Organic contaminants of emerging concern in Norwegian digestates from biogas production†
Received
12th April 2019
, Accepted 10th June 2019
First published on 1st July 2019
Abstract
The aim of this study was to analyze a variety of environmental organic contaminants of emerging concern (CEC) and their metabolites in representative digestate samples from Norwegian biogas production plants. Biogas digestates can be a valuable source for soil amendments and/or fertilizers in commercial agriculture. It is important to assess whether the digestates contain harmful contaminants in order to avoid unintended exposure of human consumers. In total 19 biogas digestates from 12 biogas production plants in Norway were collected and analyzed. Furthermore, process related parameters such as pretreatment of substrates, additives, flocculation and temperature conditions were considered for interpretation of the results. The CEC levels found in the digestates were shown to be dependent on the original composition of the substrate, dry-matter content, and conditioning of the substrate. The sunscreen octocrylene (147 μg L−1) and acetaminophen (paracetamol; 58.6 μg L−1) were found at the highest concentrations in liquid digestates, whereas octocrylene (>600 ng g−1, on a wet weight basis = ww) and the flame retardant TCPP (tris(1-chloro-2-propyl) phosphate, >500 ng g−1 ww) were found at the highest levels in solid digestates, exceeding even the upper limit of quantification (uLOQ) threshold. The highest levels of total CECs were measured in solid digestates (1411 ng g−1 ww) compared to liquid digestates (354 μg L−1 equals 354 ng g−1). The occurrence of CECs in digestate samples, even after extensive and optimized anaerobic digestion, indicates that the operational conditions of the treatment process should be adjusted in order to minimize CEC contamination.
Environmental significance
Biogas digestates are considered valuable fertilizers and soil amendments with agricultural applications. The study reported herein describes the quantification of certain organic contaminants of emerging concern (CECs) in liquid and solid anaerobic digestates from twelve biogas production facilities in Norway. The concentration of CECs was found to depend on the composition of the initial substrate (dry matter content) and how it was conditioned or pretreated prior to anaerobic digestion. The anaerobic digestion process employed at the biogas production facilities did not eliminate the analytes investigated.
|
Introduction
In order to reduce global anthropogenic CO2 production and emission, and to further the utilization of suitable renewable resources, the development of sustainable bioeconomic strategies has been given a high priority on the political agenda of today's world leaders. An important prerequisite strategy, in terms of industrial processes based on biological materials in a circular economy, is sustainable management of the residues, and hence recycling of nutrients from the source material will be imperative.1–5 There is huge diversity in organic residues depending on their origin and/or the type of process involved in their production.6,7 Application of organic residues as a soil amendment and fertilizer is expected to recycle most of the nutrients contained within. However, it may also imply a risk for the dispersal of contaminants on agricultural soils.8,9 From soils, contaminants may be absorbed into food and fodder plants, from where it may ultimately find its way into animal and human food chains.
The use of biological (waste) material in anaerobic digestion (AD), both in decentralized biogas plants on farms and in municipal plants for handling, among others, organic household waste, has increased significantly in Europe and North America.10–14 AD is a microbiological process where organic material (hereafter named the substrate) is degraded in an oxygen free environment (digesters), producing biogas and biogas digestate. This biogas is an energy rich gas consisting of methane, carbon dioxide and other trace gases (including H2). This development has not only led to an increasing amount of bioenergy being produced, but has also contributed to a considerable amount of organic residue being properly handled (i.e., biogas digestate). Consequently, digestates are currently applied as organic fertilizer to agricultural land, allowing the recovery of nutrients, primarily nitrogen and phosphorus, and, in addition, potentially improving soil quality by adding organic matter.15–18 Unfortunately, these biogas digestates may also contain harmful chemical pollutants and/or pathogenic bacteria, which may represent both environmental and human health risks.19–22 Biogas digestate is today considered an excellent bio-fertilizer and a soil amendment for agricultural applications.23–28 The physicochemical properties of digestate depend mainly on the nature of the substrate and operating conditions of the digester. Compared to raw animal manures and slurries, digestates generally contain low total solids and have a low organic carbon content, a low carbon to nitrogen ratio (C
:
N), and low viscosity.29–31 The typical pH value of fresh digestate ranges from 7.5 to 8.0, comparable to that of raw animal manures and slurries.32 Anthropogenic contaminants and hazardous pollutants have previously been reported in digestates.19,22,33 Such contaminants may influence the function of microorganisms during the AD process if present in the substrate or generated in the digester.25,34
However, only a few scientific studies have reported on the presence and fate of organic pollutants, such as dioxin-like compounds,35–37 polycyclic aromatic hydrocarbons (PAH),38,39 polychlorinated biphenyls (PCBs) and pesticides,39,40 in digestates (in a ng kg−1 to μg kg−1 range). Some organic contaminants such as mycotoxins may be effectively removed during the anaerobic digestion process.41 However, contaminants of emerging concern (CECs) have not yet been comprehensively investigated in biogas digestates.
Ultimately, nutrient rich and unpolluted digestate is expected and required for safe recycling and application in agricultural production. Hence, an important premise for producing high-quality digestate as a fertilizer in agricultural production is the use of high-quality substrates (rich in plant nutrients and without pollution and impurities) for the digestion process.42
Our study focuses on the occurrence of organic CECs including a phosphate containing flame retardant tris-(1-chloro-2-propyl) phosphate (TCPP), an insect repellant (DEET), a sunscreen ingredient, 25 pharmaceuticals and personal care products (PPCPs) and 11 selected metabolites in digestate samples collected from several representative biogas plants in Norway. The effect of the substrate composition and plant specific pretreatment procedures on the concentration of these contaminants in digestate samples was elucidated. For this purpose, a new optimized quantitative trace-analytical method was developed. The optimized quantification method was applied for the characterization of CEC patterns in 19 representative Norwegian biogas digestate samples (including one substrate sample). Based upon these results, a first assessment of levels and consequences of CEC residues in soils treated with digestates as soil amendments is reported.
Materials and methods
Contaminants and reagents
The sample preparation and quantification method are fully described in the ESI material section.† 41 reference standards and 10 isotope-labelled internal standards (ISTD) were purchased in trace analytical quality (≥97%) from Sigma-Aldrich (St. Louis, MI, USA) and Toronto Research Chemicals (Toronto, Ontario, Canada). These target compounds were selected based on their high prescription rate in Norway and their reported presence in related environmental samples. Metabolites for some compounds were selected based on their availability as purified standard materials. Complete information on the contaminants studied and the standards applied for the present reported study can be found in Table S1 (ESI material section†).
Biogas process conditions
Representative digestate samples from 12 major commercial biogas plants in Norway were collected during late autumn 2017. The general process conditions are listed in Table 1. Since most biogas plants use sewage sludge as a substrate, most of digestates are dewatered and decomposed. However, some food waste biogas plants supply liquid digestate as fertilizer for agriculture. In order to separate the digestate into a liquid and a solid digestate, a flocculant (e.g. cationic polymer) is added to destabilize colloidal materials and hence improve their sedimentation. Digestate is subsequently dewatered by centrifugation. Both liquid and solid digestates were analyzed in this study.
Table 1 Plant specific procedure strategies for optimized biogas production in representative Norwegian biogas production plantsa
Location |
Solid (S)/liquid (L) sample available |
Substrate |
Reactor temperature [°C] |
Retention time [days] |
Inoculum |
Pre treatment |
Precipitant added |
Dry matter [%] |
Polymer |
THP = thermal hydrolysis processing; temp. = pre-treatment at high temperature (70–165 °C for 20–60 min); L = liquid samples; S = solid samples; Act. Sl. = activated sludge; EcoFloc = liquid, commercially available flocculant (Ecolab, Naperville, Il, USA) mainly composed of FeCl3 in water solution. For details on polymers added to the digestates, see Table S11.
|
A |
L + S |
45% food waste, 53% sewage sludge, 2% fish silage |
40 |
16 |
No |
THP |
FeCl3 |
L = 5; S = 47 |
Yes |
B |
S |
15% food waste, 85% sewage sludge |
40 |
40 |
Yes |
THP |
No |
S = 26 |
Yes |
C |
L |
72% food waste, 18% manure |
35 |
35 |
Yes |
Temp. |
FeCl3 |
L = 5; S = 47 |
Yes |
D |
S |
100% sewage sludge |
37 |
20 |
No |
No |
FeCl3 & AlCl3 |
S = 47 |
Yes |
E |
S + L |
100% sewage sludge |
41 |
35 |
Yes |
THP |
No |
L = 3; S = 27 |
Yes |
F |
S + L |
45% food waste, 65% sewage sludge |
62 |
20 |
Yes |
Temp. |
FeCl3 |
S = 22 |
Yes |
G |
L |
100% food waste |
37 |
20 |
No |
No |
No |
L = 5 |
No |
H |
S |
100% sewage sludge |
37 |
15 |
No |
No |
No |
S = 26 |
Yes |
I |
S + L |
20% sludge from young fish, 80% manure |
40 |
40 |
No |
Temp. |
No |
L = 5; S = 33 |
Yes |
J |
S |
100% sewage sludge |
55 |
14 |
No |
No |
FeSO4 × 7H2O |
S = 32 |
Yes |
K |
S |
100% sewage sludge |
40 |
25 |
No |
THP |
EcoFloc |
S = 33 |
Yes |
L |
S + L |
100% food waste |
53 |
20 |
No |
No |
FeS |
L = 2; S = 35 |
No |
For dewatering of the digestate at 9 biogas plants, the cationic polymers listed in Table S11† were added to the digestates. The list of contributing Norwegian biogas plants was anonymized and coded as plants A–L in the following sections (Table 1). For this first survey, 19 samples were investigated (all samples were prepared and analyzed in replicates). The sample set also included one liquid substrate sample (Isub) and a liquid digestate sample (Idig) from an experimental biogas reactor associated with plant I. Biogas plant I uses 20% sludge from young fish and 80% manure as a raw substrate (Table 1). As preparation for effective anaerobic digestion and for optimization of the biogas yield (mainly CH4), the biogas plants use different conditioning steps, pretreatments and process adaptations, which may influence the contaminant levels in the digestates. Hence, in addition to the levels of organic contaminant residues, the influence of the biogas production parameters including pretreatment, additives, and temperature conditions on the resulting levels of these organic pollutants was considered in the statistical interpretation of the obtained CEC levels. The 12 stations and the production processes investigated in our study are considered representative of today's biogas production strategies both in Norway and internationally. Modifications of reactor conditions (i.e., reactor temperature), chemical composition of the substrate (i.e. by thermal hydrolysis or chemical reactions) and physical state (i.e. polymer addition or flocculants) are necessary for process optimization purposes (see Table 1). Differences in the substrate composition (various mixtures of food waste, fish silage, biosolids, manure, sewage sludge, etc.) and the resulting physical consistency of the starting material require specific adjustments for optimal processing conditions. An inoculum (e.g., manure) is added to initiate biogas production. Thermal pretreatment (including thermal hydrolysis = THP) is often used as a hygienization step but can also make the substrate more biodegradable for the subsequent biogas production process. Furthermore, additives like organic polymers and flocculants/precipitants (inorganic salts) are added to the digestates in order to bind nutrients like phosphorous. The chosen pretreatment procedures are also dependent on the dimensions and operational conditions of the respective biogas production plants (Table 1).
Analytical methods
For this study, a method for simultaneous quantification of 41 contaminants of emerging concern (CECs), including some transformation products of some compounds, was developed and applied (for details see the ESI†). The target CECs were extracted by solvent extraction followed by cleanup with solid phase extraction (SPE). For the quantification of the target compounds, liquid chromatography-electrospray ionization/tandem mass spectrometry (LC-ESI-MS/MS) was used.
The quality control protocol for the quantitative analysis, including linearity testing and documenting of non-linear matrix effects on the quantification of the target substances, was performed according to a method described in earlier related studies.43,44
Statistical methods
Principal component analysis (PCA) was performed and Pearson's correlation coefficients were calculated with R-software (R-Studio Version 1.1.143 based on R version 3.5.2.) under the GNU public license (Boston, MA, USA) and Matlab (Version 8, Mathworks, Natick, MA, USA), respectively. The significance threshold for Pearson's correlation coefficient calculation was considered to be p < 0.05.
Results and discussion
Detection of CECs in digestate samples
Concentration levels of the target CECs which met the quality control criteria of the method validation are listed in Tables S9 and S10.† Representative chromatograms and mass transitions used for their quantitative analysis are presented in Fig. S3–S17 in the ESI.† For the liquid digestates, 28 target compounds were quantified, whereas for solid digestates only 24 target contaminants met the quality control criteria for quantification and were reported in ng g−1 wet weight (ww). Ranitidine, metronidazole, trimethoprim, norfluoxetine HCl, warfarin, and carbamazepine-10,11-epoxide were not found in liquid samples (Table S10†). Of the 24 contaminants quantified in the solid digestate, 16 compounds were detected. For two solid digestate samples (E(S) and B(s)), TCPP and octocrylene concentrations were found outside the confirmed linear range of the quantification method. The levels were reported to be above the upper limit of quantification (>uLOQ, see Table S10†).
Substrate composition
In general, the concentrations in solid digestates were considerably higher compared to those in liquid sample materials. For solid digestates (dry matter content of 22–47%), sum-CEC concentrations exceeding 1000 ng g−1 were determined (Table S10:†E(S), B(s)). The highest sum-CEC levels in liquid digestates (dry matter content of 2–5%) were found in the concentration range 22.3–353.6 μg L−1 (Table S9†).
The CECs found in the various digestates, and their levels, seem directly related to the substrate used. The highest residue levels for pharmaceuticals exclusively used in human therapy (i.e. carbamazepine, metoprolol, losartan) were found in liquid and solid digestates originating mostly from sludge and food waste substrates. Monesin, an antibacterial agent only used in livestock breeding, was found in one digestate sample from a 100% food waste-based substrate. High levels of octocrylene (a sun-screen ingredient), in some cases even exceeding the uLOQ method limit, were found almost exclusively in digestates when sewage sludge was used for biogas production. The highest sum-CEC concentration was confirmed in a solid digestate sample after processing a mixed food waste/sewage sludge substrate (B(S)).
Biogas production and processing
For the optimisation of the biogas process with respect to quality and yield, plant-specific adjustments and treatment procedures are implemented in the production process (Table 1). These treatment steps may also have effects on the levels and distribution patterns of the quantified target CECs.
The calculation of Pearson correlation coefficients for the various preparation steps listed in Table 1 revealed the complex picture of the influence of substrate conditioning on the CEC levels in the resulting digestates (Fig. 1). The correlation calculations revealed a significant positive correlation between the CEC level and the use of thermal hydrolysis (PTTHP) in the pre-treatment of the substrate prior to AD as well as the dry-matter content (% DM). This is not surprising since earlier investigations have confirmed that pollutants, immobilised during biological sewage treatment through phase II metabolising enzymes such as glutathione S-transferases or UDP-glucoronosyltransferases, may be reactivated again by cleaving the conjugates with subsequent hydrolysis.45–48 In fact, such cleavage methods are frequently used for quantification of conjugated anthropogenic pollutants in biological samples.49–53 In this context combined thermal treatment, including thermal hydrolysis, where high temperature is applied in combination with catalytic or direct chemical reactions (oxidation, reduction, elimination, photochemistry, etc.) is often performed for cleaving of conjugates, initiating the breakdown of cell walls and solubilization of organic matter.54 Thermal hydrolysis is, hence, expected to change the availability and the partitioning of the target contaminants.
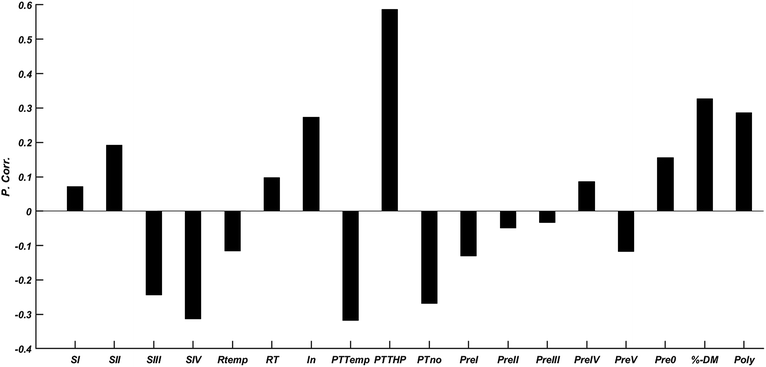 |
| Fig. 1 Relative Pearson correlation coefficients (P. corr. maximum range: −1 to +1) for the conditioning processes related to the obtained CEC concentrations. Statistical data related to available information on biogas processing and CEC concentrations measured in the respective digestate samples. % DM and PTTHP showed positive and significant correlations (P.corr. = 0.57 and 0.59 respectively, p < 0.05). | |
Adding a reactive inoculum as well as providing high dry matter content (% DM) may probably contribute to elevated CEC levels in the final digestates (Fig. 1). Adding precipitants (flocculants) to the digestate is also not expected to contribute to reduced CEC levels according to our preliminary findings.
The results presented in Fig. 1 indicate that the careful selection of substrate composition (including dry matter content) and optimised conditioning strategies for biogas production may be considered a first important step to reduce the occurrence of potential CECs in the digestate.
CEC distribution profiles
Levels and distribution profiles of contaminants in digestate samples depend on a variety of ambient factors. These include the original contaminant profile in the substrate sample, physicochemical properties of the substance, interactive processes with the substrate material during the anaerobic digestion (AD) process, and microbiological transformation processes during the digestion process. Hence, in the literature, selected contaminants are found with high removal efficiency by AD. Among these, caffeine,55,56 trimethoprim55,57 and sulfamethoxazole58 have been reported to be readily degraded and removed in such processes. However, other substances like carbamazepine and fluoxetine were found to be stable and, thus, are not expected to degrade during AD.55,57–59
To our knowledge, no previous studies have been reported in the literature yet, where indications for the partition of CECs (like those selected here) and their transformation products between liquid and solid biogas digestates were found. Based on the results available here, a first attempt is made to evaluate the physicochemical properties of the target contaminants and their influence on the final distribution profiles found in the respective digestates (Tables S9 and S10†). For this purpose, calculated partitioning coefficients (directly derived from the ACD/Labs Percepta Platform – PhysChem Module, Toronto, CA) were compared and discussed in relation to the distribution profiles identified in the digestates of the twelve plants (Table S1†). Hence, soil organic carbon–water partitioning coefficients (log
Koc) along with the octanol–water partitioning coefficient (log
P) and the pH dependent octanol–water distribution constant (log
D) for all target CECs are listed in Table S1† for comparison. Since these coefficients are quotients of concentrations, the calculated values are dimensionless.
Log
D is considered the most reliable descriptor for the ionisable CECs in our study. Substances with low log
D, log
P and log
Koc coefficients are expected to be enriched in solution whereas high values indicate sorption to particulate material and thus a tendency to reach higher levels in the solid phase (Table S1†). Carboxy-ibuprofen has the lowest log
D (log
D = −2.65) and should thus mainly be found in liquid digestates. However, carboxy-ibuprofen, a major transformation product of ibuprofen was only found in one liquid and one solid sample.
Octocrylene belongs to the CECs with the highest concentrations in both liquid and solid digestates. The high log
D (=6.34) and log
P (=7.53) indicate that octocrylene is found mainly adsorbed to particle surfaces. TCPP, on the other hand, is also found in high concentrations in both solid and liquid digestates (see Table S1†), even exceeding the uLOQ threshold in solid samples. The log
D (=1.53) and log
P (=0.48) indicate that TCPP is more likely to be detected in aqueous environments. It is also important to note that solid digestate contains a considerable amount of water (53–78%). Thus, it is not surprising to also detect water-soluble compounds in solid digestate samples (and vice versa).
Hence, the theoretical partitioning coefficients are to be considered indicators based upon inherent physicochemical properties of the investigated target contaminants.
The relative distribution of the CECs, calculated from the concentrations reported in Tables S9 and S10,† and presented in Fig. 2, indicates a matrix dependent distribution. While atorvastatin, TCPP, and octocrylene are predominant in solid digestate samples (sum = 54%), acetaminophen, prednisolone and octocrylene are observed to be the predominant CECs in liquid digestates (sum = 65%).
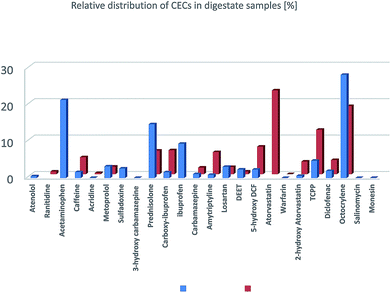 |
| Fig. 2 Average relative distributions of selected CECs (100% = sum CECs) detected and quantified in solid (red) and liquid (blue) digestate samples. | |
These differences are, however, not caused only by the water content of the samples (Table 1: liquid digestates 95–98% water content, solid digestates: 53–78% water content). As earlier outlined, the final distribution patterns are rather a consequence of the variability and interaction between substrate composition, water content, substrate preparation and physicochemical properties of the target contaminants. This is illustrated in Fig. 3, where principal component analysis (PCA) describes the influence of the identified variables (see Table 1) and the physicochemical properties (Table S1:† partitioning coefficients) on the CEC profiles in the investigated digestates. The two major principal components (PC1 & PC2) account for only approximately 40% of the overall variability in the dataset. Thus, the complexity of the influencing variables and their interactions are not completely explained by the parameters identified here.
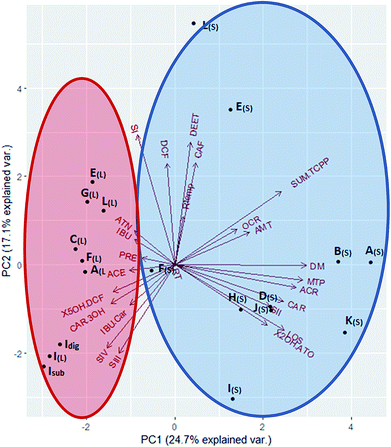 |
| Fig. 3 Bi-plot for Principal Component Analysis (PCA) conducted for variables potentially influencing the CEC distribution profile in the analysed digestate samples. Red circle (liquid digestates); blue circle (solid digestates). All abbreviations are explained in Table S1† and Fig. 1. | |
The liquid samples (red circle) are separated in a well-defined group, where the water soluble CECs dominate the distribution profile (i.e., ACE, DCF, and CAR-3OH). The substrate composition (SI–SIV) seems to have a stronger influence on the CEC profile of liquid samples compared to the solid digestates. In particular, the composition and content of SI (food waste), SIII (fish silage) and SIV (bio waste) may influence the CEC patterns in the liquid digestates investigated here.
For the solid digestates (dry matter: 22–47%), microbial transformation products, such as acridine (carbamazepine metabolite), and 3-hydroxy-atorvastatin seem to have a large influence on the overall CEC patterns.
Levels of CECs and their major transformation products in biogas digestates
For identification of potential CEC transformation during the anaerobic microbial digestion processes (resulting in the production of biogas), the CEC patterns in a substrate sample (Isub) and the corresponding digestate (Idig) were compared (marked grey in Table S9†). The comparison of the sum-CEC concentrations indicates that the digestion process will result in an overall but minor reduction of the target CECs (substrate Isub: 25.8 μg L−1 to digestate 5I: 22.3 μg L−1) as summarised in Fig. 4. Fig. 4 shows the level comparison between the target CECs in a substrate and a digestate sample collected from an experimental reactor at station I. It is worth mentioning that the substrate sample Isub was not thermally treated, and no additives were added to the digestate sample Idig. However, some compounds were detected in the digestate but not found in the substrate, namely, 3-hydroxy carbamazepine (CAR-3OH), diclofenac (DCF) and metoprolol (MEP; see Fig. 4). Hence, the occurrence of these contaminants, which were not found in the substrate, indicates that cleavage of phase-II conjugates, present in the raw substrate (20% sludge from young fish, 80% manure), may occur during the digestion process. They also contribute to the final composition and concentrations of CECs and the transformation products in the resulting digestate. However, this could also reflect the significant temporal variation in the composition of the raw substrate loaded, even in the same biogas reactor.
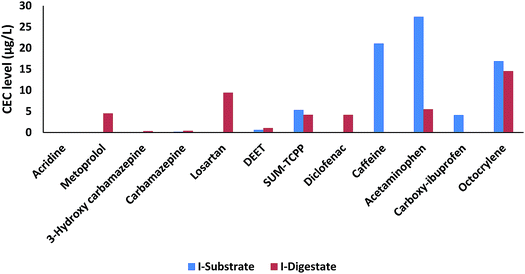 |
| Fig. 4 Concentration comparison between the target CECs in a substrate (blue – Isub) and a digestate sample (red – Idig) collected from an experimental reactor at station I. Please note these samples originate from the same production plant but are not produced during the same biogas process. | |
The role of conjugate cleavage for the remobilisation of previously immobilised contaminants in biologically active matrices has been reported previously for similar matrices to those studied here.60–65 There is apparent degradation for some compounds (e.g., ACE and OCR) but not for the majority of target substances (Fig. 4). This observed degradation may be attributed to the degradation of ACE and OCR in the resulting digestate after the anaerobic digestion as earlier reported.66,67
The levels of CAR are higher in solid digestates compared to the liquid samples, but the presence of the transformation product CAR-3OH was not confirmed in solid samples (Fig. 5). This different profile between liquid and solid digestates indicates partitioning of the compounds, for instance, the partitioning of CAR-3OH (more polar) into the liquid phase before separating the digestate into a liquid and a solid fraction.
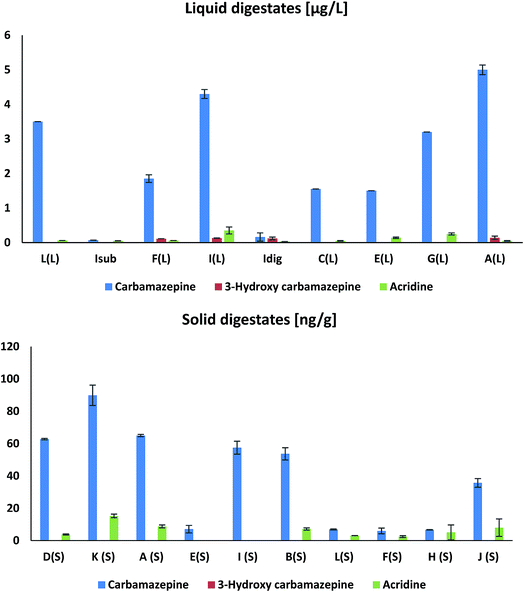 |
| Fig. 5 Levels of carbamazepine (CAR) and its transformation products acridine [ACR] and 3-hydroxy-carbamazepine [CAR-3OH] in liquid and solid digestates. | |
Contaminants of emerging concern in liquid digestates
The compounds with the highest concentrations in liquid digestates (Table S9†) were acetaminophen, caffeine, metoprolol, prednisolone, losartan, DEET, TCPP, ibuprofen, and octocrylene, which were found at levels from tens to hundreds of μg L−1. Acetaminophen, ibuprofen, prednisolone, losartan, and metoprolol are currently among the most widely used pharmaceuticals available without a prescription but also prescribed in Norway for medical treatment.68 Octocrylene is a commonly used UV-blocker and sunscreen ingredient. Therefore, the occurrence of these compounds (pharmaceuticals and sunscreen) in substrate and digestate samples is directly attributed to their wide application in cosmetics and medical treatment in Norwegian society. The stimulant caffeine, commonly used in beverages and refined food products, was found in most digestate samples at a maximum concentration of 10 μg L−1. Similar levels for some of these compounds have been reported in primary sludge samples during anaerobic digestion.69
Carbamazepine (CAR), used as an anticonvulsant in medical therapy, was detected in all liquid digestate samples with concentrations varying from 0.07 to 5.0 μg L−1. It has earlier been reported that CAR is unaffected by an aerobic digestion.57 However, as shown in Fig. 5, the CAR metabolites acridine and 3-hydroxy carbamazepine were found in the digestate samples, indicating effective transformation in the substrate or during biogas production (AD). Surprisingly, the CAR transformation product carbamazepine-10,11-epoxide, usually identified as the main CAR transformation product in waste water and other biologically active matrices,70–72 was not detected in the digestate samples investigated here.
Ibuprofen was detected in two liquid biogas digestates mainly derived from sewage sludge based substrates (E(L) and A(L)) at concentrations of 36 μg L−1 and 26.7 μg L−1, respectively. Carboxy-ibuprofen (ibuprofen transformation product) was detected in one liquid digestate at a <LOQ concentration level (F(L)). Formation of carboxylated and oxidized transformation products during the AD process is not considered a common process. However, their detection in the digestate samples may be attributed to their formation during substrate conditioning and treatment.
All other target PPCPs were found at average concentrations below and around 1–5 μg L−1. The levels and patterns of the CECs in liquid digestate reported here are found to be in agreement with earlier findings; for instance, carbamazepine, diclofenac, and metoprolol are reported to mainly partition into the aqueous phase.73,74
Contaminants of emerging concern in solid digestates
In solid digestates, higher CEC levels were found compared to those in liquid digestate samples. However, only 17 out of 24 target CECs were detected and quantified in solid digestates. The novel flame-retardant tris(chloroisopropyl) phosphate (TCPP), octocrylene (sunscreen ingredient), the angiotensin II-receptor antagonist losartan, and the β-blocker metoprolol are the predominant CECs found in solid samples. Atorvastatin was found in two cases at concentration levels above the uLOQ (samples I(S) & F(S); Fig. 2). Caffeine (frequently detected in liquid digestates) was found in concentrations of 30–210 ng g−1 wet weight (ww) in the solid digestates (3 out of 10 samples). DEET, acridine, carbamazepine, and metoprolol were quantified in both liquid and solid samples although the levels found in solid digestates were higher.
Perspectives
Biogas production is acknowledged as an important tool in terms of today's international efforts aimed at establishing sustainable circular bioeconomic structures in global economies. Many nations support this development in order to reduce the still increasing CO2 emissions on Earth. Circular strategies and improved sustainability imply that waste from production processes like biogas production is used as the basis for other production lines. To succeed, it is mandatory to assess the entire life cycle of these product waste chains in order to avoid any uncontrolled negative effects on society and the environment.
Our study shows that the many CEC residues that are present during biogas production are not retained or degraded during anaerobic digestion. In fact, most of the CECs in our study are found in potential substrate materials (Table S12†). As demonstrated earlier for similar recycling-based production processes, in order to reduce and minimize potential hazards and provide a safe platform for reuse of the resulting residues, a thorough monitoring and understanding of the production, with respect to potential anthropogenic pollutants, is required.75–80 We, therefore, recommend optimising biogas production processes also with respect to a minimal output of pollutants. This should be done, at least, when the digestate is further applied as a soil amendment or fertilizer.
The results of the current study revealed that the AD process in biogas plants does not efficiently eliminate organic micropollutants associated with substrates. Furthermore, the potential cleavage of undetected phase-II conjugates during substrate pretreatment and subsequent digestion may in some cases lead to increased CEC levels. Hence, in addition to appropriate selection and treatment of the biogas substrate, we recommend appropriate treatment of biogas digestates before their subsequent agricultural application.
According to the results obtained here, separation of digestates into liquid and solid fractions significantly enhances the partitioning of organic contaminants. Polar and ionized organic pollutants such as pharmaceuticals tend to partition into the liquid digestate. Thus, we recommend enhancing the separation process and even performing successive water extraction of the separated solid digestate.
The polar and ionized organic contaminants in the liquid fraction (digestate supernatants or diluted liquid digestate) can further be eliminated by physicochemical treatment such as UV photolysis and ultrasonication. Several pharmaceuticals undergo fast photodegradation even under solar irradiation.81 It is also recommended to enhance the recovery of phosphorus by precipitation using iron(II)-sulfate prior to digestate separation.82
As anaerobic processes are not very efficient in removing CECs from solid digestates, other technologies such as composting could be used for the solid digestate fractions.66
Conflicts of interest
There are no conflicts to declare.
Abbreviations
SI | Substrate food waste |
SII | Substrate sewage sludge |
SIII | Substrate fish silage |
SIV | Substrate manure |
RTemp | Reactor temperature |
RT | Retention time |
In | Inoculum added |
PTTemp | Pre-treatment high temperature |
PTTHP | Pre-treatment thermal hydrolysis processing |
PTno | No pre-treatment |
PreI | Precipitant FeCl3 |
PreII | Precipitant AlCl3 |
PreIII | Precipitant FeS |
PreIV | Precipitant EcoFloc90 |
PreV | Precipitant FeSO4 × 6H2O |
Pre0 | No precipitant |
% DM | % dry matter |
Poly | Polymer added |
Acknowledgements
The authors wish to thank the Research Council of Norway (RCN) for funding the project “Novel organic pollutants from recycling of organic waste as risk factors for human exposure (NovelPol)” (268214/E50). We thank Dr Ivan Dragicevic for fruitful discussions and help with the design of the study. The support of Drs John Morken, Jon Benskin (ACES, Stockholm University, SWE) and Pavel Cupr (RECETOX, Masaryk University, CZ) is highly appreciated. We extend our appreciation to all the Norwegian biogas production plants who contributed without hesitation to the study reported here. The authors are indebted to Dr Jens M. J. Nolsøe (KBM, NMBU) for reading the manuscript and for valuable comments.
References
- R. Andersson, Profits and Predation in the Human Bioeconomy, Publ. Cult., 2018, 30, 413–439 CrossRef.
- F. Bauer, Narratives of biorefinery innovation for the bioeconomy: Conflict, consensus or confusion?, Environmental Innovation and Societal Transitions, 2018, 28, 96–107 CrossRef.
- L. Biber-Freudenberger, A. K. Basukala, M. Bruckner and J. Borner, Sustainability Performance of National Bio-Economies, Sustainability, 2018, 10, 2705–2025 CrossRef.
- T. Ronzon and R. M'Barek, Socioeconomic Indicators to Monitor the EU's Bioeconomy in Transition, Sustainability, 2018, 10, 1745–1767 CrossRef.
- M. G. B. Lima, Toward Multipurpose Agriculture: Food, Fuels, Flex Crops, and Prospects for a Bioeconomy, Glob. Environ. Politics, 2018, 18, 143–150 CrossRef.
- D. Bernad-Beltran, A. Simo and M. D. Bovea, Attitude towards the incorporation of the selective collection of biowaste in a municipal solid waste management system. A case study, Waste Management, 2014, 34, 2434–2444 CrossRef CAS PubMed.
- S. Huttunen, K. Manninen and P. Leskinen, Combining biogas LCA reviews with stakeholder interviews to analyse life cycle impacts at a practical level, J. Cleaner Prod., 2014, 80, 5–16 CrossRef.
- J. H. Ebner, R. A. Labatut, J. S. Lodge, A. A. Williamson and T. A. Trabold, Anaerobic co-digestion of commercial food waste and dairy manure: Characterizing biochemical parameters and synergistic effects, Waste Management, 2016, 52, 286–294 CrossRef CAS PubMed.
- A. Veeken and B. Hamelers, Assessment of heavy metal removal technologies for biowaste by physico-chemical fractionation, Environ. Technol., 2003, 24, 329–337 CrossRef CAS PubMed.
- X. Song, W. Luo, F. I. Hai, W. E. Price, W. Guo, H. H. Ngo and L. D. Nghiem, Resource recovery from wastewater by anaerobic membrane bioreactors: Opportunities and challenges, Bioresour. Technol., 2018, 270, 669–677 CrossRef CAS PubMed.
- V. Stazi and M. C. Tomei, Enhancing anaerobic treatment of domestic wastewater: State of the art, innovative technologies and future perspectives, Sci. Total Environ., 2018, 635, 78–91 CrossRef CAS PubMed.
- Y. D. Chen, S. H. Ho, D. Nagarajan, N. Q. Ren and J. S. Chang, Waste biorefineries – integrating anaerobic digestion and microalgae cultivation for bioenergy production, Curr. Opin. Biotechnol., 2018, 50, 101–110 CrossRef CAS PubMed.
- J. C. Peinemann and D. Pleissner, Material Utilization of Organic Residues, Appl. Biochem. Biotechnol., 2018, 184, 733–745 CrossRef CAS PubMed.
- S. Dahiya, A. N. Kumar, J. Shanthi Sravan, S. Chatterjee, O. Sarkar and S. V. Mohan, Food waste biorefinery: Sustainable strategy for circular bioeconomy, Bioresour. Technol., 2018, 248, 2–12 CrossRef CAS PubMed.
- G. A. Iocoli, M. C. Zabaloy, G. Pasdevicelli and M. A. Gomez, Use of biogas digestates obtained by anaerobic digestion and co-digestion as fertilizers: Characterization, soil biological activity and growth dynamic of Lactuca sativa L, Sci. Total Environ., 2019, 647, 11–19 CrossRef CAS PubMed.
- C. Pedizzi, I. Noya, J. Sarli, S. Gonzalez-Garcia, J. M. Lema, M. T. Moreira and M. Carballa, Environmental assessment of alternative treatment schemes for energy and nutrient recovery from livestock manure, Waste Management, 2018, 77, 276–286 CrossRef CAS PubMed.
- C. Knoop, M. Tietze, C. Dornack and T. Raab, Fate of nutrients and heavy metals during two-stage digestion and aerobic post-treatment of municipal organic waste, Bioresour. Technol., 2018, 251, 238–248 CrossRef CAS PubMed.
- C. Wald, The new economy of excrement, Nature, 2017, 549, 146–148 CrossRef CAS PubMed.
- I. Dragicevic, S. Eich-Greatorex, T. A. Sogn, S. J. Horn and T. Krogstad, Use of high metal-containing biogas digestates in cereal production – Mobility of chromium and aluminium, J. Environ. Manage., 2018, 217, 12–22 CrossRef CAS PubMed.
- E. Bloem, A. Albihn, J. Elving, L. Hermann, L. Lehmann, M. Sarvi, T. Schaaf, J. Schick, E. Turtola and K. Ylivainio, Contamination of organic nutrient sources with potentially toxic elements, antibiotics and pathogen microorganisms in relation to P fertilizer potential and treatment options for the production of sustainable fertilizers: A review, Sci. Total Environ., 2017, 607–608, 225–242 CrossRef CAS PubMed.
- C. Baccot, V. Pallier and G. Feuillade-Cathalifaud, Biochemical methane potential of fractions of organic matter extracted from a municipal solid waste leachate: Impact of their hydrophobic character, Waste Management, 2017, 63, 257–266 CrossRef CAS PubMed.
- A. Pivato, S. Vanin, R. Raga, M. C. Lavagnolo, A. Barausse, A. Rieple, A. Laurent and R. Cossu, Use of digestate from a decentralized on-farm biogas plant as fertilizer in soils: An ecotoxicological study for future indicators in risk and life cycle assessment, Waste Management, 2016, 49, 378–389 CrossRef CAS PubMed.
-
T. Al Seadi, Good Practice in Quality Management of AD residues from biogas production: Task 24 og AEA Technology Environment, 2001 Search PubMed.
- B. Kelleher, J. J. Leahy, A. Henihan, T. O'dwyer, D. Sutton and M. Leahy, Advances in poultry litter disposal technology – a review, Bioresour. Technol., 2002, 83, 27–36 CrossRef CAS PubMed.
- Y. Chen, J. J. Cheng and K. S. Creamer, Inhibition of anaerobic digestion process: a review, Bioresour. Technol., 2008, 99, 4044–4064 CrossRef CAS PubMed.
-
T. Al Seadi, D. Rutz, H. Prassl, M. Köttner, T. Finsterwalder and S. Volk, More about anaerobic digestion (AD), ed. T. Al Seadi, Esbjerg: University of Southern Denmark, 2008 Search PubMed.
- L. Levén, K. Nyberg and A. Schnürer, Conversion of phenols during anaerobic digestion of organic solid waste – a review of important microorganisms and impact of temperature, J. Environ. Manage., 2012, 95, S99–S103 CrossRef PubMed.
- V. Arthurson, Closing the global energy and nutrient cycles through application of biogas residue to agricultural land – potential benefits and drawback, Energies, 2009, 2, 226–242 CrossRef CAS.
-
J. Holm-Nielsen, N. Halberg and S. Huntingford, Joint biogas plant: agricultural advantages: circulation of N, P and K, 1993 Search PubMed.
- M. H. Chantigny, D. A. Angers, P. Rochette, G. Bélanger, D. Massé and D. Côté, Gaseous nitrogen emissions and forage nitrogen uptake on soils fertilized with raw and treated swine manure, J. Environ. Qual., 2007, 36, 1864–1872 CrossRef CAS PubMed.
- F. Tambone, B. Scaglia, G. D'Imporzano, A. Schievano, V. Orzi, S. Salati and F. Adani, Assessing amendment and fertilizing properties of digestates from anaerobic digestion through a comparative study with digested sludge and compost, Chemosphere, 2010, 81, 577–583 CrossRef CAS PubMed.
-
B. Drosg, W. Fuchs, T. Al Seadi, M. Madsen and B. Linke, Nutrient recovery by biogas digestate processing, IEA Bioenergy, 2015, vol. 2015, p. 711 Search PubMed.
- I. Dragicevic, S. Eich-Greatorex, T. A. Sogn, R. Linjordet and T. Krogstad, Fate of copper, nickel and zinc after biogas digestate application to three different soil types, Environ. Sci. Pollut. Res. Int., 2017, 24, 13095–13106 CrossRef CAS PubMed.
-
A. Teodorita, R. Dominik, P. Heinz, K. Michael, F. Tobias, V. Silke and J. Rainer, Biogas handbook, University of Southern Denmark, Denmark, 2008 Search PubMed.
- R. C. Brändli, T. Kupper, T. D. Bucheli, M. Zennegg, S. Huber, D. Ortelli, J. Müller, C. Schaffner, S. Iozza and P. Schmid, Organic pollutants in compost and digestate. Part 2. Polychlorinated dibenzo-p-dioxins, and-furans, dioxin-like polychlorinated biphenyls, brominated flame retardants, perfluorinated alkyl substances, pesticides, and other compounds, J. Environ. Monit., 2007, 9, 465–472 RSC.
- M. Engwall and A. Schnürer, Fate of Ah-receptor agonists in organic household waste during anaerobic degradation—estimation of levels using EROD induction in organ cultures of chick embryo livers, Sci. Total Environ., 2002, 297, 105–108 CrossRef CAS PubMed.
-
H. Olsman, A. Schnürer, H. Björnfoth, B. van Bavel and M. Engwall, Fractionation and determination of Ah receptor (AhR) agonists in organic waste after anaerobic biodegradation and in batch experiments with PCB and decaBDE, 2005 Search PubMed.
- I. Angelidaki, A. S. Mogensen and B. K. Ahring, Degradation of organic contaminants found in organic waste, Biodegradation, 2000, 11, 377–383 CrossRef CAS PubMed.
- R. C. Brändli, T. D. Bucheli, T. Kupper, J. Mayer, F. X. Stadelmann and J. Tarradellas, Fate of PCBs, PAHs and their source characteristic ratios during composting and digestion of source-separated organic waste in full-scale plants, Environ. Pollut., 2007, 148, 520–528 CrossRef PubMed.
-
M.-L. Nilsson, Occurrence and fate of organic contaminants in wastes, 2000 Search PubMed.
- L. De Gelder, K. Audenaert, B. Willems, K. Schelfhout, S. De Saeger and M. De Boevre, Processing of mycotoxin contaminated waste streams through anaerobic digestion, Waste Management, 2018, 71, 122–128 CrossRef CAS PubMed.
-
T. Al Seadi, B. Drosg, W. Fuchs, D. Rutz and R. Janssen, in The biogas handbook, Elsevier, 2013, pp. 267–301 Search PubMed.
- A. M. Ali, H. T. Ronning, L. K. Sydnes, W. M. Alarif, R. Kallenborn and S. S. Al-Lihaibi, Detection of PPCPs in marine organisms from contaminated coastal waters of the Saudi Red Sea, Sci. Total Environ., 2018, 621, 654–662 CrossRef CAS PubMed.
- A. M. Ali, H. T. Ronning, W. Alarif, R. Kallenborn and S. S. Al-Lihaibi, Occurrence of pharmaceuticals and personal care products in effluent-dominated Saudi Arabian coastal waters of the Red Sea, Chemosphere, 2017, 175, 505–513 CrossRef CAS PubMed.
- K. S. Jewell, A. Wick and T. A. Ternes, Comparisons between abiotic nitration and biotransformation reactions of phenolic micropollutants in activated sludge, Water Res., 2014, 48, 478–489 CrossRef CAS PubMed.
- J. Hu, H. Chang, L. Wang, S. Wu, B. Shao, J. Zhou and Y. Zhao, Detection, occurrence and fate of indirubin in municipal sewage treatment plants, Environ. Sci. Technol., 2008, 42, 8339–8344 CrossRef CAS PubMed.
- M. Leclercq, O. Mathieu, E. Gomez, C. Casellas, H. Fenet and D. Hillaire-Buys, Presence and fate of carbamazepine, oxcarbazepine, and seven of their metabolites at wastewater treatment plants, Arch. Environ. Contam. Toxicol., 2009, 56, 408–415 CrossRef CAS PubMed.
- P. Sukul and M. Spiteller, Fluoroquinolone antibiotics in the environment, Rev. Environ. Contam. Toxicol., 2007, 191, 131–162 CrossRef CAS.
- P. Dwivedi, X. Zhou, T. G. Powell, A. M. Calafat and X. Ye, Impact of enzymatic hydrolysis on the quantification of total urinary concentrations of chemical biomarkers, Chemosphere, 2018, 199, 256–262 CrossRef CAS PubMed.
- G. S. Doran, A. K. Smith, J. T. Rothwell and S. H. Edwards, Direct detection of glucuronide metabolites of lidocaine in sheep urine, J. Chromatogr. B: Anal. Technol. Biomed. Life Sci., 2018, 1076, 84–90 CrossRef CAS PubMed.
- Y. Li, C. Gu, J. Gruenhagen, P. Yehl, N. P. Chetwyn and C. D. Medley, An enzymatic deconjugation method for the analysis of small molecule active drugs on antibody-drug conjugates, mAbs, 2016, 8, 698–705 CrossRef CAS PubMed.
- Y. Suzuki, A. Kubota, T. Furukawa, K. Sugamoto, Y. Asano, H. Takahashi, T. Sekito, Y. Dote and Y. Sugimoto, Residual of 17beta-estradiol in digestion liquid generated from a biogas plant using livestock waste, J. Hazard. Mater., 2009, 165, 677–682 CrossRef CAS PubMed.
- C. Starkenmann, Y. Niclass, M. Troccaz and A. J. Clark, Identification of the precursor of (S)-3-methyl-3-sulfanylhexan-1-ol, the sulfury malodour of human axilla sweat, Chem. Biodiversity, 2005, 2, 705–716 CrossRef CAS PubMed.
- Y. Xue, H. Liu, S. Chen, N. Dichtl, X. Dai and N. Li, Effects of thermal hydrolysis on organic matter solubilization and anaerobic digestion of high solid sludge, Chem. Eng. J., 2015, 264, 174–180 CrossRef CAS.
- M. Narumiya, N. Nakada, N. Yamashita and H. Tanaka, Phase distribution and removal of pharmaceuticals and personal care products during anaerobic sludge digestion, J. Hazard. Mater., 2013, 260, 305–312 CrossRef CAS PubMed.
- S. Yang, F. I. Hai, W. E. Price, J. McDonald, S. J. Khan and L. D. Nghiem, Occurrence of trace organic contaminants in wastewater sludge and their removals by anaerobic digestion, Bioresour. Technol., 2016, 210, 153–159 CrossRef CAS PubMed.
- J. Malmborg and J. Magnér, Pharmaceutical residues in sewage sludge: effect of sanitization and anaerobic digestion, J. Environ. Manage., 2015, 153, 1–10 CrossRef CAS PubMed.
- M. Carballa, F. Omil, T. Ternes and J. M. Lema, Fate of pharmaceutical and personal care products (PPCPs) during anaerobic digestion of sewage sludge, Water Res., 2007, 41, 2139–2150 CrossRef CAS PubMed.
- J. Laurent, M. Casellas, H. Carrere and C. Dagot, Effects of thermal hydrolysis on activated sludge solubilization, surface properties and heavy metals biosorption, Chem. Eng. J., 2011, 166, 841–849 CrossRef CAS.
- W. Ben, B. Zhu, X. Yuan, Y. Zhang, M. Yang and Z. Qiang, Occurrence, removal and risk of organic micropollutants in wastewater treatment plants across China: Comparison of wastewater treatment processes, Water Res., 2018, 130, 38–46 CrossRef CAS PubMed.
- W. Ben, B. Zhu, X. Yuan, Y. Zhang, M. Yang and Z. Qiang, Transformation and fate of natural estrogens and their conjugates in wastewater treatment plants: Influence of operational parameters and removal pathways, Water Res., 2017, 124, 244–250 CrossRef CAS PubMed.
- A. Jelic, S. Rodriguez-Mozaz, D. Barcelo and O. Gutierrez, Impact of in-sewer transformation on 43 pharmaceuticals in a pressurized sewer under anaerobic conditions, Water Res., 2015, 68, 98–108 CrossRef CAS PubMed.
- A. Nieto, F. Borrull, E. Pocurull and R. M. Marce, Occurrence of pharmaceuticals and hormones in sewage sludge, Environ. Toxicol. Chem., 2010, 29, 1484–1489 CrossRef CAS PubMed.
- M. Pedrouzo, F. Borrull, E. Pocurull and R. M. Marce, Estrogens and their conjugates: Determination in water samples by solid-phase extraction and liquid chromatography-tandem mass spectrometry, Talanta, 2009, 78, 1327–1331 CrossRef CAS PubMed.
- M. P. Schlusener and K. Bester, Determination of steroid hormones, hormone conjugates and macrolide antibiotics in influents and effluents of sewage treatment plants utilising high-performance liquid chromatography/tandem mass spectrometry with electrospray and atmospheric pressure chemical ionisation, Rapid Commun. Mass Spectrom., 2005, 19, 3269–3278 CrossRef PubMed.
- M. Biel-Maeso, C. Corada-Fernández and P. A. Lara-Martín, Removal of personal care products (PCPs) in wastewater and sludge treatment and their occurrence in receiving soils, Water Res., 2019, 150, 129–139 CrossRef CAS PubMed.
- S. E. Musson, P. Campo, T. Tolaymat, M. Suidan and T. G. Townsend, Assessment of the anaerobic degradation of six active pharmaceutical ingredients, Sci. Total Environ., 2010, 408, 2068–2074 CrossRef CAS PubMed.
-
S. Sakshaug, H. Strøm, C. Berg, H. S. Bli, I. Litleskare and T. Granum, Legemiddelforbruket i Norge 2013–2017 [Drug Consumption in Norway 2013–2017], Report 978-82-8082-916-0, Folkehelseinstituttet, Oslo, 2018 Search PubMed.
- H. V. Phan, R. Wickham, S. Xie, J. A. McDonald, S. J. Khan, H. H. Ngo, W. Guo and L. D. Nghiem, The fate of trace organic contaminants during anaerobic digestion of primary sludge: A pilot scale study, Bioresour. Technol., 2018, 256, 384–390 CrossRef CAS PubMed.
- K. I. Ekpeghere, W. J. Sim, H. J. Lee and J. E. Oh, Occurrence and distribution of carbamazepine, nicotine, estrogenic compounds, and their transformation products in wastewater from various treatment plants and the aquatic environment, Sci. Total Environ., 2018, 640–641, 1015–1023 CrossRef CAS PubMed.
- C. vom Eyser, A. Borgers, J. Richard, E. Dopp, N. Janzen, K. Bester and J. Tuerk, Chemical and toxicological evaluation of transformation products during advanced oxidation processes, Water Sci. Technol., 2013, 68, 1976–1983 CrossRef CAS PubMed.
- Y. Yu, Q. Huang, Z. Wang, K. Zhang, C. Tang, J. Cui, J. Feng and X. Peng, Occurrence and behavior of pharmaceuticals, steroid hormones, and endocrine-disrupting personal care products in wastewater and the recipient river water of the Pearl River Delta, South China, J. Environ. Monit., 2011, 13, 871–878 RSC.
- M. Hörsing, A. Ledin, R. Grabic, J. Fick, M. Tysklind, J. la Cour Jansen and H. R. Andersen, Determination of sorption of seventy-five pharmaceuticals in sewage sludge, Water Res., 2011, 45, 4470–4482 CrossRef PubMed.
- A. Jelic, M. Gros, A. Ginebreda, R. Cespedes-Sánchez, F. Ventura, M. Petrovic and D. Barcelo, Occurrence, partition and removal of pharmaceuticals in sewage water and sludge during wastewater treatment, Water Res., 2011, 45, 1165–1176 CrossRef CAS PubMed.
- M. Allinson, K. Kadokami, F. Shiraishi, D. Nakajima, J. Zhang, A. Knight, S. R. Gray, P. J. Scales and G. Allinson, Wastewater recycling in Antarctica: Performance assessment of an advanced water treatment plant in removing trace organic chemicals, J. Environ. Manage., 2018, 224, 122–129 CrossRef CAS PubMed.
- H. A. Leslie, P. E. G. Leonards, S. H. Brandsma, J. de Boer and N. Jonkers, Propelling plastics into the circular economy – weeding out the toxics first, Environ. Int., 2016, 94, 230–234 CrossRef CAS PubMed.
- E. Govasmark, J. Stab, B. Holen, D. Hoornstra, T. Nesbakk and M. Salkinoja-Salonen, Chemical and microbiological hazards associated with recycling of anaerobic digested residue intended for agricultural use, Waste Management, 2011, 31, 2577–2583 CrossRef CAS PubMed.
- L. Morselli, J. Luzi, C. De Robertis, I. Vassura, V. Carrillo and F. Passarini, Assessment and comparison of the environmental performances of a regional incinerator network, Waste Management, 2007, 27, S85–S91 CrossRef PubMed.
- G. B. Kester, R. B. Brobst, A. Carpenter, R. L. Chaney, A. B. Rubin, R. A. Schoof and D. S. Taylor, Risk characterization, assessment, and management of organic pollutants in beneficially used residual products, J. Environ. Qual., 2005, 34, 80–90 CAS.
- M. Asari, H. Takatsuki, M. Yamazaki, T. Azuma, H. Takigami and S. Sakai, Waste wood recycling as animal bedding and development of bio-monitoring tool using the CALUX assay, Environ. Int., 2004, 30, 639–649 CrossRef CAS PubMed.
- A. M. M. Ali, R. Kallenborn, L. K. Sydnes, H. T. Rønning, W. M. Alarif and S. Al-Lihaibi, Photolysis of pharmaceuticals and personal care products in the marine environment under simulated sunlight conditions: irradiation and identification, Environ. Sci. Pollut. Res., 2017, 24, 14657–14668 CrossRef CAS PubMed.
- L. Fang, J.-s. Li, M. Z. Guo, C. Cheeseman, D. C. Tsang, S. Donatello and C. S. Poon, Phosphorus recovery and leaching of trace elements from incinerated sewage sludge ash (ISSA), Chemosphere, 2018, 193, 278–287 CrossRef CAS PubMed.
Footnote |
† Electronic supplementary information (ESI) available. See DOI: 10.1039/c9em00175a |
|
This journal is © The Royal Society of Chemistry 2019 |
Click here to see how this site uses Cookies. View our privacy policy here.