DOI:
10.1039/C8FO01971A
(Paper)
Food Funct., 2019,
10, 38-48
Immunostimulatory effect of kumquat (Fortunella crassifolia) and its constituents, β-cryptoxanthin and R-limonene†
Received
9th October 2018
, Accepted 22nd November 2018
First published on 23rd November 2018
Abstract
Natural killer (NK) cells play an important role in the innate immune system by eliminating cancer cells and virally infected cells. Aging and stress attenuate the activity of NK cells, thereby increasing the risk of various diseases. In this study, we demonstrated that the consumption of a small number of kumquats in an in vivo model could suppress elevated plasma corticosterone levels and reverse the decline in splenocyte cytotoxicity caused by restraint stress. Our results identified β-cryptoxanthin (BCX) as an active kumquat component with a NK cell-activating effect, and R-limonene as an active component that mediates not only the anti-stress effect but also NK cell activation by oral administration. In addition, BCX, R-limonene, and R-limonene metabolites were found to enhance IFN-γ production in KHYG-1 cells, a human NK cell line. Collectively, our findings suggest that the ingestion of a few kumquats on a daily basis can help to combat stress and enhance NK cell activity.
1. Introduction
Natural killer (NK) cells can eliminate target cells such as tumor cells and virus-infected cells without presensitization. There are several mechanisms by which NK cells can kill their target cells. For example, the inhibitory receptors on NK cells reduce inhibitory signals by sensing a decrease in the expression of major histocompatibility complex class I molecules on target cells. Finally, NK cells kill target cells by releasing cytolytic granules such as perforin and granzyme. In addition, NK cells can attack target cells with the aid of target cell-specific antibodies in a process known as antibody-dependent cell-mediated cytotoxicity.1 Another function employed by NK cells is the production of cytokines such as tumor necrosis factor (TNF)-α and interferon (IFN)-γ. IFN-γ is involved in the activation of NK cells, as well as in their antitumor2 and antiviral effects.3 A decline in NK cell activity has been reported as a cause of elevated cancer4 and infection5 risks. Therefore, NK cell activation is an important process for the maintenance of a healthy life.
The United Nations has reported a rapid progress in aging, as the proportion of the world's population aged 60 years or older is growing at an annual rate of about 3%.6 Many studies have reported that aging is associated with a decrease in the activity of NK cells.7,8 Aging is also considered as a stressor.9 Although stress promotes organism's survival, acute stress has been reported to affect the brain function.10 Moreover, many studies have demonstrated that stress could inhibit NK cell activity.11,12 Stress activates the sympathetic–adrenal–medullary (SAM) and hypothalamic–pituitary–adrenal (HPA) axes,13 which interact with the immune system.14 The activation of the HPA axis leads to the release of glucocorticoids such as cortisol and corticosterone, which are considered to play a role in stress-induced inhibition of NK cell activity. For example, the synthetic glucocorticoid dexamethasone was found to reduce the expression of cytotoxicity-related proteins such as perforin, IFN-γ, and interleukin (IL)-6 and thus decrease the cytotoxicity of NK cells. Accordingly, the reduction of stress, especially by the suppression of glucocorticoid production, seems to be an important approach for maintaining the immune system function.15
Citrus fruits are among the most commonly grown fruits worldwide. Among them, sweet orange (Citrus sinensis) is the most cultivated citrus fruit. In Japan, satsuma mandarin (Citrus unshiu) is the main cultivar of citrus. The fruits of sweet orange and satsuma mandarin are usually eaten raw; however, the peels are hardly eaten raw. Meanwhile, kumquats (Fortunella spp.) are members of the Citrus genus that were introduced to Japan from China. Since the pericarp is sweet and thin and due to the difficulty of peeling, kumquat is usually eaten raw and as the whole fruit including the pericarp in China, Taiwan, and recently in Japan. Kumquat fruits have been known for a long time to serve as a cold remedy and to contain beneficial phytochemicals for health.16 Twenty-five essential oil constituents, including limonene and myrcene, have been identified in kumquats.17 These essential oils have been suggested to possess antimicrobial activities. In addition, 10 flavonoids have been identified in immature kumquats,18 among which 6 flavonoids, including 3′,5′-di-C-β-glucopyranosylphloretin and acacetin 8-C-neohesperidoside, were found to exhibit antioxidant activities. Moreover, kumquats have been reported to contain carotenoids such as lutein, β-carotene, and β-cryptoxanthin (BCX).19 A previous study demonstrated that β-carotene could enhance macrophage phagocytosis and thus be beneficial for immunity.20 Nevertheless, as far as we know, the effect of kumquats on NK cell activity has not yet been investigated. Our previous study demonstrated that kumquat pericarp acetone fraction could enhance NK cell activation in vitro in a human NK cell line (KHYG-1 cells) and in vivo in a mouse model of restraint stress.21 However, the active component was unidentified. Accordingly, in this study, we identified the kumquat constituents that exert NK cell-activating effects. In addition, we reported the anti-stress effects of kumquat and its active components.
2. Materials and methods
2.1. Materials
Acetone, n-hexane, ethanol, acetonitrile, tetrahydrofuran, Na2CO3, Na2SO4, RPMI 1640 medium with and without phenol red, and sevoflurane were purchased from Wako Pure Chemical Industries, Ltd (Osaka, Japan). Calcein-acetoxymethyl (calcein-AM), HEPES, and Cell Counting Kit-8 (CCK-8) were purchased from Dojindo (Kumamoto, Japan). Mineral oil was purchased from MP Biomedicals LLC (Illkirch, France). BCX was purchased from Extrasynthese (ZI Lyon-Nord, Genay, France). R-Limonene, perillic acid (PA), perillyl alcohol (POH), and corticosterone were purchased from Sigma-Aldrich (Saint Louis, USA). Human and mouse IFN-γ ELISA kits and human IL-2 were purchased from PeproTech (Rocky Hill, NJ, USA). An AssayMax Corticosterone ELISA kit was purchased from AssayPro (Charles, MO, USA).
2.2. Cell culture
KHYG-1 cells22 were obtained from the Japanese Collection of Research Bioresources (Osaka, Japan) and cultured in RPMI 1640 medium supplemented with 10% fetal bovine serum (FBS) and 100 U mL−1 IL-2. Human chronic myeloid leukemia cells (K562)23 and mouse lymphoma cells (Yac-1)24 were cultured in RPMI 1640 medium supplemented with 10% FBS. All cells were maintained in a humidified environment containing 5% CO2 at 37 °C.
2.3. Preparation of lyophilized kumquat powder
The kumquats (Fortunella crassifolia) used in the experiment adhered to the following specifications: a diameter of 3.3–3.7 cm, 16 degrees Brix, and more than 210 days from flowering and fruiting. Seeds and calyxes were removed, pasted using a mixer, and lyophilized. One kumquat was equivalent to 3.70 g of lyophilized kumquat powder (K-FD).
2.4. High performance liquid chromatography analysis of the n-hexane extract of K-FD
K-FD (5.0 g) was mixed with 10 mL of acetone and filtered through filter paper, and the filtrate was saved. This process was repeated 5 times. The filtrate was extracted twice with n-hexane
:
distilled water (1
:
1.7), and the organic layer was concentrated using a vacuum evaporator, while the residue was dissolved in n-hexane, added to 20% KOH/methanol, and saponified at 60 °C for 30 min. The saponified mixture was extracted twice with n-hexane
:
distilled water (1
:
1), and the organic layer was concentrated using a vacuum evaporator. The residue was dissolved in 4 mL of acetonitrile
:
ethanol
:
tetrahydrofuran (69
:
30
:
1) and filtered through a hydrophilic PTFE filter. High performance liquid chromatography (HPLC) was performed using LC-20AD vp pumps, an SPD-M20A photodiode array detector (Shimadzu, Kyoto, Japan), and an STR ODS-II column (4.6 mm i.d. × 250 mm; 5 μm particle diameter; 12 nm pore diameter, Shinwa Chemical Industries Ltd, Kyoto, Japan). The mobile phase consisted of acetonitrile
:
ethanol
:
tetrahydrofuran (69
:
30
:
1). The analysis was carried out under isocratic conditions at a flow rate of 1.0 mL min−1, the column temperature was maintained at 30 °C, and detection was performed at an absorbance wavelength of 450 nm. The standard curve was constructed using 5–100 ng of BCX.
2.5. Gas chromatography–mass spectrometry analysis of the n-hexane extract of K-FD
K-FD (1.5 g) was suspended in 3 mL of n-hexane. The suspension was stirred at 250 rpm for 30 min and then centrifuged at 1000g for 1 min. The supernatant was collected in a new test tube. The extraction process was repeated 3 times. Gas chromatography–mass spectrometry (GC-MS) analysis of the n-hexane extract was performed using a GC-2010 gas chromatograph coupled to a GCMS-QP2010 mass spectrometer (Shimadzu, Kyoto, Japan) equipped with a DB-5 capillary column (0.25 mm i.d. × 30 m; 0.25 μm film thickness, Agilent Technologies, Santa Clara, USA). The GC-MS analysis was carried out under the following conditions: injector temperature 220 °C; helium gas flow rate 0.92 mL min−1; injection volume 1 μL; split ratio 1
:
20; oven temperature programmed to rise from 60 °C to 250 °C at 3 °C min−1; electron impact ionization voltage 70 eV; interface temperature 250 °C; ion source temperature 200 °C; mass range 40–350 m/z. The identities of the components were confirmed by comparing the obtained mass spectral data with the data from the literature.25
2.6. Animals
Male 7-week-old C57BL/6N mice were purchased from Japan SLC Inc. (Shizuoka, Japan). Mice were acclimatized for 1 week prior to the experiment. All mice were housed under controlled conditions of temperature at 24 ± 1 °C and a 14 h light/10 h dark cycle (lights on from 05:00 to 19:00), and were fed CE-2 and water ad libitum. Mice were treated according to the Animal Experiment Committee of Miyazaki University, and the experimental protocol was approved by the ethics committee of Miyazaki University (permit number 2016-034, 2016-034-2, and 2016-034-4).
2.7. K-FD feeding test
The composition of the standard rodent diet AIN-7626 was partially modified to enhance the effect of K-FD consumption. The modified AIN-76 composition is shown in Table 1. Mice were divided into 3 groups: control, model, and 0.13% K-FD groups. The control and model groups were fed modified AIN-76 without K-FD, while the 0.13% K-FD group was fed modified AIN-76 containing 0.13% K-FD, which was equivalent to the consumption of 3 kumquats per day by a 60 kg human. After being acclimatized for 1 week, mice were fed their respective diets for 7 consecutive days ad libitum. On the 8th day, the model and 0.13% K-FD groups were restrained for 4 h (9:00–13:00) in a restraint cage. The restraint cage combined a 50 mL polypropylene centrifuge tube with a 50 mL syringe (Fig. s1†).
Table 1 Modified AIN-76 composition
Components |
AIN-76 |
Modified AIN-76 |
Control and model groups |
0.13% K-FD |
Casein |
20% |
20% |
20% |
Sucrose |
50% |
35% |
34.87% |
β-Cornstarch |
15% |
15% |
15% |
Corn oil |
5% |
20% |
20% |
Cellulose |
5% |
5% |
5% |
Mineral mixture |
3.5% |
3.5% |
3.5% |
Vitamin mixture |
1% |
1% |
1% |
DL-Methionine |
0.3% |
0.3% |
0.3% |
Choline bitartrate |
0.2% |
0.2% |
0.2% |
K-FD |
— |
— |
0.13% |
|
Total |
100% |
100% |
100% |
2.8.
R-Limonene and BCX oral administration
Mice were divided into 5 groups: control, model, low-dose R-limonene-administered (administered R-limonene at a dose of 28.3 μg kg−1 for 1 week), high-dose R-limonene-administered (administered R-limonene at a dose of 94.3 μg kg−1 for 1 week), and BCX-administered (administered BCX at a dose of 1.50 μg kg−1 for 1 week) groups. The control and model groups were administered mineral oil, while BCX and R-limonene-administered groups were administered BCX and R-limonene, respectively, dissolved in mineral oil. Treatments were administered orally using disposable feeding needles (Fuchigami, Kyoto, Japan) for 1 week and again 3 h before restraint stress. On the 8th day, model, R-limonene-administered, and BCX-administered groups were restrained for 4 h (9:00–13:00) in a restraint cage.
2.9. Measurement of plasma corticosterone levels
After restraint, blood samples were collected from the tail vein into a heparin-coated capillary tube (Drummond Scientific, Broomall, PA, USA). Plasma was isolated by centrifugation at 1500g for 5 min at 4 °C. Plasma corticosterone levels were measured using an AssayMax Corticosterone ELISA kit according to the manufacturer's instructions.
2.10. Measurement of plasma IFN-γ levels
Mice were anesthetized with sevoflurane and dissected. Blood samples were collected from the heart using a heparin-coated syringe. Plasma was isolated by centrifugation at 1500g for 5 min at 4 °C, and plasma samples were stored at −80 °C until measurement was done. Plasma IFN-γ levels were measured using a standard ABTS murine IFN-γ ELISA kit according to the manufacturer's instructions.
2.11. Spleen lymphocyte preparation
Spleens were harvested, mashed with frosted glass slides, and washed with cold RPMI 1640 containing 10 mM HEPES. Splenocytes were centrifuged at 400g for 5 min, hemolyzed with 1 mL of a hemolytic agent (14 mM NH4Cl/17 mM Tris-HCl, pH = 7.5) for 5 min, and washed twice with RPMI 1640 containing 10 mM HEPES. Then, cells were resuspended in cold RPMI 1640 without phenol red supplemented with 10% FBS. The total number of spleen lymphocytes was determined using a hemocytometer.
2.12. Cytotoxicity assay
Cytotoxicity was evaluated using the calcein-AM release assay as previously described.21 Target K562 or Yac-1 cells were washed with PBS, adjusted to 1 × 106 cells per mL, and labeled with 10 μg mL−1 calcein-AM solution for 0.5 or 1 h, respectively.
The in vitro cytotoxicity assay involved determining the cytotoxicity of KHYG-1 cells (effector NK cells) against K562 cells (target cells). KHYG-1 cells (2 × 105 cells per mL) were treated with BCX, R-limonene, R-limonene metabolites, or vehicle control (dimethyl sulfoxide or ethanol). After 24 h, retrieved cells were washed with PBS, resuspended in RPMI 1640 medium without phenol red, and supplemented with 1% bovine serum albumin (in vitro assay medium). KHYG-1 cells (1 × 105 cells per well) and calcein-AM-labeled K562 cells (1 × 104 cells per well) were co-cultured at an effector-to-target cell (E
:
T) ratio of 10
:
1 in 96-well U-bottomed plates, and incubated at 37 °C for 4 h. Maximum release was obtained by incubation of target cells only in in vitro assay medium with 2% Triton X-100. Spontaneous release was obtained by incubation of target cells only in in vitro assay medium. After incubation, plates were centrifuged at 1200g for 10 min, and the supernatants (100 μL) were transferred to a new plate. Fluorescence was measured with a Varioskan Flash instrument (excitation filter 490 nm, emission filter 515 nm; Thermo Fisher Scientific, Waltham, MA, USA). The percentage of specific lysis was calculated as follows: Cytotoxicity (%) = [(experimental calcein-AM release − spontaneous calcein-AM release)/(maximum calcein-AM release − spontaneous calcein-AM release)] × 100.
The in vivo cytotoxicity assay involved determining the cytotoxicity of mouse splenocytes (effector cells) against Yac-1 cells (target cells). Mouse splenocytes and calcein-AM-labeled Yac-1 cells were co-cultured at various E
:
T ratios (100
:
1, 50
:
1, 25
:
1, and 12.5
:
1) in 96 well U-bottomed plates for 4 h at 37 °C. After 4 h, plates were centrifuged at 1200g for 10 min. The percentage of specific lysis was calculated as described above. Specific lysis values obtained from the calcein-AM release assay were converted to lytic units (LU) according to a previously reported method.27 LU is defined as the number of cells required to cause 10% target cell lysis relative to 107 effector cells, as determined by the equation:
where

is the mean of the logistically transformed specific lysis values,
p is the reference lysis percentage used in defining the lytic unit (in this case,
p = 10%),
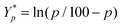
,
C is a constant set to 1,
T is the number of target Yac-1 cells, and

is the geometric mean of the E
![[thin space (1/6-em)]](https://www.rsc.org/images/entities/char_2009.gif)
:
![[thin space (1/6-em)]](https://www.rsc.org/images/entities/char_2009.gif)
T ratio used in the assay.
2.13. Cell viability
The cell viability of KHYG-1 cells was measured using CCK-8. KHYG-1 cells were seeded into 96-well plates at a density of 2 × 104 cells per well and treated with experimental compounds or a vehicle control for 24 h in a humidified environment containing 5% CO2 at 37 °C. After 24 h, 100 μL of CCK-8 diluted 10-fold with RPMI 1640 was added to all wells and plates were incubated for 2 h. The number of viable cells was determined by measuring the absorbance at 450 nm according to the manufacturer's instructions. The percentage of cell viability was calculated as follows: Cell viability (%) = [absorbance of the experimental group/absorbance of the control group] × 100.
2.14. Measurement of IFN-γ levels in the culture supernatant
KHYG-1 cells were seeded in a 48-well plate at a density of 1.2 × 105 cells per well and treated with experimental compounds or vehicle control for 24 h in a humidified environment containing 5% CO2 at 37 °C. After 24 h, cells were collected in 1.5 mL tubes and centrifuged at 1000g for 5 min to remove the cells. IFN-γ levels in the culture supernatants were measured using a standard ABTS human IFN-γ ELISA kit according to the manufacturer's instructions.
2.15. Statistical analysis
All results were expressed as the mean ± standard error (SE) or the mean ± standard deviation (SD). Multiple comparisons were performed using one-way ANOVA followed by Dunnett's test or one-way ANOVA followed by Tukey–Kramer's test. Statistical differences between the 2 groups were analyzed by Student's t-test or the Mann–Whitney U-test. All statistical analyses were carried out using GraphPad Prism version 7.04 (GraphPad Software, San Diego, CA, USA).
3. Results
3.1. Effect of K-FD on NK cell activation in vivo
Our previous study has shown that kumquat pericarp extract exerts NK cell-activating effects, but no anti-stress effects.21 However, it did not clarify the effect of the whole fruit. Therefore, in this study, we investigated the NK cell-activating and anti-stress effects of whole kumquat fruit in vivo. There was no significant difference in the amounts of food intake and body weight gain between the control and 0.13% K-FD groups over 1 week (data not shown). As an index of acute stress, plasma corticosterone levels were determined by ELISA. Plasma corticosterone levels in the model group were approximately 12.1 fold higher than in the control group. On the other hand, they were significantly reduced in the 0.13% K-FD group compared to the model group (Fig. 1A). These results suggest that the intake of K-FD could suppress restraint stress-induced elevation in plasma corticosterone levels. We also evaluated the cytotoxic activity of splenocytes using the calcein-AM release assay. The cytotoxic activity in the model group was significantly reduced compared to the control group. However, K-FD intake significantly increased the cytotoxic activity of the splenocytes compared to the model group (Fig. 1B). These results suggest that K-FD, namely whole kumquat fruit, exerts an anti-stress effect in addition to its NK cell-activating effect.
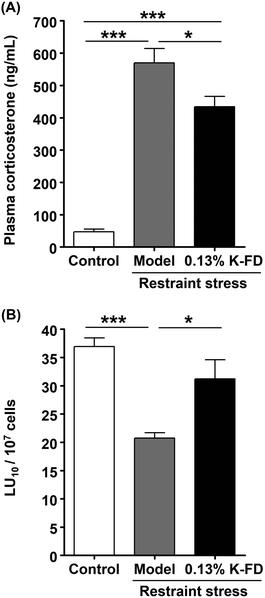 |
| Fig. 1 Effect of K-FD on plasma corticosterone levels and NK cell cytotoxicity in mice exposed to restraint stress. The control and model groups were fed modified AIN-76 and the 0.13% K-FD group was fed modified AIN-76 containing 0.13% lyophilized kumquat powder (K-FD) for 7 days. (A) Plasma corticosterone levels were determined by quantitative competitive ELISA. (B) NK cytotoxic activity per splenocyte (LU10 per 107 cells). In the determination of NK cell cytotoxic activity, calcein-AM-labeled Yac-1 (target) cells were mixed with spleen lymphocytes and incubated for 4 h. After incubation, NK cell activity was determined using the calcein-AM release assay. Results are expressed as the mean ± SE (n = 5). Multiple comparisons were performed using one-way ANOVA followed by Tukey–Kramer's post hoc test. *P < 0.05, ***P < 0.001. | |
3.2. Effect of BCX on NK cell activation in vivo
Results of our previous study have suggested that the main components of kumquat responsible for NK cell activation are carotenoids, especially BCX. However, the effect of BCX was not confirmed in vivo. Accordingly, in this study, we examined the NK cell-activating and anti-stress effects of BCX in vivo. First, the amount of BCX in K-FD was determined by HPLC. Results of the analysis showed that 1.00 g of K-FD contained 8.34 ± 1.82 μg of BCX. The daily intake of BCX was calculated from the data on food intake obtained from the K-FD feeding test, and an equivalent amount of BCX was administered orally to the BCX-administered group. Plasma corticosterone levels in the model and BCX-administered groups were significantly higher than those in the control group. However, the corticosterone level in the BCX-administered group tended to decrease compared to the model group, but this decrease was not statistically significant (Fig. 2A). Moreover, the cytotoxic activity in the model group was significantly reduced compared to the control group. Meanwhile, BCX administration reversed the restraint stress-induced decline in the cytotoxic activity (Fig. 2B). These results suggest that BCX exerts a NK cell-activating effect, but not an anti-stress effect, in vivo.
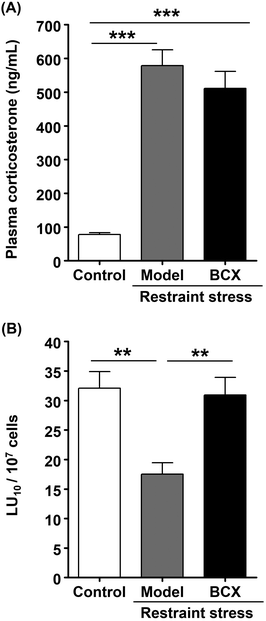 |
| Fig. 2 Effect of BCX on plasma corticosterone levels and NK cell cytotoxicity in mice exposed to restraint stress. The control and model groups were administered mineral oil, while β-cryptoxanthin (BCX)-administered groups were administered BCX at a dose of 1.50 μg kg−1 for 1 week and again 3 h before restraint stress. (A) Plasma corticosterone levels were determined by quantitative competitive ELISA. (B) NK cytotoxic activity per splenocyte (LU10 per 107 cells). In the determination of NK cell cytotoxic activity, calcein-AM-labeled Yac-1 (target) cells were mixed with spleen lymphocytes and incubated for 4 h. After incubation, NK cell activity was determined using the calcein-AM release assay. Results are expressed as the mean ± SE (n = 5 or 6). Multiple comparisons were performed using one-way ANOVA followed by Tukey–Kramer's post hoc test. **P < 0.01, ***P < 0.001. | |
3.3. Effect of BCX on NK cell activation in vitro
In order to determine whether the BCX-induced increase in the cytotoxicity of murine splenocytes (Fig. 2B) is directly involved in NK cell activation, we investigated the NK cell-activating effect of BCX in a human NK cell line (KHYG-1). As indices of NK cell activity, we evaluated IFN-γ production and the cytotoxic activity in BCX-treated KHYG-1 cells. IFN-γ production was enhanced by treatment with BCX concentrations higher than 0.5 μM (Fig. 3A). Nevertheless, the concentration with the maximum effect could not be determined because concentrations higher than 1.0 μM caused BCX precipitation in the culture medium. The cytotoxicity assays were performed using KHYG-1 cells as effector cells and K562 cells as target cells. The cytotoxic activity of BCX (1.0 μM)-treated KHYG-1 cells was significantly higher than that of vehicle control-treated cells (Fig. 3B). These results imply that BCX exerts its NK cell-activating effect by acting directly on NK cells.
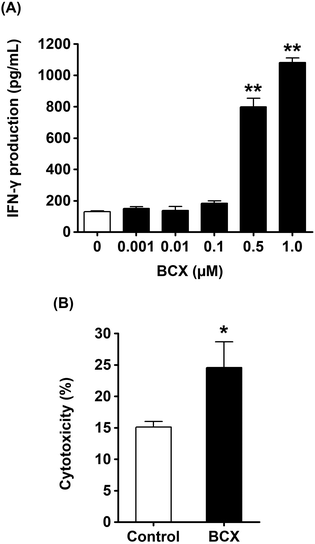 |
| Fig. 3 Effect of BCX on NK cell activation in a human NK cell line. KHYG-1 cells were treated with the indicated concentrations of β-cryptoxanthin (BCX) for 24 h. (A) IFN-γ levels in the culture supernatant were determined by ELISA. (B) Cytotoxic activity of NK cells treated with BCX (1.0 μM). Results are expressed as the mean ± SD (n = 3 or 4). Statistically significant differences were determined by the Mann–Whitney U-test (B) or one-way ANOVA followed by Dunnett's test for individual comparison of groups with 0 μM group (A). *P < 0.05, **P < 0.01. | |
3.4. Effect of R-limonene on NK cell activation and stress in vivo
Among the essential oils contained in citrus, R-limonene has been reported to exert physiological and behavioral anti-stress effects.28R-Limonene accounts for approximately 70% of the essential oils in kumquat.17 Therefore, we investigated the anti-stress effect of R-limonene in vivo. First, the dosage of R-limonene was calculated by determining the amount of R-limonene in K-FD. According to the GC-MS analysis, 1.00 g of K-FD contained 144.4 ± 7.1 μg of R-limonene. R-Limonene was administered to mice in a low dose (28.3 μg kg−1; equivalent to 3.2 kumquats per 60 kg b.w. per day) and a high dose (94.3 μg kg−1; equivalent to 10.5 kumquats per 60 kg b.w. per day) for 1 week. Then, plasma corticosterone levels and the cytotoxic activity of splenocytes were determined. The plasma corticosterone level in the model group was significantly higher than that in the control group. However, the oral administration of R-limonene significantly reduced the restraint stress-induced elevation in plasma corticosterone levels (Fig. 4A), which suggests that the anti-stress effect of K-FD (Fig. 1A) was mainly mediated by R-limonene. Since stress is reported to affect NK cell activation, we investigated the effect of corticosterone on IFN-γ production and found that corticosterone decreased IFN-γ production in a dose-dependent manner in vitro (Fig. 4B). These results imply that the R-limonene-mediated decline in corticosterone levels could reverse the restraint stress-induced decrease in NK cell activity. As shown in Fig. 4C, the cytotoxic activity in the low-dose R-limonene-administered group tended to increase compared to the model group. Meanwhile, administration of high-dose R-limonene significantly improved the cytotoxic activity compared to the model group. These results strongly suggest that R-limonene possesses a NK cell-activating effect.
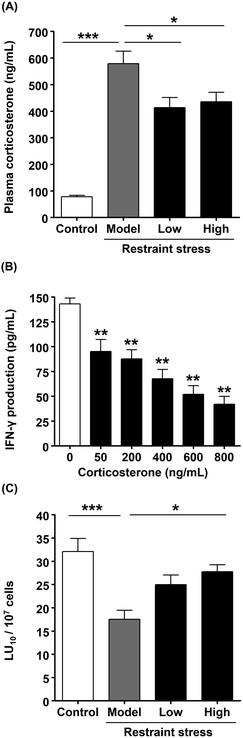 |
| Fig. 4 Effect of R-limonene on plasma corticosterone levels and NK cell cytotoxicity in mice exposed to restraint stress. The control and model groups were administered mineral oil, while low-dose administered groups were administered R-limonene at a dose of 28.3 μg kg−1 and high-dose administered groups were administered R-limonene at a dose of 94.3 μg kg−1 for 1 week and again 3 h before restraint stress. (A) Plasma corticosterone levels were determined by quantitative competitive ELISA. (B) KHYG-1 cells were treated with the indicated concentrations of corticosterone for 24 h. IFN-γ levels in the culture supernatant were determined by ELISA. (C) NK cytotoxic activity per splenocyte (LU10 per 107 cells). In the determination of NK cell cytotoxic activity, calcein-AM-labeled Yac-1 (target) cells were mixed with spleen lymphocytes and incubated for 4 h. After incubation, NK cell activity was determined using a calcein-AM release assay. Plasma corticosterone levels and NK cytotoxic activity are expressed as the mean ± SE (n = 5 or 6). IFN-γ levels are expressed as the mean ± SD (n = 3). Statistically significant differences were determined by one-way ANOVA followed by the Tukey–Kramer's post hoc test (A and C) or Dunnett's test for individual comparison of groups with 0 μM group (B). *P < 0.05, **P < 0.01, ***P < 0.001. | |
3.5. The direct effect of R-limonene and its metabolites on NK cell activation in vitro
Our previous results demonstrated that administration of R-limonene enhanced the NK cell activity in vivo, but it remained unclear whether this effect was a direct or indirect one. Therefore, we examined the direct effect of R-limonene on NK cell activation by treating KHYG-1 cells with R-limonene (0–100 μM) for 24 h and measuring IFN-γ levels in the culture supernatant by ELISA. As shown in Fig. 5Ai, treatment with 10 and 100 μM of R-limonene significantly increased IFN-γ production compared to control (0 μM) cells. Besides, R-limonene did not affect cell viability in KHYG-1 cells (Fig. 5Bi). These results suggest that R-limonene acts directly by enhancing the production of IFN-γ in NK cells. However, it was reported that R-limonene is immediately metabolized and rarely observed in the blood after its absorption.29 Therefore, we examined the NK cell-activating effects of PA, which is one of the metabolites of R-limonene, and the intermediate metabolite POH. Both PA at 1–100 μM and POH at 0.1–100 μM significantly increased IFN-γ production compared to control cells (Fig. 5Aii, iii) without affecting cell viabilities (Fig. 5Bii, iii). Next, the cytotoxic activities of KHYG-1 cells treated with the different compounds were evaluated. There was no significant difference between the control and R-limonene-treated cells, while treatment with PA and POH significantly increased the cytotoxic activity (Fig. 5C). These results suggest that although R-limonene partially enhances the NK cell activity, PA and POH exert a stronger NK cell-activating effect than that of R-limonene. To the best of our knowledge, this is a novel discovery.
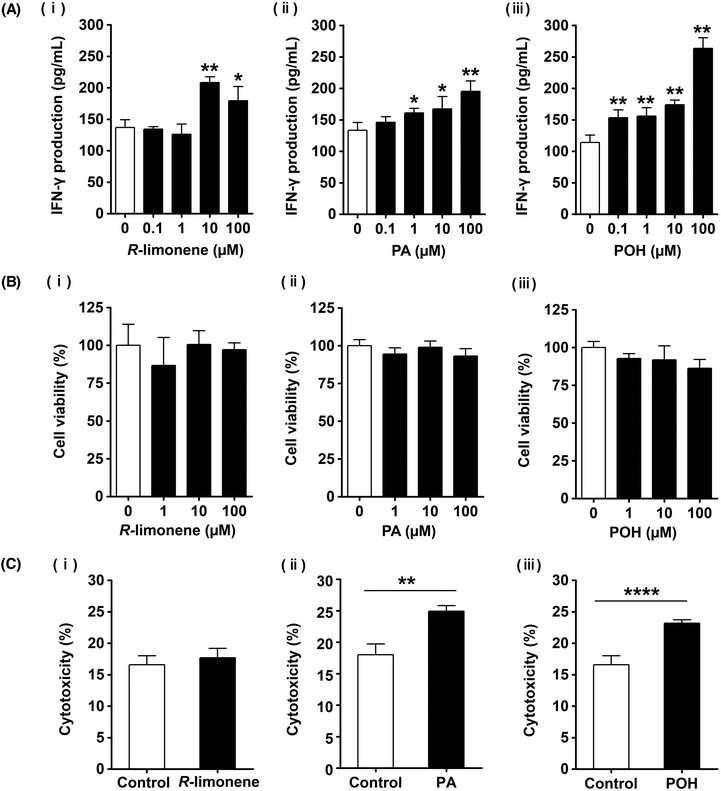 |
| Fig. 5 Effect of R-limonene, PA, and POH on NK cell activation. KHYG-1 cells were treated with the indicated concentrations of R-limonene, perillic acid (PA), or perillyl alcohol (POH) for 24 h. (A) IFN-γ levels in the culture supernatant were determined by ELISA. (B) Cell viability was determined using a Cell-Counting Kit-8. (C) NK cytotoxic activity by R-limonene (10 μM), PA (100 μM), or POH (100 μM). IFN-γ levels, cell viability, and cytotoxicity are expressed as the mean ± SD (n = 3 or 4). Statistically significant differences were determined by Student's t-test (C) or one-way ANOVA followed by Dunnett's test for individual comparison of groups with 0 μM group (A and B). *P < 0.05, **P < 0.01, ****P < 0.0001. | |
4. Discussion
In this study, we evaluated the NK cell-activating and anti-stress effects of whole kumquat fruit and its constituents, BCX and R-limonene, in vivo. We also investigated the NK cell-activating effects of BCX and R-limonene in vitro using a human NK cell line. Consumption of whole kumquat fruit in an in vivo model was found to reverse restraint stress-induced decline in cytotoxicity of murine splenocytes and elevation in plasma corticosterone levels. These results demonstrated that whole kumquat fruits exert NK cell-activating and anti-stress effects (Fig. 1). Results of our previous study have strongly suggested that BCX is the main active component of kumquat responsible for NK cell activation.21 Therefore, we investigated the effect of oral administration of BCX to mice and found that it reversed the restraint stress-induced decline in cytotoxicity of murine splenocytes. However, BCX oral administration did not suppress the restraint stress-induced elevation in plasma corticosterone levels (Fig. 2). These results demonstrated that BCX exerts a NK cell-activating effect, but not an anti-stress effect, in vivo. In addition, BCX was found to directly enhance NK cell activation by increasing cytotoxicity and IFN-γ production in vitro (Fig. 3). Then, we investigated the effect of oral administration of R-limonene to mice, as we hypothesized that the anti-stress effect of kumquat is attributed to the presence of R-limonene. R-Limonene was found to not only suppress the elevated plasma corticosterone levels, but also reverse the decline in cytotoxicity of splenocytes caused by restraint stress (Fig. 4). It can be estimated from these results that the anti-stress effect of R-limonene is mediated by lowering the plasma corticosterone levels and subsequently suppressing partially the decline in NK cell activity. However, considering the possibility that R-limonene could act directly on NK cells, we investigated the NK cell-activating effect of R-limonene, and its metabolites PA and POH, in vitro. Both R-limonene and its 2 metabolites were found to enhance the production of IFN-γ. Moreover, PA and POH enhanced the cytotoxic activity of treated cells and were demonstrated to enhance NK cell activation (Fig. 5). To the best of our knowledge, the NK cell-activating effect of the kumquat constituents BCX and R-limonene is a novel finding of this study.
Meanwhile, β-carotene, a BCX analog, has been reported to demonstrate NK cell-activating effects in vivo30 and in clinical studies.31 However, to the best of our knowledge, the mechanism of this effect has not yet been clarified. The activation of NK cells results in the lysis of target cells and the production of inflammatory cytokines.32 NK cells exert their cytotoxicity by degranulation through the activation of mitogen-activated protein kinase (MAPK) via natural cytotoxic receptors, natural-killer receptor group 2 member D (NKG2D), or TNF receptor superfamily member 9 (CD137).33 Moreover, NKG2D and CD137 regulate the activation of nuclear factor-kappa B and MAPK through adhesion and degranulation-promoting adapter protein-Carma1-Bcl10-Malt1 signalosome, which induces the production of cytokines such as IFN-γ and granulocyte-macrophage colony stimulating factor.32,33 In addition, several signaling pathways triggered by IL-12 and IL-18 finally lead to IFN-γ secretion independently of degranulation.33 Although it is unclear whether BCX was directly involved in these signaling pathways, the stimulatory effect of BCX on osteoblastic cell components seems to be partly mediated through MAPK signaling.34 We postulated that the same signaling pathway might be involved in the NK cell-activating effect of BCX. Further research is needed to elucidate this mechanism. Although BCX tended to exert an anti-stress effect, there was no significant difference between BCX-administered and model groups in plasma corticosterone levels (Fig. 2A). In a previous study, salivary α-amylase activity (sAA) was measured as an index of the SAM axis in placebo and BCX-treated humans, and BCX treatment was found to exert an anti-stress effect in females, but not in males.35 These results suggested a possible influence of gender difference on the anti-stress effect of BCX. However, we could not investigate this in our study as only male mice were used. Some studies have reported a correlation between sAA and salivary cortisol36 while others have reported no correlation.37 In this study, we measured plasma corticosterone levels as an index of the HPA axis. However, the anti-stress effect of BCX can be confirmed by measuring other additional stress indices.
There are different physical and psychological types of stress that can be induced and investigated in experimental animals. Plasma corticosterone levels in mice with physical stress, such as cold or foot shock stress, are approximately 600 ng mL−1, while in mice with psychological stress using a communication box, plasma corticosterone levels are approximately 500 ng mL−1.38 This indicates that physical stress seems to be stronger than psychological stress. Restraint stress is classified as physical stress, and we found that plasma corticosterone levels after 4 h of restraint stress were approximately 600 ng mL−1. Many previous studies have confirmed the anti-stress effect of limonene at high doses such as 50 mg kg−1 administered orally for 1 week,39 a single oral administration of 10 mg kg−1,28 or a single intraperitoneal injection of 1 mg kg−1.38 It seems that limonene was regarded as a medicine in these reports. However, interestingly, we demonstrated in this study that R-limonene can exert an anti-stress effect at low doses such as 28.3 μg kg−1, which indicates that a small amount of R-limonene is sufficient to exert an anti-stress effect.
Moreover, D-limonene has been suggested to positively regulate the immune response. For example, it was found to enhance phagocytosis and stimulate nitric oxide production in peritoneal macrophages obtained from mice with lymphoma.40 In contrast, high concentration of D-limonene was reported to negatively affect IFN-γ production in T cells isolated from mice splenocytes.41 However, our results confirmed that R-limonene, at a low concentration, could enhance IFN-γ production in a human NK cell line. These findings raise the possibility that the effect of R-limonene on immunity is strongly dependent on its concentration. Meanwhile, PA and POH successfully enhanced the cytotoxic activity, while R-limonene did not. However, the underlying mechanism for this difference remains unclear and further research is needed to elucidate it. On the other hand, limonene-1,2-diol, limonene-8,9-diol, and cis or trans-carveol are known to be the metabolites of R-limonene other than PA and POH.29 Among them, limonene-1,2-diol was reported to have an anti-inflammatory effect.41 However, as far as we know, the effect of these compounds on NK cell activity has not yet been reported, and it is a future problem to investigate.
The effect of kumquats on NK cell activation is highly influenced by the intake manner. In a previous study, mice were orally administered kumquat acetone fraction dissolved in mineral oil or PBS. However, cytotoxicity was found to increase in the mineral oil group, but not in the PBS group (data not shown). This result indicates the involvement of oil in carotenoid absorption.42 Therefore, it seems to be necessary to consume kumquat simultaneously with oil in order to exert its NK cell-activating effect. Moreover, this study indicates that the consumption of small amounts of kumquat exerts NK-cell activating and anti-stress effects, as these effects were observed by the consumption of an amount equivalent to 3 kumquats per day (in other words, 1 kumquat per meal) by a human whose body weight is 60 kg. Hence, the consumption of kumquat in practical quantities could promote health and help maintain a healthy lifestyle.
5. Conclusions
In this study, we demonstrated a novel effect of kumquat and its constituents, BCX and R-limonene, on NK cell activation both in vivo and in vitro. R-Limonene metabolites, PA and POH, were also found to exhibit the same effect. Since this effect was observed with the consumption of a small number of kumquats that can be ingested on a daily basis, this research highlights the potential benefits of kumquats and its constituents to human health.
Author contributions
N. Eto, A. Murata, and R. Terao designed most of the study. A. Murata and R. Terao performed all the experiments. K. Sugamoto designed and supervised experiments to quantify R-limonene. N. Murakami and K. Nakahara designed and supervised the in vivo experiments. T. Watanabe, T. Kondo, and K. Fukui prepared the lyophilized kumquat powder. R. Terao and N. Eto prepared the figures and wrote the manuscript using information obtained from other co-authors. All authors participated in reviewing and editing the manuscript. N. Eto directed and supervised all aspects of the study in collaboration with K. Nagahama and H. Hattori.
Conflicts of interest
There are no conflicts to declare.
Acknowledgements
We thank Dr Masanobu Sakono and Dr Hiroyuki Sakakibara for their helpful discussions. We also thank Ms Asami Matsuda and Mr Noriyuki Uegaki for their excellent assistance in animal experiments. We thank Editage (http://www.editage.jp) for English language editing. This work was financially supported by the Research Funding from the Faculty of Agriculture, University of Miyazaki.
References
- H. H. Lee, H. Kang and H. Cho, Natural killer cells and tumor metastasis, Arch. Pharmacal Res., 2017, 40, 1037–1049 CrossRef PubMed.
- M. R. Zaidi and G. Merlino, The two faces of interferon-γ in cancer, Clin. Cancer Res., 2011, 17, 6118–6124 CrossRef PubMed.
- N. Sumaria, S. L. van Dommelen, C. E. Andoniou, M. J. Smyth, A. A. Scalzo and M. A. Degli-Esposti, The roles of interferon-gamma and perforin in antiviral immunity in mice that differ in genetically determined NK-cell-mediated antiviral activity, Immunol. Cell Biol., 2009, 87, 559–566 CrossRef PubMed.
- P. Piątkiewicz, T. Miłek, M. Bernat-Karpińska, M. Ohams, A. Czech and P. Ciostek, The dysfunction of NK cells in patients with type 2 diabetes and colon cancer, Arch. Immunol. Ther. Exp., 2013, 61, 245–253 CrossRef.
- D. L. Evans, T. R. Ten Have, S. D. Douglas, D. R. Gettes, M. Morrison, M. S. Chiappini, P. Brinker-Spence, C. Job, D. E. Mercer, Y. L. Wang, D. Cruess, B. Dube, E. A. Dalen, T. Brown, R. Bauer and J. M. Petitto, Association of depression with viral load, CD8 T lymphocytes, and natural killer cells in women with HIV infection, Am. J. Psychiatry, 2002, 159, 1752–1759 CrossRef.
- United Nations, Department of Economic and Social Affairs and Population Division, World Population Prospects: The 2017 Revision, Key Findings and Advance Tables, 2017, Working Paper No. ESA/P/WP/248 Search PubMed.
- J. Hazeldine, P. Hampson and J. M. Lord, Reduced release and binding of perforin at the immunological synapse underlies the age-related decline in natural killer cell cytotoxicity, Aging Cell, 2012, 11, 751–759 CrossRef.
- J. Hazeldine and J. M. Lord, The impact of ageing on natural killer cell function and potential consequences for health in older adults, Ageing Res. Rev., 2013, 12, 1069–1078 CrossRef.
- K. Hargis, H. M. Buechel, J. Popovic and E. M. Blalock, Acute psychosocial stress in mid-aged male rats causes hyperthermia, cognitive decline, and increased deep sleep power, but does not alter deep sleep duration, Neurobiol. Aging, 2018, 70, 78–85 CrossRef.
- B. S. McEwen, C. R. McKittrick, K. L. Tamashiro and R. R. Sakai, The brain on stress: Insight from studies using the Visible Burrow System, Physiol. Behav., 2015, 146, 47–56 CrossRef.
- Z. Ma, Y. Liu, X. Zhou, H. L. Yu, M. Q. Li, C. Tomiyama-Miyaji, T. Abo and X. F. Bai, Research on stress-induced apoptosis of natural killer cells and the alteration of their killing activity in mouse liver, World J. Gastroenterol., 2013, 19, 6258–6264 CrossRef.
- J. K. Kiecolt-Glaser, R. Glaser, E. C. Strain, J. C. Stout, K. L. Tarr, J. E. Holliday and C. E. Speicher, Modulation of cellular immunity in medical students, J. Behav. Med., 1986, 9, 5–21 CrossRef.
- L. D. Godoy, M. T. Rossignoli, P. Delfino-Pereira, N. Garcia-Cairasco and E. H. de Lima Umeoka, A Comprehensive Overview on Stress Neurobiology: Basic Concepts and Clinical Implications, Front. Behav. Neurosci., 2018, 12, 127 CrossRef PubMed.
- U. M. Nater, N. Skoluda and J. Strahler, Biomarkers of stress in behavioural medicine, Curr. Opin. Psychiatry, 2013, 26, 440–445 CrossRef.
- K. Krukowski, J. Eddy, K. L. Kosik, T. Konley, L. W. Janusek and H. L. Mathews, Glucocorticoid dysregulation of natural killer cell function through epigenetic modification, Brain, Behav., Immun., 2011, 25, 239–249 CrossRef.
- S. N. Lou and C. T. Ho, Phenolic compounds and biological activities of small-size citrus: Kumquat and calamondin, J. Food Drug Anal., 2017, 25, 162–175 CrossRef.
- Y. W. Wang, W. C. Zeng, P. Y. Xu, Y. J. Lan, R. X. Zhu, K. Zhong, Y. N. Huang and H. Gao, Chemical composition and antimicrobial activity of the essential oil of kumquat (Fortunella crassifolia Swingle) peel, Int. J. Mol. Sci., 2012, 13, 3382–3393 CrossRef.
- S. N. Lou, Y. C. Lai, Y. S. Hsu and C. T. Ho, Phenolic content, antioxidant activity and effective compounds of kumquat extracted by different solvents, Food Chem., 2016, 197, 1–6 CrossRef PubMed.
- A. Agócs, V. Nagy, Z. Szabó, L. Márk, R. Ohmacht and J. Deli, Comparative study on the carotenoid composition of the peel and the pulp of different citrus species, Innovative Food Sci. Emerging Technol., 2007, 8, 390–394 CrossRef.
- H. M. Lo, S. W. Wang, C. L. Chen, P. H. Wu and W. B. Wu, Effects of all-trans retinoic acid, retinol, and β-carotene on murine macrophage activity, Food Funct., 2014, 5, 140–148 RSC.
- K. Nagahama, N. Eto, T. Shimojo, T. Kondoh, K. Nakahara, Y. Sakakibara, K. Fukui and M. Suiko, Effect of kumquat (Fortunella crassifolia) pericarp on natural killer cell activity in vitro and in vivo, Biosci., Biotechnol., Biochem., 2015, 79, 1327–1336 CrossRef.
- M. Yagita, C. L. Huang, H. Umehara, Y. Matsuo, R. Tabata, M. Miyake, Y. Konaka and K. Takatsuki, A novel natural killer cell line (KHYG-1) from a patient with aggressive natural killer cell leukemia carrying a p53 point mutation, Leukemia, 2000, 14, 922–930 CrossRef.
- C. B. Lozzio and B. B. Lozzio, Human chronic myelogenous leukemia cell-line with positive Philadelphia chromosome, Blood, 1975, 45, 321–334 Search PubMed.
- R. Kiessling, E. Klein and H. Wigzell, “Natural” killer cells in the mouse. I. Cytotoxic cells with specificity for mouse Moloney leukemia cells. Specificity and distribution according to genotype, Eur. J. Immunol., 1975, 5, 112–117 CrossRef.
-
R. P. Adams, Identification of essential oil by gas chromatography/quadrupole mass spectroscopy, Allured Publishing Corporation, Carol Stream Illinois, 2001 Search PubMed.
- American Institute of Nutrition, Report of the American Institute of Nutrition ad hoc committee on standards for nutritional studies, J. Nutr., 1977, 107, 1340–1348 CrossRef PubMed.
- J. Bryant, R. Day, T. L. Whiteside and R. B. Herberman, Calculation of lytic units for the expression of cell-mediated cytotoxicity, J. Immunol. Methods, 1992, 146, 91–103 CrossRef.
- P. A. d'Alessio, J. F. Bisson and M. C. Béné, Anti-stress effects of d-limonene and its metabolite perillyl alcohol, Rejuvenation Res., 2014, 17, 145–149 CrossRef.
- L. Schmidt and T. Göen,
R-Limonene metabolism in humans and metabolite kinetics after oral administration, Arch. Toxicol., 2017, 91, 1175–1185 CrossRef.
- B. Cui, S. Liu, Q. Wang and X. Lin, Effect of β-carotene on immunity function and tumour growth in hepatocellular carcinoma rats, Molecules, 2012, 17, 8595–8603 CrossRef CAS PubMed.
- M. S. Santos, S. N. Meydani, L. Leka, D. Wu, N. Fotouhi, M. Meydani, C. H. Hennekens and J. M. Gaziano, Natural killer cell activity in elderly men is enhanced by beta-carotene supplementation, Am. J. Clin. Nutr., 1996, 64, 772–777 CrossRef CAS.
- K. Rajasekaran, P. Kumar, K. M. Schuldt, E. J. Peterson, B. Vanhaesebroeck, V. Dixit, M. S. Thakar and S. Malarkannan, Signaling by Fyn-ADAP via the Carma1-Bcl-10-MAP3K7 signalosome exclusively regulates inflammatory cytokine production in NK cells, Nat. Immunol., 2013, 14, 1127–1136 CrossRef CAS.
- E. Vivier, S. Ugolini and J. A. Nunès, ADAPted secretion of cytokines in NK cells, Nat. Immunol., 2013, 14, 1108–1110 CrossRef CAS.
- M. Yamaguchi, Role of carotenoid β-cryptoxanthin in bone homeostasis, J. Biomed. Sci., 2012, 19, 36 CrossRef CAS.
- K. Unno, S. Noda, Y. Kawasaki, K. Iguchi and H. Yamada, Possible Gender Difference in Anti-stress Effect of β-Cryptoxanthin, Yakugaku Zasshi, 2016, 136, 1255–1262 CrossRef CAS.
- V. Engert, S. Vogel, S. I. Efanov, A. Duchesne, V. Corbo, N. Ali and J. C. Pruessner, Investigation into the cross-correlation of salivary cortisol and alpha-amylase responses to psychological stress, Psychoneuroendocrinology, 2011, 36, 1294–1302 CrossRef CAS.
- M. D. Contreras-Aguilar, D. Escribano, S. Martínez-Subiela, S. Martínez-Miró, J. J. Cerón and F. Tecles, Changes in alpha-amylase activity, concentration and isoforms in pigs after an experimental acute stress model: an exploratory study, BMC Vet. Res., 2018, 14, 256 CrossRef.
- S. Fukumoto, A. Morishita, K. Furutachi, T. Terashima, T. Nakayama and H. Yokogoshi, Effect of flavour components in lemon essential oil on physical or psychological stress, Stress and Health, 2007, 24, 3–12 CrossRef.
- W. Zhou, M. Yoshioka and H. Yokogoshi, Sub-chronic effects of s-limonene on brain neurotransmitter levels and behavior of rats, J. Nutr. Sci. Vitaminol., 2009, 55, 367–373 CrossRef CAS.
- S. Del Toro-Arreola, E. Flores-Torales, C. Torres-Lozano, A. Del Toro-Arreola, K. Tostado-Pelayo, M. Guadalupe Ramirez-Dueñas and A. Daneri-Navarro, Effect of D-limonene on immune response in BALB/c mice with lymphoma, Int. Immunopharmacol., 2005, 5, 829–838 CrossRef CAS.
- C. M. Lappas and N. T. Lappas, D-Limonene modulates T lymphocyte activity and viability, Cell. Immunol., 2012, 279, 30–41 CrossRef CAS.
- S. R. Goltz, W. W. Campbell, C. Chitchumroonchokchai, M. L. Failla and M. G. Ferruzzi, Meal triacylglycerol profile modulates postprandial absorption of carotenoids in humans, Mol. Nutr. Food Res., 2012, 56, 866–877 CrossRef CAS PubMed.
Footnote |
† Electronic supplementary information (ESI) available. See DOI: 10.1039/c8fo01971a |
|
This journal is © The Royal Society of Chemistry 2019 |