by electrophoreses, all samples were shifted to polyvinylidene difluoride membranes (Beyotime Institute of Biotechnology, China). The membranes were incubated with the first Abs (occludin, claudin-1, ZO-1, LC3 I, II, Parkin, PINK1, VDAC, GAPDH) (Abcam, Inc, USA) for 12–16 h at 4 °C and then with the HRP conjugated anti-rabbit Ab (HuaBio, Hangzhou, China) for 2 h at 21–25 °C. Western blot was monitored by using a chemiluminescence detection reagent (Beyotime Institute of Biotechnology, China) and visualized using Chemi Scope 3400 (ClinxScience Instruments, China). For quantification, GAPDH and VDAC were used as the inner standards of intestinal proteins and mitochondrial proteins, respectively.
2.12 Statistical analysis
All statistics were analysed using SPSS 19.0 statistical package (SPSS Inc., Chicago, IL). The significance between treatments was determined with Duncan's multiple range test. The result was considered significant if P < 0.05.
3. Results
3.1 Effect of dietary resveratrol on the growth performance of piglets after diquat challenge
Compared with the nonchallenged control piglets, the supplementation of dietary resveratrol in the control piglets had no (P > 0.05) effect on ADG (average daily gain) and ADFI (average daily feed intake), while piglets injected with diquat had lower (P < 0.05) ADG and ADFI (Table 3). The supplementation of resveratrol in diquat treated piglets prevented diquat induced decline of ADG and ADFI.
Table 3 Effects of dietary resveratrol supplementation on the growth performance of piglets1 (mean values with their standard errors; n = 6 piglets)
Items |
Control |
Control + resv |
Diquat |
Diquat ± resv |
SEM |
ADG = average daily gain.
ADFI = average daily feed intake.
|
ADGa, g |
503.98a |
504.05a |
309.72c |
355.99b |
11.61 |
ADFIb, g |
724.89a |
730.22a |
518.55c |
584.99b |
17.68 |
3.2 Effect of dietary resveratrol on the redox status in the jejunum of piglets after diquat challenge
Compared with the piglets injected with saline, the supplementation of resveratrol in the control piglets had no (P > 0.05) effect on the T-AOC and the level of H2O2 and MDA, while piglets injected with diquat had a lower (P < 0.05) T-AOC, and higher (P < 0.05) H2O2 and MDA levels in the jejunum (Table 4). Dietary resveratrol in diquat injected piglets prevented (P < 0.05) the diquat induced decline of T-AOC and increase of the H2O2 and MDA levels in the jejunum.
Table 4 Effects of dietary resveratrol supplementation on the redox status in the jejunum of piglets1 (mean values with their standard errors; n = 6 piglets)
Items |
Control |
Control + resv |
Diquat |
Diquat ± resv |
SEM |
T-AOC, total antioxidant capacity; H2O2, hydrogen peroxide; MDA, malondialdehyde. a,b,cMeans within a row with different letters differ significantly (P < 0.05). Control (non-challenged control), piglets receiving a control diet and injected with 0.9% (w/v) NaCl solution; control + resv, piglets injected with saline at 10 mg per kg body weight and receiving a control diet supplemented with 100 mg kg−1 resveratrol; diquat (diquat challenged control), piglets receiving the same control diet and injected with diquat at 10 mg per kg body weight; diquat + resv, piglets injected with diquat at 10 mg per kg body weight and receiving a control diet supplemented with 100 mg kg−1 resveratrol. |
T-AOC, U mg−1 protein |
0.43a |
0.44a |
0.18c |
0.33b |
0.02 |
H2O2, mmol g−1 |
12.36c |
12.18c |
20.43a |
15.61b |
0.33 |
MDA, nmol mg−1 |
0.41b |
0.38b |
1.05a |
0.59b |
0.06 |
3.3 Effect of dietary resveratrol on the barrier function and occludin, claudin-1 and ZO-1 levels in the jejunum of piglets after diquat challenge
In comparison with the nonchallenged control piglets, the supplementation of dietary resveratrol in the control piglets did not (P > 0.05) influence the transepithelial electrical resistance (TER) and the flux of fluorescein isothiocyanate dextran (FD4) in the jejunum (Table 5), while piglets challenged with diquat had a lower (P < 0.05) TER and a higher (P < 0.05) flux of fluorescein isothiocyanate dextran (FD4) from the mucosa to the serosa in the jejunum compared with those injected with saline (Table 4). Supplementation with resveratrol in piglets treated with diquat prevents the diquat induced decline (P < 0.05) of TER and enhancement (P < 0.05) of the FD4 flux from the mucosa to the serosa in the jejunum compared with the diquat group. Moreover, in relation to piglets injected with saline, the supplementation of resveratrol in the control piglets had no (P > 0.05) impact on the occludin, claudin-1 and ZO-1 levels, while piglets injected with diquat had reduced (P < 0.05) occludin, claudin-1 and ZO-1 levels (Fig. 1). However, dietary resveratrol in piglets injected with diquat reversed (P < 0.05) the decrease of the occludin, claudin-1 and ZO-1 levels induced by diquat.
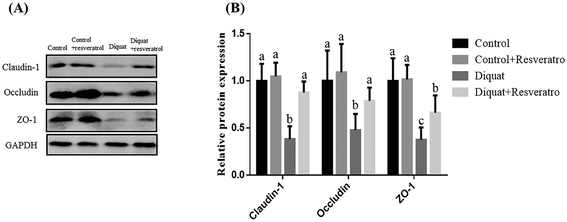 |
| Fig. 1 Effect of dietary resveratrol on tight junction proteins of piglets in the jejunum of piglets. (A) Representative blots of claudin-1, occludin, ZO-1 and β-actin in the jejunal mucosa of piglets. (B) Summary of western blot for n = 6 piglets per treatment in diquat-injected piglets and control piglets. Values are means and SD represented by vertical bars. a,b,c Means within a row with different letters differ significantly (P < 0.05). Control (non-challenged control), piglets receiving a control diet and injected with 0.9% (w/v) NaCl solution; control + resv, piglets injected with saline at 10 mg per kg body weight and receiving a control diet supplemented with 100 mg kg−1 resveratrol; diquat (diquat challenged control), piglets receiving the same control diet and injected with diquat at 10 mg per kg body weight; diquat + resv, piglets injected with diquat at 10 mg per kg body weight and receiving a control diet supplemented with 100 mg kg−1 resveratrol. The protein expression of all samples was expressed as fold changes, calculated relative to the control group. | |
Table 5 Effect of dietary resveratrol supplementation on the jejunal barrier function of piglets1 (mean values with their standard errors; n = 6 piglets)
Items |
Control |
Control + resv |
Diquat |
Diquat + RSV |
SEM |
TER, transepithelial electrical resistance; FD4, fluorescein isothiocyanate dextran (4 kDa). a,b,cMeans within a row with different letters differ significantly (P < 0.05). Control (non-challenged control), piglets receiving a control diet and injected with 0.9% (w/v) NaCl solution; control + resv, piglets injected with saline at 10 mg per kg body weight and receiving a control diet supplemented with 100 mg kg−1 resveratrol; diquat (diquat-challenged control), piglets receiving the same control diet and injected with diquat at 10 mg per kg body weight; diquat + resv, piglets injected with diquat at 10 mg per kg body weight and receiving a control diet supplemented with 100 mg kg−1 resveratrol. |
TER, Ω cm2 |
60.61a |
60.71a |
46.35b |
55.52a |
2.24 |
FD4 flux, μg cm−2 h−1 |
1.16c |
1.14c |
2.45a |
1.67b |
0.13 |
3.4 Effect of dietary resveratrol on the ultrastructure of the intestinal mitochondria of piglets after diquat challenge
The ultrastructure of the intestinal mitochondria is shown in Fig. 2. Normomorph mitochondria with an integrated membrane and cristae mitochondria were observed in the piglets injected with saline and the control piglets fed resveratrol (Fig. 2A and B). In contrast, fractured and swollen mitochondria with broken and cracked cristae were observed in the diquat group (Fig. 2C), which were mostly prevented by resveratrol supplementation (Fig. 2D).
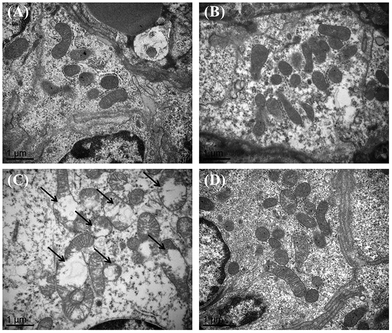 |
| Fig. 2 Effects of dietary resveratrol supplementation on the ultrastructure of intestinal mitochondria in piglets. Transmission electron micrographs of control (A), control + resv (B), diquat (C), and diquat + resv (D). Piglets’ intestinal sections are shown at 10 000× magnification. Control (non-challenged control), piglets receiving a control diet and injected with 0.9% (w/v) NaCl solution; control + resv, piglets injected with saline at 10 mg per kg body weight and receiving a control diet supplemented with 100 mg kg−1 resveratrol; diquat (diquat challenged control), piglets receiving the same control diet and injected with diquat at 10 mg per kg body weight; diquat + resv, piglets injected with diquat at 10 mg per kg body weight and receiving a control diet supplemented with 100 mg kg−1 resveratrol. The black arrows point to the mitochondria with an impaired ultrastructure. | |
3.5 Effect of dietary resveratrol on intestinal mitochondrial ROS production and ΔΨm of piglets after diquat challenge
In comparison to the nonchallenged control group, the supplementation of resveratrol in the control group had no (P > 0.05) impact on ROS generation and ΔΨm. After diquat challenge, mitochondrial ROS production was enhanced (P < 0.05) and ΔΨm declined (P < 0.05) in the jejunum in comparison with the piglets injected with saline. However, dietary resveratrol in piglets injected with diquat decreased (P < 0.05) mitochondrial ROS production and increased (P < 0.05) ΔΨm in jejunal mitochondria, compared with the diquat group (Fig. 3).
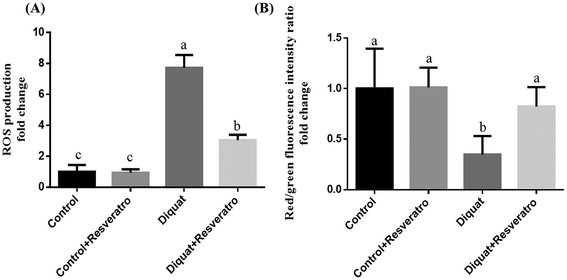 |
| Fig. 3 (A) Effect of dietary resveratrol supplementation on intestine mitochondrial ROS production of piglets. (B) Effect of dietary resveratrol supplementation on intestinal mitochondrial membrane potential change (ΔΨm) of piglets. Values are means and SD represented by vertical bars. a,b,c Means within a row with different letters differ significantly (P < 0.05). Control (non-challenged control), piglets receiving a control diet and injected with 0.9% (w/v) NaCl solution; control + resv, piglets injected with saline at 10 mg per kg body weight and receiving a control diet supplemented with 100 mg kg−1 resveratrol; diquat (diquat challenged control), piglets receiving the same control diet and injected with diquat at 10 mg per kg body weight; diquat + resv, piglets injected with diquat at 10 mg per kg body weight and receiving a control diet supplemented with 100 mg kg−1 resveratrol. | |
3.6 Effect of dietary resveratrol on the mtDNA content and activity of intestinal mitochondrial complexes I–IV in the jejunum after diquat challenge
Compared to the piglets injected with saline, the supplementation of dietary resveratrol in the control piglets did not (P > 0.05) impact the mtDNA content and activities of mitochondrial complexes I–IV, while the piglets challenged with diquat had a lower (P < 0.05) mtDNA level and activities of mitochondrial complexes I–IV in the jejunum (Fig. 4A and B). However, dietary resveratrol in piglets injected with diquat significantly prevented (P < 0.05) the diquat induced decrease of the mtDNA content and the activities of mitochondrial complexes I–IV in the jejunum (Fig. 4A and B).
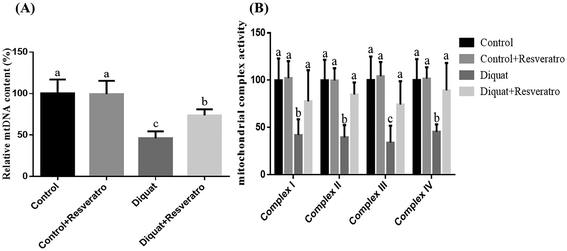 |
| Fig. 4 (A) Effect of dietary resveratrol supplementation on the intestinal content of mitochondrial DNA of piglets. (B) Effect of dietary resveratrol supplementation on the intestinal mitochondrial complex activity of piglets. Values are means and SD represented by vertical bars. a,b,c Means within a row with different letters differ significantly (P < 0.05). Control (non-challenged control), piglets receiving a control diet and injected with 0.9% (w/v) NaCl solution; control + resv, piglets injected with saline at 10 mg per kg body weight and receiving a control diet supplemented with 100 mg kg−1 resveratrol; diquat (diquat challenged control), piglets receiving the same control diet and injected with diquat at 10 mg per kg body weight; diquat + resv, piglets injected with diquat at 10 mg per kg body weight and receiving a control diet supplemented with 100 mg kg−1 resveratrol. | |
3.7 Effect of dietary resveratrol on the expression of PINK1, Parkin and LC3
Compared to piglets treated with saline, the supplementation of resveratrol in the control piglets and diquat treatment had no (P > 0.05) influence on the PINK1, Parkin and LC3 levels in the jejunum (Fig. 5A and B). Nevertheless, supplementation with resveratrol in the piglets treated with diquat significantly improved (P < 0.05) the expression of PINK1, Parkin, LC3II and LC3-II/LC3-I compared with the diquat group (Fig. 5A and B).
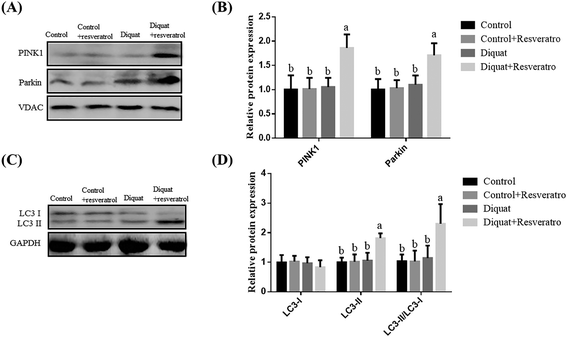 |
| Fig. 5 Effect of dietary resveratrol supplementation on the level of mitophagy-related protein in the jejunum of piglets. (A) Representative blots of PINK1 and Parkin in the intestinal mitochondria. (B) Relative PINK1 and Parkin expression. (C) Representative blots of LC3-I and LC3-II in the jejunum mucosa. (D) Relative LC3-I and LC3-II expression. Summary of western blot for n = 6 piglets per treatment in diquat-injected piglets and control piglets. Values are means and SD represented by vertical bars. a,b,c Means within a row with different letters differ significantly (P < 0.05). Control (non-challenged control), piglets receiving a control diet and injected with 0.9% (w/v) NaCl solution; control + resv, piglets injected with saline at 10 mg per kg body weight and receiving a control diet supplemented with 100 mg kg−1 resveratrol; diquat (diquat challenged control), piglets receiving the same control diet and injected with diquat at 10 mg per kg body weight; diquat + resv, piglets injected with diquat at 10 mg per kg body weight and receiving a control diet supplemented with 100 mg kg−1 resveratrol. | |
4. Discussion
In the present study, we took advantage of a model for inducing oxidative stress in piglets by injecting diquat to evaluate whether resveratrol supplementation could attenuate intestinal oxidative stress in piglets. In the current trial, diquat challenge severely decreased the performance of piglets, which is in accordance with the previous report,23 whereas the supplementation with resveratrol significantly reverses the decline of the growth performance of the piglets. Similarly, Zhang et al. (2017) had reported that resveratrol treatment improved the feed efficiency of intrauterine growth retarded suckling piglets.46 Resveratrol (3,5,4′-trihydroxystilbene) is a natural polyphenol belonging to the phytoalexin family. Its antioxidant function has been shown in multiple in vitro and in vivo studies.35,36 Moreover, increasing evidence suggested that resveratrol can protect the intestinal mucosal–epithelial barrier in the ischemia/reperfusion rat model, due to its antioxidant capacity.5,10 So far, the information regarding the effect of supplementation with resveratrol on intestinal injury induced by the oxidative stress of piglets is unavailable. Thus, we took advantage of a well-proven model that used diquat to lead to oxidative stress, which could lead to intestinal dysfunction and nutrients metabolism dysfunction.21,22,37 In the present study, we found that dietary resveratrol in piglets injected with diquat alleviates the oxidative stress of piglets treated with diquat, reflecting higher T-AOC activity and lower H2O2 and MDA levels in the jejunal mucosa. Consistently, Samsamikor et al. (2016) had suggested that resveratrol significantly increased SOD and T-AOC and decreased the MDA content in the serum of patients with ulcerative colitis.11 Similarly, supplementation with enteral resveratrol attenuates the release of inducible nitric oxide synthase under experimental necrotizing enterocolitis.38
An integrated intestinal barrier is important for the host health.39 In this trial, the intestinal barrier function was measured by an ex vivo Ussing chamber to detect the TER and paracellular flux of FD4. The TER could reflect the opening of the tight junctions between epithelial cells and the paracellular permeability of the intestinal mucosa. The flux of FD4 across the intestinal epithelium occurs mainly through paracellular pathways. A decreased TER and increased flux of FD4 reflect an impaired intestinal barrier in the revised manuscript. In the current experiment, the data indicate that the administration of diquat impaired the intestinal barrier, which is in accordance with previous studies.21,23,40 However, dietary resveratrol in piglets treated with diquat increased the TER and decreased the flux of FD4 relative to the diquat group. Similarly, Zhang et al. (2017) had shown that supplementation with resveratrol exerts a protective action as indicated by lower serum D-lactic acid and FITC-D contents in heat stress broilers.41 Wang et al. (2016) had shown that supplementation with resveratrol decreased the lactulose/mannitol ratio of urine in obstructive jaundice rats, and increased the TEER of Caco-2 challenged with hydrogen peroxide, known as an oxidative stress model.42 The intestinal barrier is physically composed of epithelial cells linked by tight-junction proteins, such as occludin, claudin-1, and ZO-1, regulating the selective permeability between epithelial cells.43 Consistently, with improved intestinal barrier function, dietary resveratrol reversed the decreased occludin, claudin-1 and ZO-1 levels in the jejuna induced by diquat. Wang et al. (2016) had reported that pre-treatment with resveratrol prevented the decrease of the phosphorylation levels of occludin and ZO-1 stimulated by hydrogen peroxide.42 Therefore, dietary resveratrol alleviates the intestinal barrier function damage induced by the diquat-oxidative stress.
Mitochondria are the power plants of the cell via the formation of adenosine triphosphate (ATP) by the mitochondrial respiratory chain. In mitochondria, the basis for generating ATP is the complete mitochondrial ultrastructure, as indicated by the intact double membranes and cristae invaginations.44 The inner and outer mitochondrial membranes surround the inter-membrane space and matrix. Then, the invagination of the intima forms cristae in which the oxidative respiratory chain complexes are located.45 Therefore, the integrated cristae are tightly related to energy production of mitochondria. Until now, the data on the influence of resveratrol on the ultrastructure of mitochondria in the oxidative stress model of piglets was unavailable. So in the current experiment, we found that diquat-induced oxidative stress led to the breaking of cristae, vacuolization and turgidity of the mitochondrion. Then, we firstly demonstrated that dietary resveratrol in piglets injected with diquat ameliorated the diquat-induced ultrastructural dysfunction of mitochondria, reflected by mitochondria with intact membranes and regular-shaped cristae. Similarly, Zhang et al. (2017) had shown that resveratrol effectively decreased the intrauterine growth retardation-induced irregular-shaped mitochondria and disrupted cristae in the liver of piglets.46 The damaged mitochondrial morphology may be due to the overproduction of mitochondrial ROS.47 So in the present experiment, we determined, for the first time, the influence of resveratrol on mitochondrial ROS production in diquat-treated piglets. Our data showed that diquat challenge increased the mitochondrial ROS production of piglets, consistent with our previous report.23 However, we found that supplementation with resveratrol reversed the decreased ROS production of intestinal mitochondria of piglets injected with diquat. Danz et al. (2009) had reported that pretreatment with resveratrol can inhibit doxorubicin-induced mitochondrial ROS production in neonatal rat ventricular myocytes.48 Zhang et al. (2018) had reported that resveratrol exerts cardioprotective effects by decreasing mitochondrial ROS production against anoxia/reoxygenation-induced oxidative stress damage.49 According to the preceding results of the oxidative stress indicator, the probable reason for the lower production of mitochondrial ROS may be that resveratrol alleviated diquat induced the disruption of antioxidative enzymes to eliminate the overproduction of mitochondrial ROS immediately. Furthermore, ROS overgeneration by mitochondria will impair electron transport chain complexes.50 Disruption of electron transport chain complexes might result in an opening of the mitochondrial permeability transition pore, which can bring about depolarization of the mitochondrial membrane. In this trial, we found that dietary resveratrol can inhibit the loss of the mitochondrial membrane potential induced by diquat. Similarly, Wang et al. (2014) had shown that resveratrol significantly decreased the high fat diet induced loss of the mitochondrial membrane potential in regulatory T cells of mice. Wang et al. (2018) had found that resveratrol abrogated the loss of the mitochondrial membrane potential induced by β-amyloid in neuronal cell line PC12 cells.20 Thus, it is possible to believe that the advantageous influence of resveratrol on intestinal injury induced by oxidative stress may be partially by decreasing ROS production and alleviating the depolarization of intestinal mitochondria to improve mitochondrial morphology.
Several studies have reported that increased ROS generation by mitochondria and decreased ΔΨm of mitochondria can lead to mitochondrial DNA damage, which results in impaired electron transport chain complexes.50,51 In this experiment, our data suggested that dietary resveratrol in piglets injected with diquat prevented decreased mtDNA contents in the intestine. Consistently, Lagouge et al. (2006) had indicated that dietary resveratrol increased the mtDNA content in the gastrocnemius muscle of high-fat diet mice.52 Xu et al. (2012) had shown that resveratrol efficiently preserved the mtDNA in the hyperglycemia-induced oxidative damage of rat mesangial cells.8 Mitochondrial DNA encodes 13 subunits of the approximately 100 proteins that constitute the respiratory electron-transport chain, including complexes I–V, which are located at the inner membrane of mitochondria.53,54 Thus, the increased mutation of mtDNA will damage the oxidation respiratory chain complexes and then decrease energy production. To better clarify whether the protective effect of resveratrol on the intestinal injury induced by diquat is exerted by protecting mitochondrial oxidation respiratory chain function, we firstly determined the activities of oxidation respiratory chain complexes in diquat challenged piglets. In the current study, our results suggested that supplementation with resveratrol alleviates the decrease in activities of the oxidative phosphorylation complexes I, II, III, and IV induced by diquat in the intestine of piglets. Folbergrová et al. (2018) had shown that the application of resveratrol completely restrained the deletion of complex I activity of the immature brain during the epileptogenesis induced by pilocarpine of Wistar rats.55 Zhang et al. (2018) had reported that resveratrol efficiently suppressed the decrease in complex I activity upon reoxygenation in H9c2 cardiomyocytes that underwent anoxia/reoxygenation.49 Xu et al. (2012) had reported that supplementation with resveratrol inhibited the reduction of mitochondrial complex III activity induced by hyperglycemia in rat mesangial cells.8 We speculate that dietary resveratrol exerts intestinal protection function by inhibiting the overproduction of superoxide and the depolarization of intestinal mitochondria in diquat-treated piglets, which protect the mtDNA and the oxidation respiratory chain complexes, and as a result, maintain the energy generation system and intestinal integrity.
Mitophagy has been regarded as one of the important pathways to remove dysfunctional mitochondria before it causes cell death.16 It is a complex process where damaged mitochondria are taken up into vesicles coated with the autophagosome marker LC3.56 Nevertheless, no data are available regarding the influence of resveratrol on the mitophagy level during oxidative stress in piglets. Here, we determined for the first time the effect of resveratrol on mitophagy levels in diquat challenged piglets, as indicated by the measurement of the protein levels of LC3, PINK-1 and Parkin. Currently, there is an abundance of studies that have illustrated that PINK1 and Parkin are involved in regulating mitophagy.17–19 PINK1 is located on healthy and polarized mitochondria and maintained at a low level. Meanwhile, the accumulation of PINK1 on dysfunctional mitochondria is necessary to recruit Parkin to induce mitophagy.19 During the inception phase of mitophagy, the LC3-I was converted into LC3-II with a phosphatidylethanolamine-conjugated form and translocated to the membrane of the autophagosome.57 In the present experiment, we found that resveratrol excited mitophagy in diquat induced oxidative stress, reflected by the enhanced expression of PINK1, Parkin and LC3-II/LC3-I, compared with diquat injected piglets. Similarly, Wang et al. (2018) had shown that resveratrol can activate mitophagy to attenuate oxidative damage in β-amyloid challenged PC12 cells.20 Wu et al. (2016) had reported that resveratrol induced mitophagy to alleviate the activation of the NLRP3 inflammasome in SV40 immortalised human peritoneal mesothelial cells challenged by a conventional high glucose-based peritoneal dialysis solution.58 So we speculate that resveratrol can induce mitophagy to eliminate the dysfunctional mitochondria caused by oxidative stress, which can protect cells from apoptosis induced by damaged mitochondria, and then maintain intestinal homeostasis.
In conclusion, the current trial demonstrated that supplementation with resveratrol attenuates intestinal damage induced by oxidative stress and in diquat-challenged piglets. Additionally, resveratrol may protect intestinal mitochondrial function by improving the intestinal mitochondrial ultrastructure, decreasing ROS production, and increasing mtDNA content, mitochondrial membrane potential and intestinal mitochondrial complex activity. Meanwhile, resveratrol enhances the level of mitophagy in intestinal mitochondria to degrade the damaged mitochondria induced by diquat challenge. These findings imply that resveratrol can induce mitophagy in the intestine to alleviate the intestinal barrier dysfunction induced by oxidative stress.
Conflicts of interest
The authors declare no competing financial interest.
Acknowledgements
This research was supported by the National Key R & D Program (2016YFD0501210), the National Natural Science Foundation of China (31872387, 31472103) and the Major Science and Technology Projects in Henan Province (171100110500).
References
- A. Bhattacharyya, R. Chattopadhyay, S. Mitra and S. E. Crowe, Oxidative stress: an essential factor in the pathogenesis of gastrointestinal mucosal diseases, Physiol. Rev., 2014, 94, 329–354 CrossRef CAS PubMed.
- H. Zhu and Y. R. Li, Oxidative stress and redox signaling mechanisms of inflammatory bowel disease: updated experimental and clinical evidence, Exp. Biol. Med., 2012, 237, 474–480 CrossRef CAS PubMed.
- H. Sies, Oxidative stress: a concept in redox biology and medicine, Redox Biol., 2015, 4, 180–183 CrossRef CAS PubMed.
- A. Rahal, A. Kumar, V. Singh, B. Yadav, R. Tiwari, S. Chakraborty and K. Dhama, Oxidative stress, prooxidants, and antioxidants: the interplay, BioMed Res. Int., 2014, 761264 Search PubMed.
- O. V. Ozkan, M. F. Yuzbasioglu, H. Ciralik, E. B. Kurutas, Z. Yonden, M. Aydin, E. Bulbuloglu, E. Semerci, M. Goksu, Y. Atli, V. Bakan and N. Duran, Resveratrol, a natural antioxidant, attenuates intestinal ischemia/reperfusion injury in rats, Tohoku J. Exp. Med., 2009, 218, 251–258 CrossRef CAS PubMed.
- P. E. B. Ferreira, E. J. Beraldi, S. C. Borges, M. R. M. Natali and N. C. Buttow, Resveratrol promotes neuroprotection and attenuates oxidative and nitrosative stress in the small intestine in diabetic rats, Biomed. Pharmacother., 2018, 105, 724–733 CrossRef CAS PubMed.
- K. V. Kiselev, Perspectives for production and application of resveratrol, Appl. Microbiol. Biotechnol., 2011, 90, 417–425 CrossRef CAS PubMed.
- Y. Xu, L. Nie, Y. G. Yin, J. L. Tang, J. Y. Zhou, D. D. Li and S. W. Zhou, Resveratrol protects against hyperglycemia-induced oxidative damage to mitochondria by activating sirt1 in rat mesangial cells, Toxicol. Appl. Pharmacol., 2012, 259, 395–401 CrossRef CAS PubMed.
- E. K. Hao, F. F. Lang, Y. Chen, H. L. Zhang, X. Cong, X. Q. Shen and G. H. Su, Resveratrol alleviates endotoxin-induced myocardial toxicity via the NRF2 transcription factor, PLoS One, 2013, 8, e69452 CrossRef CAS PubMed.
- C. Cucolas, A. I. Daneasa, D. Olteanu, N. Decea, R. Moldovan, F. Tabaran and G. A. Filip, Resveratrol and curcumin as protective agents in an experimental rat model of intestinal ischemia and reperfusion, Can. J. Physiol. Pharmacol., 2016, 94, 1151–1158 CrossRef CAS PubMed.
- M. Samsamikor, N. E. Daryani, P. R. Asl and A. Hekmatdoost, Resveratrol supplementation and oxidative/anti-oxidative status in patients with ulcerative colitis: A randomized, double-blind, placebo-controlled pilot study, Arch. Med. Res., 2016, 47, 304–309 CrossRef CAS PubMed.
- E. Candelario-Jalil, A. C. de Oliveira, S. Graf, H. S. Bhatia, M. Hull, E. Munoz and B. L. Fiebich, Resveratrol potently reduces prostaglandin e2 production and free radical formation in lipopolysaccharide-activated primary rat microglia, J. Neuroinflammation, 2007, 4, 25 CrossRef PubMed.
- D. A. Pi, Y. L. Liu, H. F. Shi, S. Li, J. Odle, X. Lin, H. L. Zhu, F. Chen, Y. Q. Hou and W. B. Leng, Dietary supplementation of aspartate enhances intestinal integrity and energy status in weanling piglets after lipopolysaccharide challenge, J. Nutr. Biochem., 2014, 25, 456–462 CrossRef CAS PubMed.
- R. Marcu, Y. Zheng and B. J. Hawkins, Mitochondria and angiogenesis, Adv. Exp. Med. Biol., 2017, 982, 371–406 CrossRef CAS PubMed.
- E. M. Jeong, J. Chung, H. Liu, Y. Go, S. Gladstein, A. Farzaneh-Far, E. D. Lewandowski and S. C. Dudley, Role of mitochondrial oxidative stress in glucose tolerance, insulin resistance, and cardiac diastolic dysfunction, J. Am. Heart Assoc., 2016, 5(5), 173–173 CrossRef PubMed.
- K. Palikaras, E. Lionaki and N. Tavernarakis, Mitophagy: in sickness and in health, Mol. Cell. Oncol., 2016, 3–1 Search PubMed.
- S. Saita, M. Shirane and K. I. Nakayama, Selective escape of proteins from the mitochondria during mitophagy, Nat. Commun., 2013, 4, 1410 CrossRef PubMed.
- M. Z. Springer and K. F. Macleod, In brief: mitophagy: mechanisms and role in human disease, J. Pathol., 2016, 240, 253–255 CrossRef CAS PubMed.
- A. Eiyama and K. Okamoto, Pink1/parkin-mediated mitophagy in mammalian cells, Curr. Opin. Cell Biol., 2015, 33, 95–101 CrossRef CAS PubMed.
- H. Wang, T. Y. Jiang, W. Li, N. Gao and T. Zhang, Resveratrol attenuates oxidative damage through activating mitophagy in an in vitro model of alzheimer's disease, Toxicol. Lett., 2018, 282, 100–108 CrossRef CAS PubMed.
- J. Yin, M. F. Liu, W. K. Ren, J. L. Duan, G. Yang, Y. R. Zhao, R. J. Fang, L. X. Chen, T. J. Li and Y. L. Yin, Effects of dietary supplementation with glutamate and aspartate on diquat-induced oxidative stress in piglets, PLoS One, 2015, 10(4), e0122893 CrossRef PubMed.
- X. B. Mao, M. Lv, B. Yu, J. He, P. Zheng, J. Yu, Q. Y. Wang and D. W. Chen, The effect of dietary tryptophan levels on oxidative stress of liver induced by diquat in weaned piglets, J. Anim. Sci. Biotechnol., 2014, 5, 1–7 CrossRef PubMed.
- S. T. Cao, H. Wu, C. C. Wang, Q. H. Zhang, L. F. Jiao, F. H. Lin and C. H. Hu, Diquat-induced oxidative stress increases intestinal permeability, impairs mitochondrial function, and triggers mitophagy in piglets, J. Anim. Sci., 2018, 96, 1795–1805 CrossRef PubMed.
- S. T. Cao, C. C. Wang, H. Wu, Q. H. Zhang, L. F. Jiao and C. H. Hu, Weaning disrupts intestinal antioxidant status, impairs intestinal barrier and mitochondrial function, and triggers mitophagy in piglets, J. Anim. Sci., 2018, 96, 1073–1083 CrossRef CAS PubMed.
- Y. Zhang, P. Zheng, B. Yu, J. He, J. Yu, X. B. Mao, J. X. Wang, J. Q. Luo, Z. Q. Huang, G. X. Cheng and D. W. Chen, Dietary spray-dried chicken plasma improves intestinal barrier function and modulates immune status in weaning piglets, J. Anim. Sci., 2016, 94, 173–184 CrossRef CAS PubMed.
- C. H. Hu, K. Xiao, Z. S. Luan and J. Song, Early weaning increases intestinal permeability, alters expression of cytokine and tight junction proteins, and activates mitogen-activated protein kinases in pigs, J. Anim. Sci., 2013, 91, 1094–1101 CrossRef CAS PubMed.
- B. L. McLamb, A. J. Gibson, E. L. Overman, C. Stahl and A. J. Moeser, Early weaning stress in pigs impairs innate mucosal immune responses to enterotoxigenic e. Coli challenge and exacerbates intestinal injury and clinical disease, PLoS One, 2013, 8, e59838 CrossRef CAS PubMed.
- K. Xiao, L. F. Jiao, S. T. Cao, Z. H. Song, C. H. Hu and X. Y. Han, Whey protein concentrate enhances intestinal integrity and influences transforming growth factor-beta 1 and mitogen-activated protein kinase signalling pathways in piglets after lipopolysaccharide challenge, Br. J. Nutr., 2016, 115, 984–993 CrossRef CAS PubMed.
- N. Pipatpiboon, W. Pratchayasakul, N. Chattipakorn and S. C. Chattipakorn, Ppar gamma agonist improves neuronal insulin receptor function in hippocampus and brain mitochondria function in rats with insulin resistance induced by long term high-fat diets, Endocrinology, 2012, 153, 329–338 CrossRef CAS PubMed.
- W. J. Li, Y. Chen, S. P. Nie, M. Y. Xie, M. He, S. S. Zhang and K. X. Zhu, Ganoderma atrum polysaccharide induces anti-tumor activity via the mitochondrial apoptotic pathway related to activation of host immune response, J. Cell. Biochem., 2011, 112, 860–871 CrossRef CAS PubMed.
- W. Li, S. Nie, Y. Chen, Y. Wang, C. Li and M. Xie, Enhancement of cyclophosphamide-induced antitumor
effect by a novel polysaccharide from ganoderma atrum in sarcoma 180-bearing mice, J. Agric. Food Chem., 2011, 59, 3707–3716 CrossRef CAS PubMed.
- S. T. Cao, Q. H. Zhang, C. C. Wang, H. Wu, L. F. Jiao, Q. H. Hong and C. H. Hu, LPS challenge increased intestinal permeability, disrupted mitochondrial function and triggered mitophagy of piglets, Innate Immun., 2018, 24(4), 221–230 CrossRef CAS PubMed.
- Q. Huang, W. Xu, K. W. Bai, J. T. He, H. Ahmad, L. Zhou, L. L. Zhang and T. Wang, Protective effects of leucine on redox status and mitochondrial-related gene abundance in the jejunum of intrauterine growth-retarded piglets during early weaning period, Arch. Anim. Nutr., 2017, 71, 93–107 CrossRef CAS PubMed.
- G. Liu, H. Tian, Y. Q. Huang, J. Hu, Y. X. Ji, S. Q. Li, Y. Q. Feng, L. Guo and Y. G. Zhu, Alterations of mitochondrial protein assembly and jasmonic acid biosynthesis pathway in honglian (hl)-type cytoplasmic male sterility rice, J. Biol. Chem., 2012, 287(47), 40051–40060 CrossRef CAS PubMed.
- A. Malhotra, S. Bath and F. Elbarbry, An organ system approach to explore the antioxidative, anti-inflammatory, and cytoprotective actions of resveratrol, Oxid. Med. Cell. Longevity, 2015, 1–15 Search PubMed.
- C. Zhang, J. Q. Luo, B. Yu, P. Zheng, Z. Q. Huang, X. B. Mao, J. He, J. Yu, J. L. Chen and D. W. Chen, Dietary resveratrol supplementation improves meat quality of finishing pigs through changing muscle fiber characteristics and antioxidative status, Meat Sci., 2015, 102, 15–21 CrossRef CAS PubMed.
- N. Jiao, Z. L. Wu, Y. Ji, B. Wang, Z. L. Dai and G. Y. Wu, L-glutamate enhances barrier and antioxidative functions in intestinal porcine epithelial cells, J. Nutr., 2015, 145, 2258–2264 CrossRef CAS PubMed.
- O. Ergun, G. Ergun, G. Oktem, N. Selvi, H. Dogan, M. Tuncyurek, G. Saydam and A. Erdener, Enteral resveratrol supplementation attenuates intestinal epithelial inducible nitric oxide synthase activity and mucosal damage in experimental necrotizing enterocolitis, J. Pediatr. Surg., 2007, 42, 1687–1694 CrossRef PubMed.
- K. Xiao, Z. H. Song, L. F. Jiao, Y. L. Ke and C. H. Hu, Developmental changes of TGF-beta 1 and smads signaling pathway in intestinal adaption of weaned pigs, PLoS One, 2014, 9(8), e104589 CrossRef PubMed.
- H. K. Wei, G. Chen, R. J. Wang and J. Peng, Oregano essential oil decreased susceptibility to oxidative stress-induced dysfunction of intestinal epithelial barrier in rats, J. Funct. Foods, 2015, 18, 1191–1199 CrossRef CAS.
- C. Zhang, X. H. Zhao, L. Yang, X. Y. Chen, R. S. Jiang, S. H. Jin and Z. Y. Geng, Resveratrol alleviates heat stress-induced impairment of intestinal morphology, microflora, and barrier integrity in broilers, Poult. Sci., 2017, 96, 4325–4332 CrossRef CAS PubMed.
- N. Wang, Q. Han, G. Wang, W. P. Ma, J. Wang, W. X. Wu, Y. Guo, L. Liu, X. Y. Jiang, X. L. Xie and H. Q. Jiang, Resveratrol protects oxidative stress-induced intestinal epithelial barrier dysfunction by upregulating heme oxygenase-1 expression, Dig. Dis. Sci., 2016, 61, 2522–2534 CrossRef CAS PubMed.
- S. H. Lee, Intestinal permeability regulation by tight junction: implication on inflammatory bowel diseases, Intest. Res., 2015, 13, 11–18 CrossRef PubMed.
- Y. F. Jiang, S. S. Lin, J. M. Chen, H. Z. Tsai, T. S. Hsieh and C. Y. Fu, Electron tomographic analysis reveals ultrastructural features of mitochondrial cristae architecture which reflect energetic state and aging, Sci. Rep., 2017, 7, 45474 CrossRef CAS PubMed.
- J. R. Friedman and J. Nunnari, Mitochondrial form and function, Nature, 2014, 505, 335–343 CrossRef CAS PubMed.
- H. Zhang, Y. Li, W. P. Su, Z. X. Ying, L. Zhou, L. L. Zhang and T. Wang, Resveratrol attenuates mitochondrial dysfunction in the liver of intrauterine growth retarded suckling piglets by improving mitochondrial biogenesis and redox status, Mol. Nutr. Food Res., 2017, 61, 1600653 CrossRef PubMed.
- G. Benard and R. Rossignol, Ultrastructure of the mitochondrion and its bearing on function and bioenergetics, Antioxid. Redox Signaling, 2008, 10, 1313–1342 CrossRef CAS PubMed.
- E. D. B. Danz, J. Skramsted, N. Henry, J. A. Bennett and R. S. Keller, Resveratrol prevents doxorubicin cardiotoxicity through mitochondrial stabilization and the sirt1 pathway, Free Radical Biol. Med., 2009, 46, 1589–1597 CrossRef PubMed.
- Y. Zhang, X. R. Li, L. Zhao, G. L. Duan, L. Xiao and H. P. Chen, DJ-1 preserving mitochondrial complex I activity plays a critical role in resveratrol-mediated cardioprotection against hypoxia/reoxygenation-induced oxidative stress, Biomed. Pharmacother., 2018, 98, 545–552 CrossRef CAS PubMed.
- H. P. Indo, M. Davidson, H. C. Yen, S. Suenaga, K. Tomita, T. Nishii, M. Higuchi, Y. Koga, T. Ozawa and H. J. Majima, Evidence of ros generation by mitochondria in cells with impaired electron transport chain and mitochondrial DNA damage, Mitochondrion, 2007, 7, 106–118 CrossRef CAS PubMed.
- A. J. Kowaltowski, N. C. de Souza-Pinto, R. F. Castilho and A. E. Vercesi, Mitochondria and reactive oxygen species, Free Radical Biol. Med., 2009, 47, 333–343 CrossRef CAS PubMed.
- M. Lagouge, C. Argmann, Z. Gerhart-Hines, H. Meziane, C. Lerin, F. Daussin, N. Messadeq, J. Milne, P. Lambert, P. Elliott, B. Geny, M. Laakso, P. Puigserver and J. Auwerx, Resveratrol improves mitochondrial function and protects against metabolic disease by activating SIRT1 and PGC-1alpha, Cell, 2006, 127, 1109–1122 CrossRef CAS PubMed.
- F. Diaz, H. Kotarsky, V. Fellman and C. T. Moraes, Mitochondrial disorders caused by mutations in respiratory chain assembly factors, Semin. Fetal Neonatal Med., 2011, 16, 197–204 CrossRef PubMed.
- N. Nissanka and C. T. Moraes, Mitochondrial DNA damage and reactive oxygen species in neurodegenerative disease, FEBS Lett., 2018, 592, 728–742 CrossRef CAS PubMed.
- J. Folbergrová, P. Jesina, H. Kubova and J. Otahal, Effect of resveratrol on oxidative stress and mitochondrial dysfunction in immature brain during epileptogenesis, Mol. Neurobiol., 2018, 55, 7512–7522 CrossRef PubMed.
- I. Kim, S. Rodriguez-Enriquez and J. J. Lemasters, Selective degradation of mitochondria by mitophagy, Arch. Biochem. Biophys., 2007, 462, 245–253 CrossRef CAS PubMed.
- S. M. Yoo and Y. K. Jung, A molecular approach to mitophagy and mitochondrial dynamics, Mol. Cells, 2018, 41, 18–26 CAS.
- J. Wu, X. Li, G. Zhu, Y. Zhang, M. He and J. Zhang, The role of resveratrol-induced mitophagy/autophagy in peritoneal mesothelial cells inflammatory injury via NLRP3 inflammasome activation triggered by mitochondrial ros, Exp. Cell Res., 2016, 341, 42–53 CrossRef CAS PubMed.
|
This journal is © The Royal Society of Chemistry 2019 |
Click here to see how this site uses Cookies. View our privacy policy here.