DOI:
10.1039/C8SC01657G
(Edge Article)
Chem. Sci., 2018,
9, 5850-5854
Unusual biaryl torsional strain promotes reactivity in Cu-catalyzed Sommelet–Hauser rearrangement†
Received
11th April 2018
, Accepted 13th June 2018
First published on 14th June 2018
Abstract
A Cu-catalyzed Sommelet–Hauser dearomatization of dihydrophenanthridine and diazo compounds is reported for the synthesis of spiro-indolines. A spiro-structure with adjacent quaternary and tertiary carbon centers was constructed in one step as a single isomer. Increasing steric hindrance by introducing ortho-substituents dramatically improved substrate reactivity in this transformation.
Introduction
Dearomatization is a robust method for the construction of polyfunctionalized cyclic compounds; it can generate quaternary carbon centers and multi-substituted C
C double bonds in one or two steps. Due to the high stabilization of aromaticity, dearomatization reactions are traditionally performed under harsh conditions, such as oxidation in the presence of hypervalent iodine,1 Birch reduction,2 enzymatic conditions,3 or other conditions.4 Dearomatization has recently been achieved using photochemistry and transition metal catalysis. In particular, catalytic asymmetric dearomatization (CADA) has attracted substantial attention, such as from the You group, for constructing optically pure molecules from arenes.5
The Sommelet–Hauser reaction is an intramolecular rearrangement of benzyl ammonium salts to give ortho-substituted benzylamines (Scheme 1a, path A).6–8 Sommelet–Hauser dearomatization can efficiently generate valuable three-dimensional compounds bearing quaternary carbon centers if the two ortho positions have non-hydrogen substituents (Scheme 1b). In a variation known as thio-Sommelet rearrangement, dearomatized compounds bearing thioether functionalities can be prepared by replacing the ammonium ylides with sulfur ylides.9,10
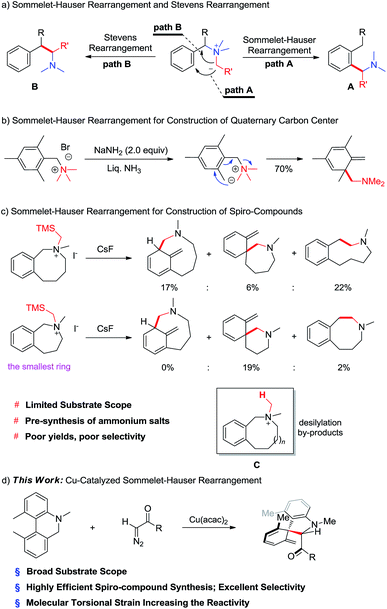 |
| Scheme 1 The Sommelet–Hauser rearrangement. | |
A challenge to exploiting the Sommelet–Hauser reaction is preventing competitive Stevens rearrangement,11,12 in which formal intramolecular nucleophilic attack at the benzylic position affords 2-phenylethanamine analogues (Scheme 1a, path B). Singleton has argued that these two types of rearrangements compete dynamically because they can occur via the same transition state.13
Sommelet–Hauser rearrangement can be used to produce spiro-compounds from benzocyclic ammonium salts, but the reported reactions usually give low yields and wide product distribution.14 For example, the reaction usually produces two regioisomers together with ring-enlarged products from Stevens rearrangement (Scheme 1c). Ring strain in seven- and eight-membered structures lowers the rearrangement rate, significantly increasing the yields of desilylation by-products C. In addition, the requirement for pre-synthesized ammonium salts considerably limits the substrate scope of the reaction. Third, the Sommelet–Hauser rearrangement appears incompatible with ammonium salts with fewer than seven members. As a result, the reaction cannot generate rings smaller than six-membered N-heterocycles (Scheme 1c).
Herein we report the copper-catalyzed generation of nitrogen ylide intermediates capable of Sommelet–Hauser rearrangement, in which N-containing six-membered rings efficiently participate. We further report that molecular torsional strain in biaryl substrates increases their reactivity in this rearrangement.
We wondered whether we could adapt dearomatization via Sommelet–Hauser rearrangement to generate the important spirocyclohexaneindoline structural motif, found in several natural products including gelsemine,15 21-oxogelsemine16 and koumine (Fig. 1).17 It also comprises the core structural unit of various biologically active molecules.18
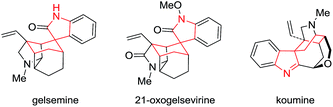 |
| Fig. 1 Natural products containing a spirocyclohexaneindoline skeleton. | |
Results and discussion
Optimization
We were unable to dearomatize biaryl compound 1a using diazo compound 2a in the presence of silver, rhodium or gold salts as catalysts, which are frequently used in reactions involving carbene (Table 1, entries 1–3). We were pleased to find that 1a reacted with 2a in the presence of Cu(OTf) 0.5PhH at 90 °C to give 27% of spiro product 3a as a single isomer, along with a small amount of 4a (entry 4). The reaction generated a spiro-structure with adjacent quaternary and tertiary carbon centers in one step. Screening of copper salts showed Cu(acac)2 to perform the best in terms of yield and reaction time (entries 5–9). Lowering the reaction temperature increased the selectivity for 3a over the Stevens rearrangement product 4a, improving the isolated yield of 3a to 72% (entry 10). Solvent screening identified methyl tert-butyl ether (MTBE) as the best (entries 11–13). The reaction proceeded smoothly with only 5 mol% of Cu(acac)2, albeit with a slightly lower yield (entry 14).
Table 1 Optimization of reaction conditionsa
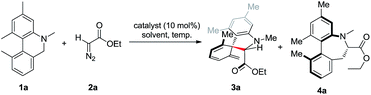
|
Entry |
Catalyst |
Solvent |
T/°C |
Yield of 3a/% |
Yield of 4a/% |
Unless stated otherwise, the reaction was conducted with 1a (0.10 mmol), 2a (0.20 mmol), and a metal catalyst (10 mol%).
An unidentified product was formed.
|
1 |
Rh2(OAc)4 (5 mol%) |
Toluene |
90 |
<5 |
— |
2 |
(Ph3P)AuCl |
Toluene |
90 |
–b |
— |
3 |
AgOTf |
Toluene |
90 |
NR |
— |
4 |
Cu(OTf) 0.5PhH |
Toluene |
90 |
27 |
<5 |
5 |
Cu(OTf)2 |
Toluene |
90 |
NR |
— |
6 |
Cu(MeCN)4PF6 |
Toluene |
90 |
32 |
<5 |
7 |
CuTC |
Toluene |
90 |
47 |
<5 |
8 |
Cu(acac)2 |
Toluene |
90 |
63 |
<5 |
9 |
Cu(hfacac)2 |
Toluene |
90 |
<5 |
— |
10 |
Cu(acac)2 |
Toluene |
50 |
72 |
— |
11 |
Cu(acac)2 |
MTBE |
50 |
83 |
— |
12 |
Cu(acac)2 |
DCE |
50 |
53 |
— |
13 |
Cu(acac)2 |
THF |
50 |
72 |
— |
14 |
Cu(acac)2 (5 mol%) |
MTBE |
50 |
79 |
— |
Substrate scope
With the optimal conditions in hand, we tested the generality of this Cu(acac)2-catalyzed Sommelet–Hauser rearrangement. Diazo compounds with different ester groups, such as methyl, tert-butyl and substituted benzyl esters, reacted smoothly (3b–f). A diazo substrate with an electron-withdrawing group (CF3) led to a slightly lower yield (3e). The reaction tolerated additional methyl groups, a fluorine atom and methoxyl groups in the biaryl aniline skeleton (3g–3j, 3t). Naphthalene-based substrates and a binaphthalene derivative reacted uneventfully to give the spiro compounds in decent to excellent yields (3k–3r, 3t–3z and 3bb). Stereoinduction did not occur with the (R)-1-phenylethanol-derived diazo compound, such that a pair of diastereoisomers was isolated in a 1
:
1 ratio (3t). This rearrangement was compatible with ketones and amides, which afforded the desired products in decent to good yields (3u–3x). Introducing a methyl group adjacent to the C–N bond completely inhibited this transformation (3cc). The reaction tolerated an aryl-Br bond. Scale-up also proved possible, with compound 3x isolated in 69% yield on the 1.0 mmol scale.
The two ortho-substituents (R1 and R2 in Table 2) strongly influenced the efficiency of the Sommelet–Hauser reaction and selectivity for this type of rearrangement over Stevens rearrangement (Scheme 2). For example, under the standard conditions at 50–90 °C, the conversions of 5 were less than 55%, while isolated yields of 6 were no more than 35%. Product 6 was much less stable during purification than products 3a–3bb. Performing the reaction at higher temperature in toluene gave Stevens rearrangement product 7 as the major product, with 5 recovered at 55% yield.
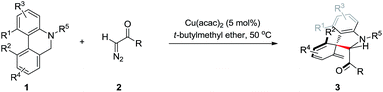
|
The reaction was conducted with 1 (0.10 mmol), diazo compound 2 (0.20 mmol), and Cu(acac)2 (0.005 mmol, 5 mol%) in MTBE at 50 °C.
The reactions were performed in toluene.
|
|
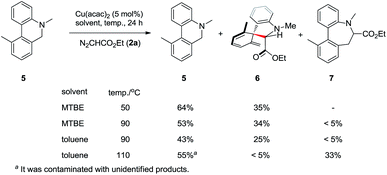 |
| Scheme 2 Substituent effects. | |
Synthetic applications
Preliminary synthetic studies were performed to demonstrate the utility of this rearrangement. Under 1 atm H2, the diene compound 3k underwent chemoselective 1,4-hydrogenation in the presence of Pd/C to give 8k in 83% yield, whose structure was unambiguously confirmed by single-crystal X-ray diffraction analysis. The N-methyl group could be further modified via rhodium-catalyzed C–H functionalization to afford 9k. Finally, the ester functionality could be reduced to the corresponding alcohol using DIBAL-H at −78 °C without eliminating the diene moiety. The diene was further cyclized in the presence of the MeNHOH·HCl catalyst to yield polycyclic compound 10k in 57% yield (Scheme 3).
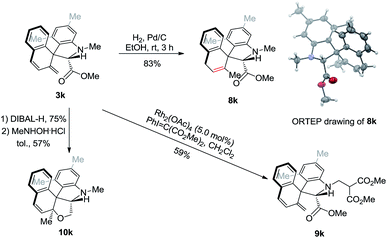 |
| Scheme 3 Control experiment and synthetic applications. | |
Mechanistic studies
In a plausible catalytic cycle (Scheme 4), the reaction begins when copper salt interacts with the diazo compound to give copper–carbene complex D, releasing one molecule of nitrogen gas. The subsequent reaction of the copper–carbene complex with amine 1 affords the ylide E. Intramolecular nucleophilic attack in E delivers the spiro product 3 either before or after dissociation of the copper catalyst. To obtain structural information about ammonium salt E and explain the different reactivity between 1 and 5, two ammonium compounds F and G were synthesized and analyzed by single-crystal X-ray diffraction. The torsional angle (C5–C6/C7–C13) was around 41° in F and 29° in G.19 Steric repulsion between the two ortho-substituents increased the distortion in the biaryl structure. We reasoned that the two ortho-substituents in E should distort its structure to a similar extent as in F. As a result, the relatively high-energy intermediate E undergoes a rapid intramolecular nucleophilic reaction to deliver spiro compounds. Alternatively, the increased torsion reduces the delocalization of the aniline lone-pair, which increases the basicity and accelerates the ammonium formation. Substituents on the substrates strongly influence the ratio of the [2,3]-rearrangement product to the Stevens rearrangement product;13 the latter rearrangement usually proceeds via a bi-radical pathway under thermal conditions.20 These considerations imply that less distorted substrates such as 5 generate lower-energy ylide intermediates, which therefore must overcome a higher activation barrier to give spiro products. Consistent with this, compound 5 was associated with lower conversion rates under the similar conditions.
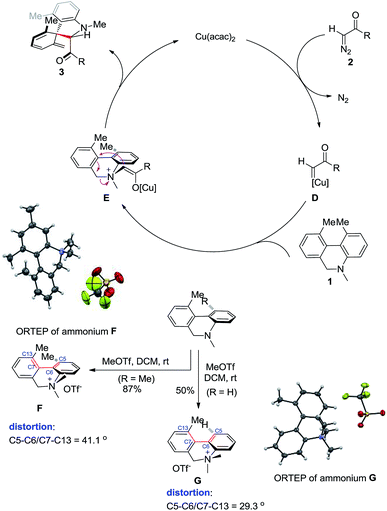 |
| Scheme 4 Plausible mechanism. | |
Conclusions
We have reported a copper-catalyzed Sommelet–Hauser rearrangement to construct spiroindoline compounds bearing adjacent quaternary and tertiary carbon centers. Increasing the torsional strain in the biaryl substrate increases its reactivity in this transformation. This is, to our knowledge, the first report in which Sommelet–Hauser rearrangement was used to generate spiro compounds bearing five-membered N-heterocycles.
Conflicts of interest
There are no conflicts to declare.
Acknowledgements
This work was supported by the NSFC (21622206, 21472179), ‘973’ project from the MOST of China (2015CB856600), the Strategic Priority Research Program of the Chinese Academy of Sciences (XDB20000000), and the Fundamental Research Funds for the Central Universities (WK2060190086).
Notes and references
-
(a) A. Yoshimuar and V. V. Zhdankin, Chem. Rev., 2016, 116, 3328 CrossRef PubMed;
(b) R. D. Richardson and T. Wirth, Angew. Chem., Int. Ed., 2006, 45, 4402 CrossRef PubMed;
(c) M. Uyanik and K. Ishihara, ChemCatChem, 2012, 4, 177 CrossRef;
(d) T. Dohi and Y. Kita, Chem. Commun., 2009, 2073 RSC.
- P. W. Rabideau and Z. Marcinow, Org. React., 1992, 42, 1–334 Search PubMed.
-
(a) M. Sono, M. P. Roach, E. D. Coulter and J. H. Dawson, Chem. Rev., 1996, 96, 2841 CrossRef PubMed;
(b) R. G. Harvey, Acc. Chem. Res., 1981, 14, 218 CrossRef;
(c) T. Hudlicky, D. Gonzalez and D. T. Gibson, Aldrichimica Acta, 1999, 32, 35 Search PubMed;
(d) F. L. Ortiz, M. J. Iglesias, I. Fernández, C. M. A. Sánchez and G. R. Gómez, Chem. Rev., 2007, 107, 1580 CrossRef PubMed.
- For some typical examples:
(a) J. Barluenga, A. A. Trabanco, J. Flórez, S. García-Granda and E. Martín, J. Am. Chem. Soc., 1996, 118, 13099 CrossRef;
(b) J. García-Fortanet, F. Kessler and S. L. Buchwald, J. Am. Chem. Soc., 2009, 131, 6676 CrossRef PubMed;
(c) C. Shen, R.-R. Liu, R.-J. Fan, Y.-L. Li, T.-F. Xu, J.-R. Gao and Y.-X. Jia, J. Am. Chem. Soc., 2015, 137, 4936 CrossRef PubMed;
(d) J. Nan, Z. Zuo, L. Luo, L. Bai, H. Zheng, Y. Yuan, J. Liu, X. Luan and Y. Wang, J. Am. Chem. Soc., 2013, 135, 17306 CrossRef PubMed;
(e) A. Seoane, N. Casanova, N. Quiñones, J. L. Mascareñas and M. Gulías, J. Am. Chem. Soc., 2014, 136, 7607 CrossRef PubMed;
(f) F. C. Pigge, J. J. Coniglio and R. Dalvi, J. Am. Chem. Soc., 2006, 128, 3498 CrossRef PubMed;
(g) L. Bai, Y. Yuan, J. Liu, J. Wu, L. Han, H. Wang, Y. Wang and X. Luan, Angew. Chem., Int. Ed., 2016, 55, 6946 CrossRef PubMed;
(h) Z. Zuo, H. Wang, L. Fan, J. Liu, Y. Wang and X. Luan, Angew. Chem., Int. Ed., 2017, 56, 2767 CrossRef PubMed.
-
(a)
S. You and S.-L. You, Asymmetric Dearomatization Reactions, Wiley-VCH, 2016 CrossRef;
(b) C. Zheng and S.-L. You, Chem, 2016, 1, 830 CrossRef;
(c) C.-X. Zhuo, C. Zheng and S.-L. You, Acc. Chem. Res., 2014, 47, 2558 CrossRef PubMed.
-
(a) M. Sommelet, C. R. Hebd. Seances Acad. Sci., 1937, 205, 56–58 Search PubMed;
(b) S. W. Kantor and C. R. Hauser, J. Am. Chem. Soc., 1951, 73, 4122–4131 CrossRef.
- E. Tayama, Chem. Rec., 2015, 789–800 CrossRef PubMed.
-
(a) E. Tayama, R. Sato, K. Takedachi, H. Iwamoto and E. Hasegawa, Tetrahedron, 2012, 68, 4710 CrossRef;
(b) E. Tayama and H. Kimura, Angew. Chem., Int. Ed., 2007, 46, 8869 CrossRef PubMed;
(c) E. Tayama, K. Orihara and H. Kimura, Org. Biomol. Chem., 2008, 6, 3673 RSC;
(d) E. Tayama, K. Watanabe and Y. Matano, Eur. J. Org. Chem., 2016, 3631 CrossRef;
(e) E. Tayama, K. Watanabe and S. Sotome, Org. Biomol. Chem., 2017, 15, 6668–6678 RSC.
-
(a) T.-J. Lee and W. J. Holtz, Tetrahedron Lett., 1983, 24, 2071 CrossRef;
(b) R. Berger, J. W. Ziller and D. L. Van Vranken, J. Am. Chem. Soc., 1998, 120, 841 CrossRef;
(c) Y. Li, Y. Shi, Z. Huang, X. Wu, P. Xu, J. Wang and Y. Zhang, Org. Lett., 2011, 13, 1210 CrossRef PubMed.
- For a reaction involving Sommelet-Hauser rearrangement: P. G. Gassman, T. J. van Bergen, D. P. Gilbert and B. W. Jr Cue, J. Am. Chem. Soc., 1974, 96, 5495–5517 CrossRef.
-
(a) A. Jończyk and D. Lipiak, J. Org. Chem., 1991, 56, 6933–6937 CrossRef;
(b) A. Jończyk, D. Lipiak and K. Sienkiewicz, Synlett, 1991, 493–496 CrossRef;
(c) T. Zdrojewski and A. Jonczyk, J. Org. Chem., 1998, 63, 452 CrossRef PubMed;
(d) S. Harthong, R. Bach, C. Besnard, L. Guénée and J. Lacour, Synthesis, 2013, 45, 2070 CrossRef.
- For some related reactions:
(a) Z. Zhang, Z. Sheng, W. Yu, G. Wu, R. Zhang, W.-D. Chu, Y. Zhang and J. Wang, Nat. Chem., 2017, 9, 970–976 CrossRef PubMed;
(b) A. Soheili and U. K. Tambar, J. Am. Chem. Soc., 2011, 133, 12956 CrossRef PubMed;
(c) C.-Y. Zhou, W.-Y. Yu, P. W. H. Chan and C.-M. Che, J. Org. Chem., 2004, 69, 7072 CrossRef PubMed;
(d) M. S. Holzwarth, I. Alt and B. Plietker, Angew. Chem., Int. Ed., 2012, 51, 5351–5354 CrossRef PubMed.
- B. Biswas, S. C. Collins and D. A. Singleton, J. Am. Chem. Soc., 2014, 136, 3740–3743 CrossRef PubMed.
- K. Narita, N. Shirai and Y. Sato, J. Org. Chem., 1997, 62, 2544 CrossRef PubMed.
-
(a) F. L. Sonnenschein, Ber. Dtsch. Chem. Ges., 1876, 9, 1182–1186 CrossRef;
(b) H. Conroy and J. K. Chakrabarti, Tetrahedron Lett., 1959, 1, 6–13 CrossRef;
(c) Y. Schun and G. A. Cordell, J. Nat. Prod., 1985, 48, 969–971 CrossRef PubMed;
(d) F. M. Lovell, R. Pepinsky and A. J. C. Wilson, Tetrahedron Lett., 1959, 1, 1–5 CrossRef.
- Y. Schun, G. A. Cordell and M. Garland, J. Nat. Prod., 1986, 49, 483–487 CrossRef.
- Y.-K. Xu, L. Yang, S.-G. Liao, P. Cao, B. Wu, H.-B. Hu, J. Guo and P. Zhang, J. Nat. Prod., 2015, 78, 1511 CrossRef PubMed.
-
(a) A. Fensome, M. Koko, J. Wrobel, P. Zhang, Z. Zhang, J. Cohen, S. Lundeen, K. Rudnick, Y. Zhu and R. Winneker, Bioorg. Med. Chem. Lett., 2003, 13, 1317 CrossRef PubMed;
(b) H. Venkatesan, M. C. Davis, Y. Altas, J. P. Snyder and D. C. Liotta, J. Org. Chem., 2001, 66, 3653 CrossRef PubMed.
- K. Zhao, L. Duan, S. Xu, J. Jiang, Y. Fu and Z. Gu, Chem, 2018, 4, 599–612 Search PubMed.
-
(a) W. D. Ollis, M. Rey and I. O. Sutherland, J. Chem. Soc., Chem. Commun., 1975, 543 RSC;
(b) Y. Maeda and Y. Sato, J. Chem. Soc., Perkin Trans. 1, 1997, 1491 RSC;
(c) G. Ghigo, S. Cagnina, A. Maranzana and G. Tonachini, J. Org. Chem., 2010, 75, 3608 CrossRef PubMed.
|
This journal is © The Royal Society of Chemistry 2018 |