Short-chain fatty acid (SCFA) production maximization by modeling thermophilic sludge fermentation†
Received
20th August 2018
, Accepted 15th November 2018
First published on 16th November 2018
Abstract
Producing more versatile green chemicals such as short-chain fatty acids (SCFAs) from waste activated sludge (WAS) via fermentation is a promising approach in wastewater treatment. In this study, we investigated how SCFA production can be maximized within wastewater treatment plants. An ordinary differential equation model was devised that encompasses organic inputs to a reactor as well as acetogenic and methanogenic bacterial populations. The model was calibrated and validated on an independent set of data during thermophilic sludge fermentation. A series of experiments were performed to determine the effects of reactor parameters, such as organic inputs and solid retention time (SRT), on SCFA production. Model simulation results show that optimization of SRT plays an important role in improving SCFA production. SCFA production can be enhanced with an increase in biodegradable particulate organic matter, an increase in acidogenic bacteria or a decrease in methanogens in the feed sludge. The maximum SCFA yield has been proven to benefit from thermophilic fermentation at a temperature of up to 50 °C, in which the maximum SCFA yield reaches 18% per VSS in terms of COD. The model predictions indicated that a high ratio of acidogenic bacteria to methanogens (i.e., 2
:
1) in the WAS is critical to achieve a maximum yield of approximately 30%.
Water impact
Anaerobic fermentation technology has been widely applied in wastewater treatment plants to produce useful chemicals such as short-chain fatty acids (SCFAs). The present study devised, calibrated and validated a model to optimize organic inputs, temperature and solid retention time for production of more SCFAs. Our results revealed that an increment in the ratio of acidogenic bacteria to methanogens of up to 2.0 is critical to achieve a maximum SCFA yield of approximately 30%.
|
1. Introduction
The production of short-chain fatty acids (SCFAs) from waste activated sludge (WAS) has attracted growing attention in wastewater treatment plants (WWTPs) because SCFAs allow for a wide range of applications in bioenergy, bioplastics and biological removal of nutrients from wastewater.1–5 Thermophilic fermentation has recently attracted extensive attention for treating WAS because it could increase the hydrolysis rate and produce more SCFAs than conventional mesophilic fermentation.6 Several studies have reported reactor performances and microbial responses for thermophilic sludge fermentation.7–10 Xiong et al.11 studied the influence of high temperature conditions on the hydrolysis and accumulation of SCFAs during fermentation and found that the increase in temperature from 40 to 50 °C induced a 1.65-fold increase in the SCFA yield. Hao and Wang6 observed similar results in semi-continuous fermentation reactors, and microbial analyses indicated that a higher fermentation temperature could increase the activities of key hydrolases and raise the proportions of bacteria involved in hydrolysis and acidogenesis. Additionally, the solid retention time (SRT) has proven to be an important factor for SCFA production because a short SRT has a negative effect on the population levels of methanogens.12–16 Although operational parameters such as SRT and temperature and the feed sludge composition have important impacts on the fermentation process, so far few studies have provided quantitative details on this process for SCFA production maximization.
Mathematical models have been considered as useful and convenient tools for describing, designing and optimizing biological treatment systems. An anaerobic digestion model (ADM) is a general structured model consisting of hydrolysis, acidogenesis and methanogenesis processes under anaerobic conditions.17 Modified ADM models gave good simulation results on complex anaerobic hydrolysis18–22 and methane production processes under anaerobic conditions.23–25 However, the traditional ADM-based model structure needs an enormous amount of parameter inputs and calibrations, which make model simulation difficult, especially with limited data collection in many cases. As such, some models have been simplified to improve the model identifiability and predictability. For example, a simplified sludge fermentation model was introduced to predict SCFA production under alkaline fermentation after model calibration and validation.26 However, certain parameter estimations and scenario analysis on maximizing the SCFA yield have not been investigated in current simplified models.
The objective of the present work is to calibrate and validate a simplified sludge fermentation model and then use model simulations to investigate the impacts of the feed sludge composition and operational parameters on the SCFA yield. The model calibration was performed using experimental data from batch sludge fermentation tests, and the validation procedure was carried out using independent data in a continuous reactor. The model simulation strategies include four scenarios, which respectively represent the effects of SRT, degradable particulate and active microbial components of the WAS, and temperature on the SCFA and CH4 yields as well as VSS reduction. SRT optimization and sludge pretreatment strategies to achieve maximum SCFA production are proposed based on the insights generated from the simulation results.
2. Materials and methods
2.1 Sludge fermentation model
The model was structured with seven components, including total particulate organic matter (XP), degradable particulate organic matter (XS), hydrolysate (Sh), produced SCFAs (SV), produced methane (SCH4), acidogenic bacteria (Xh) and methanogens (XV) to describe the processes of hydrolysis, fermentation, CH4 generation and acidogenic bacteria and methanogen decay. The units of the seven parameters are g COD per L. The particulate organic matter XP is the sum of degradable particulate organic matter XS and non-biodegradable particulate organic matter (XU). The components of the conceptual model for anaerobic fermentation of sludge are shown below:
The non-steady-state mass balances are as follows:
| 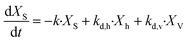 | (1) |
| 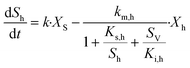 | (2) |
| 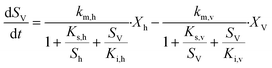 | (3) |
| 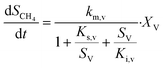 | (4) |
| 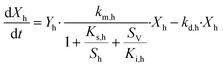 | (5) |
| 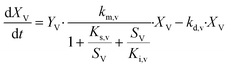 | (6) |
where
k is the hydrolysis rate constant of
XS;
kd,h is the decay rate of acidogenic bacteria and
kd,v is the decay rate of methanogens;
km,h is the maximum specific production rate of SCFAs and
km,v is the maximum specific production rate of CH
4;
Ks,h and
Ks,v are the half-saturation constant of acidogenic bacteria growth and methanogen growth, respectively;
Ki,h and
Ki,v are the inhibition constant of SCFAs on acidogenic bacteria growth and methanogen growth based on a Haldane inhibition model, respectively;
Yh and
YV are the yield of acidogenic bacteria and methanogens, respectively.
Table 1 summarizes the kinetic parameters involved in the model. The sensitivity analysis is described in the ESI
† (Fig. S1).
Table 1 Kinetic parameters for the model (50 °C)
Parameters |
Definition |
Values |
Unit |
k
|
Hydrolysis rate of degradable particulate organic matter |
2.0 |
d−1 |
k
m,h
|
Maximum specific utilization of the hydrolysate |
6.0 |
d−1 |
k
m,v
|
Maximum specific utilization of SCFAs |
1.3 |
d−1 |
k
d,h
|
Decay rate of acidogenic bacteria |
0.5 |
d−1 |
k
d,v
|
Decay rate of methanogens |
0.03 |
d−1 |
K
s,h
|
Half-saturation constant of acidogenic bacteria growth |
0.2 |
g COD L−1 |
K
s,v
|
Half-saturation constant of methanogen growth |
0.3 |
g COD L−1 |
Y
h
|
Yield of acidogenic bacteria |
0.05 |
g COD g−1 COD |
Y
V
|
Yield of methanogens |
0.05 |
g COD g−1 COD |
K
i,h
|
Inhibition constant of SCFAs on acidogenic bacteria growth |
1.5 |
g COD L−1 |
K
i,v
|
Inhibition constant of SCFAs on methanogen growth |
1.5 |
g COD L−1 |
2.2 Chemical analyses
The measurements of VSS, COD and SCFA concentrations have been described in our previous study.11 The measured SCFA concentration was converted to the model parameter SV in terms of COD by using the following conversion factors: 1.07 g COD per g acetic acid, 1.51 g COD per g propionic acid, 1.82 g COD per g butyric and iso-butyric acid, and 2.04 g COD per g valeric and iso-valeric acid. The XP in terms of COD was found to be 1.42-fold higher than the measured VSS concentration.
2.3 Calculation
The model simulation, calibration and validation procedures were all performed using the AQUASIM 2.0 software.
2.4 Model calibration using data from batch tests
The model calibration procedure was conducted by fitting the model simulation results to the measured SCFA, SCOD and VSS data in batch tests. The batch tests were conducted in a cylindrical reactor with an effective volume of 3.6 L anaerobically. The diameter of the reactor was 120 mm. A magnetic stirrer was fixed at the bottom of the reactor with a rotation speed of 1200 rpm. Heating rods were used to control the fermentation temperature at 50 °C. The used waste sludge was obtained from a drum thickener in the Xiao Hongmen WWTP, located in Beijing, China. The initial VSS, SCOD and SCFA concentrations of the sampled sludge were 15.04 g L−1, 0.56 g L−1 and 0.42 g COD L−1, respectively.
2.5 Model validation using independent data from a continuous reactor experiment
The continuous reactor experiment was carried out in the same reactor mentioned above. The operation includes two phases (Table S1†): I, start-up for 2 days, in which the WAS was only added initially; II, continuous feeding of the WAS under different SRT (2–4 days) parameter conditions. The feed sludge was the same as that above. The fermentation temperature was controlled at 50 °C. The effluent SCFA, SCOD and VSS data during the reactor operation were collected and then used for the model validation procedure.
2.6 Simulation strategies
Four simulation scenarios were designed to implement the investigation of the impacts of different SRTs, WAS compositions and temperatures on the SCFA yield during the thermophilic fermentation (Table 2). The ratios of XS/XP, Xh/XP, and XV/XP in the model represented the biodegradable particulate organic matter fraction, active acidogenic bacteria fraction and active methanogen fraction in the WAS, respectively. The variations of effluent SCFAs, CH4 and VSS in a continuous operational fermentation reactor were simulated under steady-state conditions with SRTs in range of 0.2–20 days at 50 °C. The initial XS/XP, Xh/XP, XV/XP values in the WAS are defined to be 0.35, 0.12, 0.12, respectively. The SCFA and CH4 yields are defined to be the percentage of steady-state SV and SCH4 in initial XP, respectively.
Table 2 An overview of the simulation strategies
Factors |
Simulation conditions |
Variable conditions |
The optimal SRT corresponds to the maximum SCFA yield.
|
Scenario 1: effect of SRT on SCFA and CH4 production and VSS reduction |
SRT (d) |
|
0–20 |
X
S/XP |
0.35 |
|
X
h/XP |
0.12 |
|
X
V/XP |
0.12 |
|
Temperature (°C) |
50 |
|
Scenario 2: effect of the XS/XP ratio on maximum SCFA production |
SRT (d) |
The optimal SRTa |
|
X
S/XP |
|
0.05–0.5 |
X
h/XP |
0.12 |
|
X
V/XP |
0.12 |
|
Temperature (°C) |
50 |
|
Scenario 3: combined effects of Xh/XP and XV/XP on maximum SCFA production |
SRT (d) |
The optimal SRT |
|
X
S/XP |
0.35 |
|
X
h/XP |
|
0–0.2 |
X
V/XP |
|
0–0.2 |
Temperature (°C) |
50 |
|
Scenario 4: effect of temperature on maximum SCFA production |
SRT (d) |
The optimal SRT |
|
X
S/XP |
0.35 |
|
X
h/XP |
0.12 |
|
X
V/XP |
0.12 |
|
Temperature (°C) |
|
40, 50, 60 |
2.7 Statistical analyses
The significance of model fitting results was evaluated by variance analysis, in which p < 0.05 was considered to be statistically significant and p > 0.05 showed statistical insignificance.
3. Results and discussion
3.1 Model calibration
The model calibration was conducted to fit the model simulation results on the measured VSS, SCOD and SCFA data in a short-term fermentation for two days. The VSS concentration of the sludge gradually decreased from 21.62 g COD L−1 to 16.01 g COD L−1 with the increase in SCOD and SCFA concentration (Fig. 1A). The model simulation results matched these measured data well. Two parameters, hydrolysis rate k and maximum specific utilization of the hydrolysate km,h, were calibrated to be 2.0 d−1 and 6.0 d−1 respectively. The correlation coefficient between them in 95% confidence regions was lower than 0.20, which confirmed the identifiability and reliability of the estimated parameters. The estimated k value in this work was higher than the reported values in the range of 0.005–1.2 d−1 in the literature.27 The reason was that our model assumed the hydrolysis of degradable particulate organic matter instead of total VSS in the previous model. The results indicated that the proposed model could properly reflect the relationships between the VSS reduction and SCFA production during the short-term fermentation. In addition to that, the model calibration was conducted using the measured SCOD and SCFA data in a relatively long-term experiment of 15 days. The parameter maximum specific utilization of SCFAs km,v of the methanogenesis process was estimated to be 1.3 d−1 (Fig. 1B), which is similar to the value reported in the literature.26
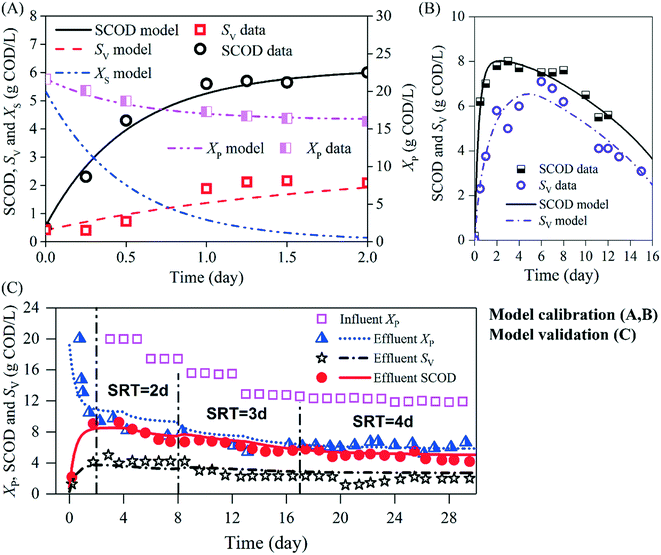 |
| Fig. 1 Model calibration using measured XP (VSS), SCOD and SV (SCFAs) data in a 2 day fermentation batch experiment at 50 °C (A), and using measured SCOD and SCFA data in a 15 day sludge fermentation experiment at 50 °C (B). Model validation using measured XP (VSS), SCOD and SV (SCFAs) from a sludge fermentation reactor operated at 50 °C (C). The lines represent the simulated results. | |
3.2 Model validation
The model validation was conducted based on statistical tests for agreement between the behavior of the model and that of the actual continuous thermophilic fermentation system. The VSS, SCOD and SCFA data were collected from the thermophilic fermentation reactor treating the WAS under different operating conditions. As shown in Fig. 1C, although complex fluctuations of VSS concentration appeared in the in-flow WAS at varied SRT operational parameters, the predictions of VSS, SCOD and SCFA concentrations in the reactor agree with the experimental data well (p < 0.05). This result indicated the validity of the proposed model, and that the calibrated model could be used to investigate the impacts of the operational parameters and sludge composition on the maximum SCFA yield from the WAS.
3.3 Model simulations
3.3.1 Effect of SRT.
The bioconversion process for SCFA production during thermophilic fermentation was investigated under different SRT operating conditions at 50 °C (scenario 1). Based on the model calibrated above, the steady-state conversion ratios for SCFAs and CH4, as well as the VSS reduction ratio along the gradient of SRT from 0.2 to 20 days were evaluated in a typical thermophilic sludge fermentation process. The simulated results showed that the SCFA yield increases from the initial value of 1%, reaches up to 18% at a SRT of 3.8 days and then gradually decreases with the increase of SRT to 20 days (Fig. 2A). The increase in SRT enhances both CH4 production and VSS reduction, which are consistent with the conventional anaerobic digestion process. The investigations on microbial community abundance showed that the methanogens would be dominant compared with the acidogenic bacteria.28 This is a good indication that the SRT optimization strategy is essential for achieving maximum SCFA production.
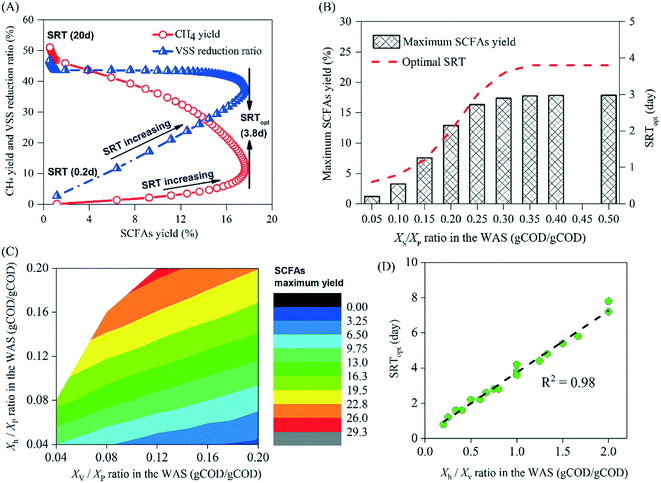 |
| Fig. 2 Model simulation results: (A) SCFA and CH4 yields, VSS reduction ratio, acidogenic bacteria and methanogens in a steady-state sludge fermentation process along the gradient of SRT from 0.2 to 20 days; (B) maximum SCFA yield with different XS/XP ratios in the WAS; (C) maximum SCFA yield with different Xh/XP and XV/XP ratios in the WAS; and (D) relationship between the optimal SRT and the ratio of acidogenic bacteria to methanogens (Xh/XV). | |
Moreover, the simulations showed that the VSS reduction ratio significantly increased from 11 to 38% with the increase in SRT from 0.2 days to 3.8 days, but further increasing the SRT up to 20 days had little effect on the enhancement of VSS reduction (Fig. 2A). These results have also been observed in previous studies on the anaerobic digestion process.11,14,16 Overall, optimization of SRT plays an important role in maximizing SCFA production, as well as achieving high-efficiency VSS reduction. As such, the effects of the WAS composition and temperature on the maximum SCFA production via SRT optimization will be further investigated in the following sections.
3.3.2 Effect of WAS composition.
In the WAS, the biodegradable particulate fraction in total VSS (XS/XP ratio) is the actual organic component involved in the hydrolysis process. Following that, the acidogenic bacteria and methanogens converted the dissolved substrate to SCFAs and CH4, respectively. The WAS usually refers to the residual, semisolid that is produced as a by-product from the wastewater biological treatment. So, the WAS composition is related to the amount and concentration of wastewater treated, as well as the type of wastewater treatment process used. Herein, we investigated the impacts of these fractions in the WAS on the maximum SCFA production.
The effect of the biodegradable particulate fraction (scenario 2) is shown in Fig. 2B; the maximum SCFA yield gradually increases up to 18% with the increase in the XS/XP ratio from 0.05 to 0.30, and then reaches a stable level as the XS/XP ratio exceeds 0.30. The model simulation showed that the optimal SRT and maximum SCFA yield both stabilized beyond an XS/XP ratio of 0.3. That is because the rate of the acidogenesis process became the limiting factor for SCFA production at a stable SRT of 3.8 d, even though a high XS/XP above 0.3 could be supplied. The result indicated that the low fraction of biodegradable particulate organic matter in the WAS would be a limiting factor to achieve a high SCFA yield. The SRT parameter optimization result depends on different XS/XP ratios in the WAS. For instance, the optimal SRT is 2 days with an XS/XP ratio of 0.2, while it increases to 4 days when the XS/XP ratio is 0.4.
The predicted variations of the maximum SCFA yield along the respective gradient of Xh/XP and XV/XP ratios from 0.04 to 0.20 are shown in Fig. 2C (scenario 3). The results showed that the SCFA accumulation benefitted from the increase in acidogenic bacteria, as well as the decrease in methanogens. The optimized results for the SRT parameter also increase with the increase in the Xh/XP ratio while they decrease with the increase in the XV/XP ratio. Furthermore, the optimal SRT was found to be linearly related to the ratio of acidogenic bacteria to methanogens (Xh/XV) with a high correlation coefficient R squared of 0.98 (Fig. 2D), indicating the key role of SRT in optimizing the microbial community structure of the fermentation reactor. The optimal SRT is as long as 8 days when the Xh/XV ratio reaches 2.0. The maximum SCFA yield under such a circumstance could reach the highest level of approximately 30% (Fig. 2C), which is 1.6-fold higher compared with the yield of 18% experimentally obtained from the sludge fermentation in this study. Selective toxicants or conditions to eliminate acid consumers at a longer SRT are definitely useful for improving the SCFA production. Recent reports had showed that FNA pretreatment of sludge could significantly eliminate the methanogen population. After the FNA treatment, the methane production rates of anaerobic biofilms took over 50 days to reach 50% recovery.29 However, the acidified FNA conditions even enhanced the key acid-forming activities by 5.9–81.8%.30 Therefore, to achieve such conditions, these experimental results indicated that the newly-developed FNA sludge pretreatment method can be used to maintain a high Xh/XV ratio at an SRT longer than 5 days.
3.3.3 Effect of temperature.
Our previous experimental studies compared SCFA production in a fermenter with temperatures of 40 °C, 50 °C and 60 °C.11 By using the reported data, the determined k and km,h in 95% confidence regions at 40 °C, 50 °C and 60 °C are shown Fig. S2.† The rate k gradually increases from 2.95 ± 0.50 to 8.18 ± 1.30 d−1 with the increase in temperature from 40 °C to 60 °C. The highest increase in km,h was attained at 60 °C, which was determined to be 6.12 ± 0.38 d−1, but it was close to the estimated value of 6.00 ± 0.47 d−1 at 50 °C. With the calibrated parameters, the model simulations for maximum SCFA production at different temperatures are shown in Table S2.† On the whole, sludge fermentation at 50–60 °C produces more SCFAs and CH4 and promotes VSS reduction compared to that at 40 °C. The maximum SCFA conversion ratio of 18% was obtained at 50 °C with an optimal SRT of 4.2 days. The results are consistent with the experimental results reported previously,11 that is, the increase in temperature from 40 °C to 50 °C induced a 1.65-fold increase in the SCFA yield.
3.4 Mechanistic analysis of SCFA production maximization
With the calibrated and validated model, these simulation results revealed that the SRT, WAS composition and temperature all significantly affected the SCFA production. Among them, an increase in the ratio of acidogenic bacteria to methanogens in the WAS is identified as the key factor to achieve the highest yield of approximately 30%. The range of high SCFA yields (20–30%) was only correlated with the ratio of acidogenic bacteria (P value < 0.0001). This indicated that optimization of the microbial community structure, i.e., selectively eliminating the methanogens, should be critical to maximize the SCFA production. According to recent studies, some sludge pretreatment methods could be effective in selectively eliminating methanogens in the WAS. For instance, with the combination of free ammonia (FA) with rhamnolipid (RL) pretreatment of sludge, Xu et al.31 reported a high SCFA production yield of 32 ± 1.4% per VSS. In this case, acidogenesis and methanogenesis were both inhibited, but the inhibition of methanogenesis was much stronger than that of acidogenesis. Attributed to the pyrosequencing method, it had been found that some fermenters could play important roles in efficient SCFA production. These microorganisms included Pseudomonas sp., Alcaligenes sp., and Clostridium sp., which had been demonstrated to have the capacity to produce SCFAs.32 Further study on key microbes as well as their responses to sludge pretreatment should be essential to reveal the mechanisms of SCFA production maximization.
Thermophilic fermentation at high temperature has significant effects on both k and km,h. The results confirmed that the thermophilic range of temperatures induced biochemical reaction rate acceleration and higher growth rates of microorganisms.7 Therefore, thermophilic fermentation can lead to greater accumulation of SCFAs than mesophilic fermentation.6 The effect of the reaction is not always positively correlated with the temperature in anaerobic digestion. Thermophilic fermentation produces more SCFAs than mesophilic fermentation but it still requires higher energy consumption due to heating. Therefore, the balance between the benefit and cost should be considered and further evaluated in future study.
3.5 Potential pretreatment technology for enhancing SCFA production
Previous studies had reported that sludge fermentation with high pH (>10),26,33–35 salinity36–38 or surfactant concentration4 could promote SCFA production. These conditions improved the hydrolysis and acidification processes but in situ inhibited methanogenesis in sludge fermentation reactors. In this study, the simulations indicated that pretreatment of sludge is also effective for weakening the methanogenesis process during sludge fermentation. Sludge pretreatment generated a high biodegradable particulate fraction (XS). On the other hand, some possible pretreatment methods can effectively inactivate the methanogens in the WAS. The growth of the methanogens (XV) was then limited to a low level with an appropriate SRT operational parameter in fermentation. Recent studies on free nitrous acid (FNA)-based sludge pretreatment technology suggested that sludge solubilization, hydrolysis and acidification could be enhanced but the activities of methanogens were inhibited after FNA pretreatment of the sludge.30,39 The reason is that FNA (a biocidal agent produced from a side-stream process in a WWTP40 or from source-separated urine41) can cause microbial cell death/lysis.29 Attributed to FNA pretreatment of the sludge, the increase in the ratio of acidogenic bacteria to methanogens can further enhance the SCFA production.
4. Conclusion
In this letter, a fermentation model was devised to optimize key reactor parameters for producing more SCFAs. The model was calibrated and validated well by batch tests and long-term operational data, respectively. The model simulation results showed that optimization of SRT plays an important role in maximizing SCFA production; increasing the fraction biodegradable particulate organic matter or the ratio of acidogenic bacteria (Xh) to methanogens (XV) can further improve SCFA production. The model simulation results revealed the SCFA yield could reach the highest level of approximately 30% when the optimal SRT is as longs as 8 days as well as when the Xh/XV ratio reaches 2.0. However, to increase the Xh/XV ratio in the sludge, it is imperative to develop some sludge pretreatment technologies, selectively eliminating the methanogens over acidogenic bacteria in the sludge.
Conflicts of interest
There are no conflicts to declare.
Acknowledgements
This research was supported by the National Natural Science Foundation of China (No. 51678337, No. 51708326), the Major Science and Technology Program for Water Pollution Control and Treatment of China (No. 2014ZX07305001). Dr. Min Zheng acknowledges the support of the international Postdoctoral Exchange Fellowship Program (China).
References
- Y. Chen, A. A. Randall and T. McCue, The efficiency of enhanced biological phosphorus removal from real wastewater affected by different ratios of acetic to propionic acid, Water Res., 2004, 38, 27–36 CrossRef CAS PubMed.
- P. Elefsiniotis, D. G. Wareham and M. O. Smith, Use of volatile fatty acids from an acid-phase digester for denitrification, J. Biotechnol., 2004, 114, 289–297 CrossRef CAS PubMed.
- C. Mengmeng, C. Hong, Z. Qingliang, S. N. Shirley and R. Jie, Production of polyhydroxyalkanoates (PHA) in activated sludge fed by volatile fatty acids (VFAs) generated from alkaline excess sludge fermentation, Bioresour. Technol., 2009, 100, 1399–1405 CrossRef PubMed.
- S. Jiang, Y. Chen, Q. Zhou and G. Gu, Biological short-chain fatty acids (SCFAs) production from waste-activated sludge affected by surfactant, Water Res., 2007, 41, 3112–3120 CrossRef CAS PubMed.
- J. Tong and Y. Chen, Enhanced biological phosphorus removal driven by short-chain fatty acids produced from waste activated sludge alkaline fermentation, Environ. Sci. Technol., 2007, 41, 7126–7130 CrossRef CAS PubMed.
- J. Hao and H. Wang, Volatile fatty acids productions by mesophilic and thermophilic sludge fermentation : biological responses to fermentation temperature, Bioresour. Technol., 2015, 175, 367–373 CrossRef CAS PubMed.
- J. Lu, H. N. Gavala, I. V. Skiadas, Z. Mladenovska and B. K. Ahring, Improving anaerobic sewage sludge digestion by implementation of a hyper-thermophilic prehydrolysis step, J. Environ. Manage., 2008, 88, 881–889 CrossRef CAS.
- H. N. Gavala, U. Yenal, I. V. Skiadas, P. Westermann and B. K. Ahring, Mesophilic and thermophilic anaerobic digestion of primary and secondary sludge. effect of pre-treatment at elevated temperature, Water Res., 2003, 37, 4561–4572 CrossRef CAS PubMed.
- H. Siegrist, D. Vogt, J. L. Garcia-Heras and W. Gujer, Mathematical model for meso- and thermophilic anaerobic sewage sludge digestion, Environ. Sci. Technol., 2002, 36, 1113–1123 CrossRef CAS PubMed.
- C. Chu, K. Xu, Y. Li and Y. Inamori, Hydrogen and methane potential based on the nature of food waste materials in a two-stage thermophilic fermentation Process, Int. J. Hydrogen Energy, 2012, 37, 10611–10618 CrossRef CAS.
- H. Xiong, J. Chen, H. Wang and H. Shi, Influences of volatile solid concentration, temperature and solid retention time for the hydrolysis of waste activated sludge to recover volatile fatty acids, Bioresour. Technol., 2012, 119, 285–292 CrossRef CAS PubMed.
- S. Bengtsson, J. Hallquist, A. Werker and T. Welander, Acidogenic fermentation of industrial wastewaters : effects of chemostat retention time and pH on volatile fatty acids production, Biochem. Eng. J., 2008, 40, 492–499 CrossRef CAS.
- W. S. Lee, A. Seak, M. Chua, H. K. Yeoh and G. C. Ngoh, A review of the production and applications of waste-derived volatile fatty acids, Chem. Eng. J., 2014, 235, 83–99 CrossRef CAS.
- Y. Miron, G. Zeeman, J. B. Van Lier and G. Lettinga, The role of sludge retention time in the hydrolysis and acidification of lipids, carbohydrates and proteins during digestion of primary sludge in CSTR systems, Water Res., 2000, 34, 1705–1713 CrossRef CAS.
- M. A. De Rubia, M. Perez, L. I. Romero and D. Sales, Effect of solids retention time (SRT) on pilot scale anaerobic thermophilic sludge digestion, Process Biochem., 2006, 41, 79–86 CrossRef.
- P. Zhang, Y. Chen, T. Y. Huang and Q. Zhou, Waste activated sludge hydrolysis and short-chain fatty acids accumulation in the presence of SDBS in semi-continuous flow reactors: effect of solids retention time and temperature, Chem. Eng. J., 2009, 148, 348–353 CrossRef CAS.
- D. J. Batstone, J. Keller, I. Angelidaki, S. V. Kalyuzhnyi, S. G. Pavlostathis, A. Rozzi, W. T. Sanders, H. Siegrist and V. A. Vavilin, The IWA anaerobic digestion model No 1 (ADM1), Water Sci. Technol., 2002, 45, 65–73 CrossRef CAS.
- V. A. Vavilin, B. Fernandez, J. Palatsi and X. Flotats, Hydrolysis kinetics in anaerobic degradation of particulate organic material: an overview, Waste Manage., 2008, 28, 939–951 CrossRef CAS PubMed.
- A. Donoso-Bravo, S. I. Pérez-Elvira and F. Fdz-Polanco, Application of simplified models for anaerobic biodegradability tests. evaluation of pre-treatment processes, Chem. Eng. J., 2010, 160, 607–614 CrossRef CAS.
- H. Yasui, R. Goel, Y. Y. Li and T. Noike, Modified ADM1 structure for modelling municipal primary sludge hydrolysis, Water Res., 2008, 42, 249–259 CrossRef CAS PubMed.
- V. A. Vavilin, S. V. Rytov and L. Y. Lokshina, A description of hydrolysis kinetics in anaerobic degradation of particulate organic matter, Bioresour. Technol., 1996, 56, 229–237 CrossRef CAS.
- I. Ramirez, A. Mottet, H. Carrère, S. Déléris, F. Vedrenne and J.-P. Steyer, Modified ADM1 disintegration/hydrolysis structures for modeling batch thermophilic anaerobic digestion of thermally pretreated waste activated sludge, Water Res., 2009, 43, 3479–3492 CrossRef CAS PubMed.
- Y. Liu, B. Ni, R. Ganigué, U. Werner, K. R. Sharma and Z. Yuan, Sulfide and methane production in sewer sediments, Water Res., 2015, 70, 350–359 CrossRef CAS PubMed.
- Y. Liu, K. R. Sharma, B. Ni, L. Fan, S. Murthy, G. Q. Tyson and Z. Yuan, Effects of nitrate dosing on sulfidogenic and methanogenic activities in sewer sediment, Water Res, 2015, 74, 155–165 CrossRef CAS PubMed.
- M. Maurer, W. Pronk and T. A. Larsen, Treatment processes for source-separated urine, Water Res., 2006, 40, 3151–3166 CrossRef CAS PubMed.
- P. Zhang, Y. Chen, Q. Zhou, X. Zheng, X. Zhu and Y. Zhao, Understanding short-chain fatty acids accumulation enhanced in waste activated sludge alkaline fermentation: kinetics and microbiology, Environ. Sci. Technol., 2010, 44, 9343–9348 CrossRef CAS PubMed.
- B. Jin, S. Wang, L. Xing, B. Li and Y. Peng, The effect of salinity on waste activated sludge alkaline fermentation and kinetic analysis, J. Environ. Sci., 2015, 43, 80–90 CrossRef PubMed.
- Y. Yan, L. Feng, C. Zhang, C. Wisniewski and Q. Zhou, Ultrasonic enhancement of waste activated sludge hydrolysis and volatile fatty acids accumulation at pH 10.0, Water Res., 2010, 44, 3329–3336 CrossRef CAS PubMed.
- G. Jiang, O. Gutierrez and Z. Yuan, The strong biocidal effect of free nitrous acid on anaerobic sewer biofilms, Water Res., 2011, 45, 3735–3743 CrossRef CAS PubMed.
- J. Zhao, D. Wang, X. Li, Q. Yang, H. Chen, Y. Zhong and G. Zeng, Free nitrous acid serving as a pretreatment method for alkaline fermentation to enhance short-chain fatty acid production from waste activated sludge, Water Res., 2015, 78, 111–120 CrossRef CAS PubMed.
- Q. Xu, X. Liu, Y. Fu, Y. Li, D. Wang, Q. Wang, Y. Liu, H. An, J. Zhao, Y. Wu, X. Li, Q. Yang and G. Zeng, Feasibility of enhancing short-chain fatty acids production from waste activated sludge after free ammonia pretreatment: role and significance of rhamnolipid, Bioresour. Technol., 2018, 267, 141–148 CrossRef CAS PubMed.
- X. Zheng, Y. Su, X. Li, N. Xiao, D. Wang and Y. Chen, Pyrosequencing reveals the key microorganisms involved in sludge alkaline fermentation for efficient short-chain fatty acids production, Environ. Sci. Technol., 2013, 47, 4262–4268 CrossRef CAS PubMed.
- Y. Chen, S. Jiang, H. Yuan, Q. Zhou and G. Gu, Hydrolysis and acidification of waste activated sludge at different pHs, Water Res., 2007, 41, 683–689 CrossRef CAS PubMed.
- H. Liu, J. Wang, X. Liu, B. Fu, J. Chen and H. Q. Yu, Acidogenic fermentation of proteinaceous sewage sludge: effect of pH, Water Res., 2012, 46, 799–807 CrossRef CAS PubMed.
- H. Yuan, Y. Chen, H. Zhang, S. Jiang, Q. Zhou and G. Gu, Improved bioproduction of short-chain fatty acids (SCFAs) from excess sludge under alkaline conditions, Environ. Sci. Technol., 2006, 40, 2025–2029 CrossRef CAS PubMed.
- J. Zhao, C. Zhang, D. Wang, X. Li, H. An, T. Xie, F. Chen, Q. Xu, Y. Sun, G. Zeng and Q. Yang, Revealing the underlying mechanisms of how sodium chloride affects short-chain fatty acid production from the cofermentation of waste activated sludge and food waste, ACS Sustainable Chem. Eng., 2016, 4, 4675–4684 CrossRef CAS.
- G. Su, S. Wang, Z. Yuan and Y. Peng, Enhanced volatile fatty acids production of waste activated sludge under salinity conditions : performance and mechanisms, J. Biosci. Bioeng., 2016, 121, 293–298 CrossRef CAS PubMed.
- B. Jin, S. Wang, L. Xing, B. Li and Y. Peng, Effect of salinity on enhancing waste activated sludge alkaline fermentation at different temperature, Clean, 2016, 44, 1750–1758 CAS.
- X. Li, J. Zhao, D. Wang, Q. Yang, Q. Xu, Y. Deng, W. Yang and G. Zeng, An efficient and green pretreatment to stimulate short-chain fatty acids production from waste activated sludge anaerobic fermentation using free nitrous acid, Chemosphere, 2016, 144, 160–167 CrossRef CAS PubMed.
- Y. Law, L. Ye, Q. Wang, S. Hu, M. Pijuan and Z. Yuan, Producing free nitrous acid - a green and renewable biocidal agent - from anaerobic digester liquor, Chem. Eng. J., 2015, 259, 62–69 CrossRef CAS.
- M. Zheng, Z. Zuo, Y. Zhang, Y. Cui, Q. Dong, Y. Liu, X. Huang and Z. Yuan, Nitrite production from urine for sulfide control in sewers, Water Res., 2017, 122, 447–454 CrossRef CAS PubMed.
Footnote |
† Electronic supplementary information (ESI) available. See DOI: 10.1039/c8ew00572a |
|
This journal is © The Royal Society of Chemistry 2019 |
Click here to see how this site uses Cookies. View our privacy policy here.