The effect of peer-led team learning on undergraduate engineering students’ conceptual understanding, state anxiety, and social anxiety†
Received
20th October 2017
, Accepted 28th March 2018
First published on 29th March 2018
Abstract
This study aims to compare the effectiveness of a Peer-Led Team Learning (PLTL) model with that of traditional college instruction (TCI) in enhancing the conceptual understanding and reducing both the state anxiety and social anxiety of undergraduate engineering students in a general chemistry course in a quasi-experimental design. 128 engineering students taking the course participated in the study. One of the course sections was randomly assigned to the experimental group and the other section was assigned to the control group. Both sections were taught by the same instructor. The control group was instructed using traditional college instruction, while the experimental group was instructed using the PLTL model. Throughout this study, six peer-led chemistry workshops and leader training sessions were performed simultaneously. The General Chemistry Concept Test, the State–Trait Anxiety Inventory, and the Social Anxiety Questionnaire for Adults were administered before and after the treatment to both groups. One-way Multivariate Analysis of Covariance (MANCOVA) indicated that after controlling students’ university entrance scores, trait anxiety scores and pre-test scores of both the General Chemistry Concept Test and state anxiety, the PLTL model was more effective in improving the conceptual understanding and reducing the situational anxiety of engineering students in undergraduate general chemistry. However, it was not so effective in lessening their social anxiety when compared to traditional college instruction.
Introduction
Despite rapid advances in technology, the number of students choosing science, technology, engineering, and mathematics (STEM) and related careers has decreased progressively with time, while the rates of students’ retention in these fields have remained steady or decreased (Higher Education Research Institute, 2010). A high rate of attrition or dropouts of students in post-secondary and tertiary education is another significant problem, and the reasons are attributed to having low levels of students’ success and effort in their learning (e.g., Lovitts, 2001), having differences in the learning approach (e.g., Tobias, 1990), having problems related to pedagogy, student assessment, curriculum design and guidance (e.g., Seymour and Hewitt, 1997), and having problems in basic competencies – creative problem-solving skills, study skills, complex communication skills, self-development and critical thinking skills (e.g., Gafney and Varma-Nelson, 2008). Various methods such as summer programs, active-learning techniques, and structured research experiences for undergraduates have been recommended by institutions to remedy those problems (Drane et al., 2014). According to Tinto (1975), one way of doing this is by improving the instruction in a way that students feel a sense of belonging to an academic society. Tobias (1992) also stresses the crucial role of mentoring in students’ careers. Besides, “the most powerful source of influence on growth and development during the undergraduate years” was reported as the employment of students’ peer groups (Astin, 1993, p. 398), which is not included in traditional instruction.
Peer-led team learning (PLTL)
Peer-Led Team Learning (PLTL) is one of the instructional models which have been implemented by numerous faculties and institutions in STEM courses to overcome such problems. It creates an active learning environment where students construct their own knowledge, communicate more effectively with one another, review the lecture material, have opportunity to ask questions and think more deeply about the conceptual side of their learning using higher-level reasoning and problem solving skills (Tien et al., 2002; Varma-Nelson and Coppola, 2005; Varma-Nelson, 2006). It was first introduced in general chemistry as “Workshop Chemistry” supported by the National Science Foundation (NSF) for systemic change in chemistry in the early 1990s and this was followed by many institutions across STEM disciplines (Gafney and Varma-Nelson, 2008). In PLTL, lectures are first presented by course instructors and then these lectures are supplemented with PLTL workshops in which a successful undergraduate student guides a team of students in weekly meetings to solve problems developed by the course instructor. In a typical workshop session, a group of 6–8 students meet with their peer leader for one or two hours per week to discuss the topics and solve the problems related to the course content by providing conceptual understanding and critical thinking (Gafney and Varma-Nelson, 2008). A PLTL workshop is different from a conventional recitation session since its core activity is discussion and debate on the concepts and ideas that form the basis for solving the problems. Unlike a teacher in the traditional classroom or a teaching assistant in demonstrating how to solve the problem, a peer leader is a facilitator or guide who earned at least a B grade in the same course in the previous years. The role of the peer leader is to engage the group in problem-solving activities, assist students in developing conceptual understanding, support students to reach their full potential and facilitate discussion of scientific concepts and ideas (Roth et al., 2001a, 2001b; Gafney and Varma-Nelson, 2008).
As a second-generation pedagogy, PLTL is the combination of well-established theories and methods for instructional design such as group learning, reciprocal teaching, studio instruction, and social constructivism (Varma-Nelson et al., 2004; Varma-Nelson and Coppola, 2005). Group learning, reciprocal teaching, and studio instruction have their theoretical base in social constructivism in which students build their knowledge from an interaction between the previously learned concept and new knowledge and skills by discussing and criticizing the ideas in a social and collaborative environment (Phillips, 2000; Gredler, 2009). Vygotsky, as a social constructivist, advocates that community plays a fundamental role in the process of making meaning and development of cognition because cognitive skills and thinking patterns are determined in the social and cultural contexts of the learning environment (1978). Two significant ideas in the work of Vygotsky consist of the roots of PLTL. First, the interaction with a more knowledgeable and skilled peer or teacher is an effective way of developing skills and strategies. In other words, knowledge is scaffolded with the support of a more experienced and successful learner. Second, tasks and activities should be designed for students in order to enhance their level of performance. Vygotsky proposed the concept of the Zone of Proximal Development (ZPD), which is the difference between students’ independent problem-solving capabilities (lower end of the ZPD) and their potential development (higher end of the ZPD) with the guidance of more knowledgeable peers. When training peer-leaders, as well as designing and selecting appropriate instructional materials, these ideas are key concepts because students cannot solve challenging problems easily on their own, but they can accomplish by the interaction with the members of the team and peer leaders within their ZPD in chemistry workshops (Cracolice, 2000; Varma-Nelson and Coppola, 2005; Gafney and Varma-Nelson, 2007).
Many studies about the original PLTL model and its several variants have been conducted in many different STEM areas. Wilson and Varma-Nelson (2016) summarized research studies in terms of five types of PLTL variants: (1) peer-led guided inquiry (PLGI); (2) online PLTL; (3) PLTL in the chemistry laboratory; (4) utilization of in-class peer leaders; and (5) increased student-to-peer-leader ratios. Since the intervention of this study was performed in a general chemistry course with standard PLTL, previous studies were examined and analyzed in this regard; see Table 1, which briefly summarizes research studies in General Chemistry courses. As can be seen from the table, PLTL has been generally designed by either comparisons across semesters or comparisons in concurrent sections of a course to improve students’ achievement, course pass rate, retention, self-confidence, engagement, and attitude towards both the subject and the course in the First-Semester General Chemistry course (Gosser and Roth, 1998; Stewart et al., 2007; Hockings et al., 2008; Lyon and Lagowski, 2008; Kampmeier and Varma-Nelson, 2009; Lewis, 2011; Shields et al., 2012; Drane et al., 2014; Chan and Bauer, 2015) and in the Second-Semester General Chemistry course (Alger and Bahi, 2004; Báez-Galib et al., 2005; Mitchell et al., 2012).
Table 1 PLTL studies implemented in general chemistry courses
Author/year |
Participants/institution |
Duration |
Course |
Intervention |
Group/period |
Dependent variables |
Major findings |
Note: ACS: American Chemical Society, PLTL: peer-led team learning, TI: traditional instruction, NR: not reported, ER: extended recitation, RR: regular recitation. |
Alger and Bahi (2004)
|
Students at Southern Utah University |
Fall and spring semesters of 2001–2002 and 2002–2003 academic years |
First- and Second-Semester General Chemistry |
During 2002–2003 |
✓ 6 to 8 students per group |
✓ Midterm exams |
✓ PLTL group had higher means and medians of scores on mid-term examinations than TI |
|
|
|
|
✓ PLTL vs. RC with computer-aided instruction |
✓ 2 h a week |
✓ ACS final exams |
✓ Both groups had the same means and medians of scores on the ACS final exams during the fall semester, while the PLTL group had higher scores during the spring semester |
|
|
|
|
During 2001–2002 |
|
|
|
|
|
|
|
✓ PLTL vs. TI |
|
|
|
|
Báez-Galib et al. (2005)
|
A total of 1849 students from chemistry, biology, mathematics, physics, and education programs at the University of Puerto Rico |
Successive seven semesters from 1997 to 2000 |
First- and Second-Semester General Chemistry |
✓ PLTL: Chem-2-Chem Program (C2C) |
✓ 3 h a week |
✓ Successful and unsuccessful course outcomes |
✓ For both successful (final grades of A, B, and C) and unsuccessful (final grades of F and W) course outcomes, there were highly statistically significant differences among groups, favoring the C2C program |
|
|
|
|
✓ TI: non-participants |
|
✓ Student perception of C2C |
✓ C2C participants had a low withdrawal rate |
|
|
|
|
|
|
|
✓ C2C participants perceived the program in a positive way |
|
Chan and Bauer (2015)
|
A majority of student majoring in engineering, sciences, health and human services, and liberal arts at the University of New Hampshire |
Fully-randomized design for two falls of 2008 and 2009 |
First-Semester General Chemistry |
Fully-randomized |
✓ 4–9 students per group |
✓ Exam achievement |
For fully-randomized study |
|
|
Quasi-experimental design in falls of 2004, 2005, 2006, 2007, 2011, 2012, and 2013 |
|
✓ PLTL |
Fully randomized design |
✓ Affective characteristics (self-concept and attitude towards chemistry) |
✓ There was no significant difference in achievement, attitude, or self-concept between PLTL participants and non-PLTL participants |
|
|
|
|
✓ Non-PLTL (one or more of the following options) |
✓ Nearly every week, 80 min in 2008 and 50 min in 2009 |
|
✓ First-year students had higher positive attitude, self-concept, and achievement than others |
|
|
|
|
• Self-organized study groups 45% |
Quasi-experimental design |
|
✓ Male students showed higher positive attitude and self-concept than female students |
|
|
|
|
• Instructor-led review sessions |
✓ 50 min in 2009 and 2011 while 80 min in other years |
|
For quasi-experimental study |
|
|
|
|
• Drop-in tutorials led by graduate or undergraduate chemistry majors |
|
|
✓ PLTL participants showed stronger exam achievement than non-PLTL participants |
|
|
|
|
• Instructor office hours |
|
|
|
|
|
|
|
Quasi-experimental |
|
|
|
|
|
|
|
✓ PLTL |
|
|
|
|
|
|
|
✓ TI |
|
|
|
|
Drane et al. (2014)
|
All students enrolled in seven courses from five STEM disciplines at Northwestern University |
Over a 10 year period between fall 2001 and spring 2011 |
Biology 210, Introductory Chem 101, Organic Chem, Physics 130, Physics 135, Calculus, and Engineering |
✓ PLTL: Gateway Science Workshop (GSW) |
✓ 5 to 7 students per group |
✓ Course grades |
✓ Participants benefited more from GSW than TI in Chemistry regardless of their gender or ethnicity |
|
|
|
|
✓ TI: non-participants |
✓ 2 h a week |
✓ Course retention |
✓ GSW participants had a statistically significant higher retention rate in Chemistry than non-PLTL participants, favoring minority and female participants |
|
Hockings et al. (2008)
|
1125 students from Arts and Sciences, Engineering, Business, Art, or Architecture faculty at Washington University |
The fall semesters of 2003 and 2004 |
First-Semester General Chemistry |
✓ PLTL groups |
✓ 8 students per group |
✓ Student performance (grade) |
✓ PLTL participants performed higher and had a higher retention rate than non-PLTL participants |
|
|
|
|
✓ Non-PLTL groups |
✓ 2 h a week |
✓ PLTL students’ attitude and self-confidence |
✓ PLTL participants had positive attitudes toward PLTL and toward chemistry |
|
|
|
|
|
|
|
✓ Students expecting a higher grade tended to view PLTL more positively |
|
|
|
|
|
|
|
✓ Females felt more afraid than males about working in groups and females used more visuals than males to better understand a concept |
|
Lewis (2011)
|
29 general chemistry classes in a large undergraduate institution located in the Southeastern United States |
NR |
First-Semester General Chemistry |
✓ PLTL instruction |
|
✓ Retention |
✓ PLTL participants had a statistically significant higher retention rate than non-PLTL participants |
|
|
|
|
✓ TI |
✓ 50 min per week |
✓ ACS exam performance |
✓ Both groups showed equivalent performance on the ACS final exam |
|
|
|
|
|
✓ 12 to 16 students per group; 4 students per subgroup |
|
✓ Males and females had similar gains in passing the course |
|
|
|
|
|
|
|
✓ Minority PLTL students had the largest gain in pass rates |
|
Lyon and Lagowski (2008)
|
348 students at the University of Texas at Austin |
Spring of 2000 |
First-Semester General Chemistry |
✓ PLTL groups |
✓ 4 to 5 students per group |
✓ Course achievement |
✓ PLTL group had significantly higher performance than TI for the four lecture-oriented, hour-long examination |
|
|
|
|
✓ Non-PLTL groups |
|
|
✓ PLTL participants received higher course grades |
|
Mitchell et al. (2012)
|
55 classes of GC1 and GC2 in an institution located in the Southeast United States |
Fall and spring semesters of 2009–2010 and 2010–2011 academic years |
First (GC1) and Second-Semester (GC2) General Chemistry |
Case 1: GC1: PLTL, GC2: PLTL |
✓ 50 min per week |
✓ GC1 pass rate |
✓ The pass GC1 and GC2 rates were higher for PLTL classes than TI |
|
|
|
|
Case 2: GC1: PLTL, GC2: TI |
✓ 12 to 16 students per leader |
✓ Students’ decision to enroll in GC2 (attrition) |
✓ PLTL had no observable impact on the enroll GC2 rate |
|
|
|
|
Case 3: GC1: TI, GC2: PLTL |
|
✓ GC2 pass rate |
✓ PLTL participants in GC1 had a lower pass rate in GC2 than TI participants in GC1, but not statistically significant |
|
|
|
|
Case 4: GC1: TI, GC2: TI |
|
|
|
|
Shields et al. (2012)
|
426 underprepared students who predicted end-of-course (EOC) scores of 67 or below |
Fall semesters of 2007, 2008, and 2009 |
First-Semester General Chemistry |
Comparison groups: |
✓ 90 min ER |
✓ Course performance |
✓ Group2 participants showed significantly the highest course performance, while group4 participants performed significantly lower among the four groups. |
|
|
|
|
✓ Group1 (ER and PLTL) |
✓ 2 h PLTL workshops |
✓ Student perception |
✓ Group1 participants performed better than group3 participants but not statistically significant |
|
|
|
|
✓ Group2 (ER, PLTL and peer mentoring) |
✓ 2 h peer mentoring and 4 to 5 students per group |
|
✓ Extended-length recitations and peer-mentoring improved students’ chemistry performance |
|
|
|
|
✓ Group3 (RR and PLTL) |
|
|
|
|
|
|
|
✓ Group4 (RR only) |
|
|
|
|
Stewart et al. (2007)
|
A majority of students at the University of Maine |
Fall semesters from 1997 through 2003 |
First-Semester General Chemistry |
✓ PLTL |
✓ 2 h a week |
✓ Grade |
✓ PLTL enhanced student grades and retention rates |
|
|
|
|
✓ TI: no PLTL |
|
✓ PLTL attendance |
✓ PLTL attendance significantly improved grades |
|
|
|
|
|
|
✓ Success rates (%ABC) |
✓ %ABC grades increased with time |
Conceptual understanding
Holme et al. (2015) defined the conceptual understanding in general chemistry as “applying core chemistry ideas (theories, patterns, relationships, etc.) to chemical situations; reasoning about these core chemistry ideas using higher order skills; expanding situational knowledge to predict and/or explain behaviour of chemical systems, demonstrating the critical thing and reasoning in solving problem; translating across scales and representations (p. 1480)”. Numerous studies have revealed that since course instructors generally have not sustained an environment for students to develop conceptual understanding of scientific concepts, students have some alternative conceptions of these concepts that are in conflict with the consensus of the scientific community (Treagust, 1986; Driver et al., 1994; Duit and Treagust, 2003; Pabuccu and Geban, 2006; Duit et al., 2008). Through team learning, students are able to discuss and negotiate scientific principles, ideas and meaning with peers via critical thinking, and problem-solving skills to construct their knowledge and understanding of science concepts in a social context (Varma-Nelson and Coppola, 2005). Therefore, in line with Bramaje and Espinosa (2013) too, PLTL is expected to enhance students’ conceptual understanding more than traditional ways.
State and social anxiety
In addition to the above-mentioned contributions of PLTL, it promises to affect students’ state anxiety and social anxiety. State anxiety refers to the temporary emotional state or feelings caused by a particularly stressful situation at a particular period of time or at a particular moment in time, while trait anxiety refers to the permanent and general feeling of anxiety related to the personality characteristic (Spielberger et al., 1983). Additionally, social anxiety can be defined as an intense fear of embarrassment or humiliation in social and performance situations (American Psychiatric Association, 2000). In the science learning process, some degree of anxiety may be helpful for an individual to perform effectively; however, a high level of anxiety may hinder students’ academic performance by blocking utilization and attention resources, or developing more cognitive interference from worries and fears (Mallow and Greenburg, 1982).
Moreover, a growing body of research on the role of anxiety emphasizes that anxiety, one of the affective factors, is likely to influence students’ achievement and performance in science, and thus, a high level of anxiety can lead to low science achievement and academic performance (Czerniak and Chiarelott, 1984; Vitasari et al., 2010; Sridevi, 2013). Gafney and Varma-Nelson (2008) conducted focus group studies about the reasons why students are so unwilling to ask questions in the classroom. Two reported causes of students’ failing to ask questions in lecture are fear, anxiety, and stress regarding speaking in a large group and concern about making comments and asking questions that would be perceived as stupid by the professor and their peers. In contrast, they can easily ask questions in workshops because leaders and peers are more supportive and accessible. PLTL can alleviate some of the anxiety that students feel in the lecture hall due to public speaking and inadequate knowledge. According to Johnson and Johnson (1994), small group work increases students’ critical thinking abilities, academic achievement, and self-esteem as well as develops social skills. Since studying in a group environment is able to foster social skills and interaction as well as improve confidence and self-esteem, it may reduce anxiety about social situations.
Significance and purpose of the study
Although there is increasing attention to numerous college-level initiatives and reforms to improve science education and remedy problems in colleges and universities in the USA, such reforms were not much implemented to foster more active learning and reduce concerns in higher education of the country where the study is conducted. The influence of this model on students was not investigated yet for this context. For this reason, the study offers a solution to the deficiencies, problems, or obstacles in post-secondary education and serves as an example in this area to implement and disseminate the PLTL model for other universities. As can be seen from Table 1, it is explicit that only a small number of studies have been conducted to examine the conceptual understanding of students (Alger and Bahi, 2004; Lewis, 2011) and its impact on affective factors (Hockings et al., 2008; Chan and Bauer, 2015). Instead, the majority of them aim to investigate its effect on students’ pass rate, course performance, and retention. Accordingly, it is worth investigating whether and how PLTL influences undergraduate engineering students’ conceptual understanding, state anxiety, and social anxiety in general chemistry courses. Based on these purposes, the following research question is investigated:
Is there a significant mean difference between the groups exposed to Peer-Led Team Learning (PLTL) instruction and Traditional College Instruction (TCI) in terms of undergraduate engineering students’ conceptual understanding, state anxiety, and social anxiety in general chemistry courses after controlling some extraneous variables?
Methodology
Research design
A quasi-experimental non-equivalent pre-test/post-test control group design was used to compare the effect of peer-led team learning with that of traditional instruction on improving students’ conceptual understanding and alleviating students’ state and social anxiety. In this design, although the groups being compared are randomly assigned as control and experimental, the subjects are not randomly assigned to these groups; instead already formed groups or intact groups are used (Fraenkel and Wallen, 2006).
In the current study, participants were enrolled in two sections of the general chemistry course. Since the course sections were already formed at the beginning of the semester, students could not be assigned to experimental or control groups so course sections were randomly assigned as an experimental group (EG) and a control group (CG) by a random procedure. Section numbers were written on a piece of paper and put into a box and then a number was drawn and assigned to the control group and the other number was assigned to the experimental group. Students in the experimental group were engaged in PLTL workshops, but those in the control group received no PLTL workshops; in contrast, they took traditional instruction only. Before and after the treatment each group was tested. Table 2 briefly describes the design of the study.
Table 2 Research design of the study
Group |
Pre-test |
Treatment |
Post-test |
Note. EG: experimental group, CG: control group, GCCT: General Chemistry Concept Test, STAI: State–Trait Anxiety Inventory, and SAQ: Social Anxiety Questionnaire for Adults. |
EG |
GCCT |
Peer-led team learning (PLTL) |
GCCT |
STAI |
STAI |
SAQ |
SAQ |
|
CG |
GCCT |
Traditional college instruction (TCI) |
GCCT |
STAI |
STAI |
SAQ |
SAQ |
Internal validity can be affected by subject characteristics, mortality, location, instrumentation, testing, history, and maturation, as well as the attitude of the subjects, regression, and implementation (Fraenkel and Wallen, 2006). Some of these threats to internal validity can be controlled with the design of the study such as subject characteristics, mortality, testing, history, maturation, and regression because groups’ general characteristics and other information about mortality and history were examined after random assignment of the groups. For example, there were some missing subjects during the data collection of pre-tests; however, the number of students who were lost was about the same in both groups. Fraenkel and Wallen (2006) state that in studies comparing groups if the loss of subjects in all groups is very close to each other, mortality is most likely not to be a big issue. Besides, there may be a problem resulting from the location but the nature of PLTL is group work and the location of the regular university classroom is not suitable for that collaborative work. On the other hand, the location in which data were collected was the same for both groups. In this kind of study, a researcher's beliefs or expectations can also cause him or her to unconsciously influence the participants; however, we observe only the processes and interfere with only the organizational arrangements during the workshop time. After all, leaders played the key role in providing communication. In addition, researchers observed some lecture hours and whole workshops. Throughout the implementation of the study, both groups had the same professor in lecture hours so there is no teaching ability difference. As a result, leaders and the instructor showed no overt bias in favor of PLTL over TCI or vice versa.
Setting and participants
All undergraduate engineering students have taken General Chemistry as a must course at the University where the medium of instruction is English. CEAC 105 General Chemistry is a one-semester course which combines the concepts of first and second-semester General Chemistry courses. This course is instructed in three 50 minute lecture sessions per week and three 50 minute laboratory sessions biweekly. In the fall semester of the 2016–2017 education year, two intact sections of the CEAC 105 General Chemistry course were selected. The instructor had about 20 years of experience in teaching this course and she was a volunteer for participating in the study. For the experimental group, PLTL was a combination of two thirds teacher-centered lecture interspersed with one third chemistry workshop including student–peer discussion and problem-solving. Thus, it was a course requirement that all students participate in the peer-led chemistry workshops. On the other hand, for the control group, the traditional instruction style was a teacher-centered lecture at weekly three 50 minute periods. The two comparison groups in this study were determined as illustrated in Fig. 1.
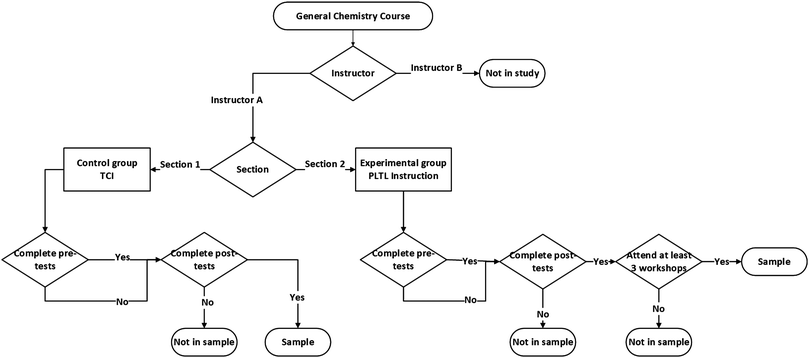 |
| Fig. 1 Process by which students were included in the sample. Note:participants who did not complete the pre-tests were retained in the sample after missing data analysis was conducted. | |
Course enrollments for experimental group and control group sections were 181 and 174, respectively, in that semester. If the students in section 1 completed both the pre-tests and post-tests or only the post-tests, they were taken as the sample of the control group. If the students in section 2 completed both the pre-tests and post-tests or only the post-tests as well as attended at least 50% of the PLTL sessions, they were taken as the sample of the experimental group. Students who did not complete these conditions were dropped out of the analysis. All demographic data in terms of students’ age, gender, major, and achievement level based on university entrance scores (LYS scores) were checked with the help of the registrar's office and compared between participants of the study and non-participants and between the experimental group and the control group to avoid bias among them. The majority of the students were 19 years old for both participants (P) and non-participants (NP) of the study. The gender percentages were also very close for participants (78% male students and 22% female students) and non-participants (79% male students and 21% female students). Both had students from six different major areas (27% of P and 14% of NP in electrical and electronics engineering, 3% of P and 14% of NP in manufacturing engineering, 17% of P and 22% of NP in mechatronics engineering, 33% of P and 22% of NP in civil engineering, 5% of P and 11% of NP in automotive engineering, and 15% of P and 17% of NP in software engineering).
Although some of the percentages for both groups were not close to each other, the university entrance scores (LYS scores) for each major varied between 220 and 400. The LYS mean scores, which were 295.37 for participants and 287.93 for non-participants of the study, are not different from each other (SDP = 37.37, SDNP = 33.14; t(353) = 1.77, p = 0.084). As a result, it was seen that they were similar in such characteristics.
Moreover, the subjects of the study consisted of 128 undergraduate engineering students with different majors (electrical and electronics engineering, manufacturing engineering, and mechatronics engineering for the control group, and civil engineering, automotive engineering, and software engineering for the experimental group). Of them, 28 were females and 100 were males. 60 (47 males and 13 females) of the participants were in CGs and 68 (53 males and 15 females) of them were in EGs. The age range of the students was between 18 and 27 years, generally centering on 19 years.
In addition, 14 students were chosen in this study as peer leaders from individuals who had attended the CEAC 105 General Chemistry course previously and completed it successfully. Purposive sampling was used to select peer leaders because peer leaders should have some requirements and characteristics for the proper implementation of PLTL chemistry workshops such as successful students as well as higher level skills about leadership, communication, and group work. All participants in this study signed the voluntary participation and acceptance form.
Data collection
In order to assess students’ conceptual understanding and anxiety levels in many different circumstances, three instruments were used as pre-tests and post-tests: the General Chemistry Concept Test (GCCT), the State–Trait Anxiety Inventory (STAI), and the Social Anxiety Questionnaire for Adults (SAQ). The details of these instruments are explained below.
General Chemistry Concept Test (GCCT).
The GCCT was developed by the authors to assess students’ conceptual understanding in the general chemistry course. During the developmental process of the test, questions in chemistry textbooks, master's theses, dissertations, journal articles (Mulford and Robinson, 2002; Uzuntiryaki, 2003; Bozkoyun, 2004; Pabuccu, 2004; Pabuccu and Geban, 2006; Yalcınkaya, 2010; Kaya, 2011; Al-Balushi et al., 2012; Ayyıldız and Tarhan, 2012; Brown et al., 2013), and high-stakes tests such as ACS Chemistry Olympiad Exams (American Chemical Society [ACS], 2016) and Advanced Placement (AP) Chemistry Exams (College Board, 2016) were analyzed. One question from an ACS Olympiad local section exam (ACS, 2014) and four questions from sample multiple choice questions of AP Chemistry (College Board, 2014) were used with their copyright notice. Then, items were constructed and examined by three chemistry educators with regard to content validity and format. After all revisions, 30 multiple choice items were identified and some of them were paired. In these cases, the first pair represents a chemical or physical effect, while the second pair expresses the reason for the observed effect in the first pair. The incorrect reasons in the second pair usually include students’ alternate conceptions or difficulties related to specific content area gathered from the literature.
This draft version of the test was piloted with 67 university students who major in chemistry education and engineering before the treatment. Item analysis was conducted to check reliability, item difficulty and item discrimination with the SPSS 24.0 program. The mean item difficulty was 0.51, which means a medium level as supported by Crocker and Algina (2008). Furthermore, the mean item discrimination index was 0.31, which is an acceptable value based on the estimated minimum critical value (Crocker and Algina, 2008). Additionally, items whose biserial correlation indices were below the minimum critical value were revised. The Cronbach alpha reliability of the test scores was found to be 0.799, which indicates high internal consistency (Pallant, 2011).
The final version with 30 multiple choice items was administered to both groups as a pre-test and post-test. The administration of the test took approximately 40–45 minutes. The correct answer was scored as 1 and an incorrect one was scored 0 for each question even if it was a pair question (Mulford and Robinson, 2002). Thus, a student can get a maximum of 30 as a total score. The sample items are indicated in the Appendix.
State–Trait Anxiety Inventory (STAI).
In this study, the last version of the STAI which was revised by Spielberger et al. (1983) was implemented to measure anxiety. The inventory consists of 2 subscales: the state anxiety scale and the trait anxiety scale. It has a total of 40 items with a 4-point Likert-type scale: items 1–20 measure situational or state anxiety (STAI-S) and items 21–40 measure trait anxiety (STAI-T). Each subscale requires respondents to rate the degree of various self-statements. In the state anxiety subscale the items were assessed how a respondent feels “at this moment” or “right now”, while in the trait anxiety subscale the items were assessed how a respondent “generally” feel. Some items in the scale are “I feel secure”, “I feel nervous”, “I wish I could be as happy as others seem to be”, and “Some unimportant thoughts run through my mind and bothers me”. After reversing scores for positively-worded items, a total score that a student got from each scale was a minimum of 20 and a maximum of 80. The high score shows a high level of anxiety.
Spielberger (1972) reported test–retest reliability with high school and college student samples. For the trait anxiety scale the internal consistency reliability coefficient ranged from 0.65 to 0.86, while for the state anxiety scale the internal consistency reliability coefficients ranged from 0.16 to 0.62. He also specified that, at the time of testing, situational factors may influence the level of state anxiety of respondents so it causes great changes in the reliability of the state anxiety scale. In other words, it is normal to have a low level of stability in reliability. In the current study, the Cronbach alpha reliabilities for the state anxiety scale, the trait anxiety scale, and the STAI scale were found to be 0.915, 0.909 and 0.948, respectively, which indicate high reliability according to Pallant (2011).
In the present study, the STAI State scale was used to assess the level of stress that was present at the beginning and to determine whether students’ anxiety was successfully decreased after the intervention. Since it is generally difficult to change trait anxiety, which refers to a permanent personality characteristic, the STAI Trait scale was used to specify the level of students’ general anxiety at the outset of the term and was adjusted as a covariate in the study.
Social Anxiety Questionnaire for Adults (SAQ).
This scale was developed in different item formats (i.e., 512-item, 118-item, and 82-item versions) by Caballo et al. (2010a). Then, it was revised to its 30-item format with a 5-point Likert type scale (known as SAQ-A30) by Caballo et al. (2010b) to measure university students’ uneasiness, stress, or nervousness level in social situations. SAQ consists of five factors and each has six items: speaking in public/talking with people in authority, interactions with the opposite sex, assertive expression of annoyance, disgust, or displeasure, criticism, and embarrassment, as well as interactions with strangers. As examples, “Maintaining a conversation with someone I’ve just met” and “Being asked a question in a class by the teacher or by a superior in a meeting” are the social situations in the scale. A student can get a maximum of 30 from each dimension and a maximum of 150 from the whole questionnaire. The high score on the scale represents that the anxiety level is high.
Caballo et al. (2010b) reported Cronbach's alpha and the split-half reliability coefficient for the whole SAQ to be 0.91, and 0.93, respectively. For the current study, Cronbach's alpha reliability was found to be 0.923 and the split-half reliability coefficient was found to be 0.898. The SAQ-A30 scale was used in the present study to assess undergraduate students’ level of social stress that was present at the outset of the term and to determine whether such anxiety was successfully decreased at the end of the term after the intervention.
Treatments
Three months before the implementation, a meeting was held with the course instructor, the head of the department, and the vice rector of the University and brief information about the model was given. Then, ethical permission from the applied ethics research center was taken. The workshop materials for the PLTL model were prepared by researchers taking the course curriculum into account. Some revisions were done to these materials by the course professor. Then, the PLTL leaders were selected and began training regarding how they could help their team members to solve challenging team problems in workshops. The experimental group students attended biweekly chemistry workshops. In the weeks when workshops were not held students attended three lecture periods. At the end of each workshop, 10 minute quizzes were implemented. In order to make the treatment less novel for the control group, the workshop questions were presented to the control group via the learning management system in their course. During the workshop, the course instructor did not attend in order not to cause any anxiety in students and leaders and to affect the group dynamics. The researchers joined the workshops as non-participant observers and leader training sessions as supervisors and learning specialists.
Treatment in the experimental group: PLTL instruction
For an effective PLTL instruction, the processes before, during, and after the implementation were crucial for the study. Gafney's theoretical framework for implementing and evaluating the PLTL program (2001a, 2001b, 2001c) with six essential principles was employed. These components were faculty involvement, integral to the course, leader selection and training, appropriate materials, appropriate organizational arrangements and administrative support. When PLTL has been properly implemented based on these components, it offers a more positive gain in student engagement, attitude, satisfaction, motivation, and academic performance (Varma-Nelson et al., 2004; Gafney and Varma-Nelson, 2008). Therefore, these critical components were taken into account in these processes and explained briefly.
Departmental and institutional support.
To implement the model, the first thing was to get approval from the administration of the institution and the head of the department. After taking their support, a search was conducted on how we could have inspired leaders to take part in this study. The university had a program called “Sharing the Success”, which aimed to empower successful students to share their success by working in an academic or administrative unit within the university. The role of these students was to assist the academic staff in the academic units, train their peers, serve as a model for other students and work on various projects in administrative units. Students having a minimum CGPA of 2.50 over 4.00 could apply to the “Sharing the Success” programme. Each student who successfully completed the program by working at least 40 hours would be given certificates of participation at the end of the academic term. Consequently, peer leaders, who completed both the workshops and their training sessions successfully throughout the semester, were awarded a Sharing the Success Program Certificate by the administration of the university at the end of the semester.
Integration of workshops to the course.
Chemistry workshop hours should be seen as important as other components of the course (lecture, laboratory); therefore, it was required all students attend fully and attendance was taken by the team leaders in each workshop. The workshops were coordinated with the content of the corresponding week. Quizzes were added to assess students' individual progress in the chemistry concept and used to provide regular attendance at the workshops. The course instructor underlined the importance of the workshops in lecture time and encouraged students to attend the workshops.
Course professor involvement.
The general chemistry course professor was in cooperation throughout the study especially in the preparation of workshop materials and selection and training of peer leaders. For example, she examined the materials, gave feedback and helped in revising the materials to the last version because the content of workshop materials should be overlapped with the course content and objectives. In the leader selection process, she helped to reach the sophomore students taking the course already and provided support to the leaders in their training.
Workshop materials.
The content of the course and the time needed for them presented in the course syllabus were taken into account by constructing the workshop materials. As indicated in Table 3, selected topics of general chemistry were identified and the course textbook was examined for these issues in order to write the learning objectives for each topic. In this direction, a booklet was prepared for use in the chemistry workshop. Within each unit, the subject and subheadings of the subject and the corresponding learning outcomes were presented. The workshop problems were prepared based on the fact that problems should be enforcing the students at the appropriate level in a challenging way and encourage active and collaborative learning at the same time (Strozak and Wedegaertner, 2001). Many resources were utilized to construct problems such as course textbooks and chemistry books, PLTL guidebooks, materials presented in dissertations, ACS and AP Chemistry exam questions, etc. Besides, their theoretical framework was established according to the concept of the “zone of proximal development” proposed by Vygotsky (1978), and they demonstrated as three parts in the booklet (1) individual review problems that students would have completed before joining the workshop, (2) team learning problems to be solved in cooperation with the peer leaders in the workshops, and (3) assessment problems to be solved individually after the workshops to investigate students’ understanding and development. In the review section, the preliminary evaluation was conducted with multiple-choice questions that students could solve on their own about the subject of the week. These materials were supplied to the students before workshops. In Stewart et al.'s (2007) experience, if students take the material in a lecture before the workshop, they are so eager to learn and ready for PLTL instruction. Then, they continued with team problems which consisted of three or four open-ended problems being solved by a team under the guidance of a peer leader. These problems were stepwise or structured problems which included reflective problem solving, interpretation of graphs and data, inductive reasoning (moving from data to structures and mechanisms), and conceptual and critical thinking. They generally had not been able to solve these problems alone, but with the help of peer leaders and social interaction between students, it was tried to increase the potentials of the students. After that, the students were given a quiz (individual problems), consisting of 1 or 2 multiple choice or open-ended questions, which are similar to team learning questions in order to assess individual performance. For multiple choice questions, the correct answer was scored as 1 and an incorrect one was scored as 0. For open-ended items, the rubric presented by Pyburn et al. (2014) was used to analyze the response in terms of its scientific accuracy and its relationship with the question. Thus, full credit was scored as 2, and half credit was scored as 1, while no credit was scored as 0. Feedback regarding individual problems was also provided via team leaders. Students were expected to be able to solve these questions individually after group work. Their potential development was supported by a learning environment in which students were engaged in social interaction by challenging and reasonable activities.
Table 3 Workshop programs
Workshop |
Topics |
Workshop 1 |
Electronic Structure of Atoms/Periodic Properties of the Elements |
Workshop 2 |
Basic Concepts of Chemical Bonding |
Workshop 3 |
Molecular Geometry and Bonding Theories/Gases |
Workshop 4 |
Liquids and Intermolecular Forces/Solid and Modern Materials |
Workshop 5 |
Thermochemistry/Thermodynamics |
Workshop 6 |
Chemical Kinetics |
Peer leaders.
The selection and training of a peer leader is a very significant process for the proper implementation of the model. In the selection process, the course instructor helped to identify the sophomore students who completed the general chemistry course successfully. After interviewing all potential leaders from these students by taking into consideration their communication skills, 14 peer leaders, majoring in different engineering departments, were selected. Two of them passed the general chemistry course with CB but they showed enthusiasm about this project and had good leadership and communication skills. Others’ chemistry grade changed between AA and BB. The next step was to train leaders who were required to attend at least 1.5 hour training sessions, which were held before discussing the questions in chemistry workshops. Time arrangement was made according to their course schedule. Throughout these training sessions, peer leaders were informed about leadership skills, chemistry content knowledge, and pedagogical support, which were discussed in PLTL: A Handbook for Team Leaders written by Roth et al. (2001a, 2001b). Leaders received support for pedagogical information and social communication as well as for discussing the workshop materials for that week when they met.
Related to the leadership skills, they learned about how they become effective leaders in their teams. For the pedagogical support, the researcher gave a brief training on learning theories and styles related to PLTL, learning differences in students, motivation, ethics, teaching strategies and tools such as analogy, paired problem solving, flowcharts, and round-robin. Leaders were expected to select and apply the appropriate teaching strategy and tool in each topic according to the content and student profile in their team. Finally, the content knowledge related to the workshop problems was the main issue in the training session, and nearly seventy-five percent of the time was spent on the understanding of the subject matter. For each session, leaders worked the chemistry problems of the next workshop in groups and covered each concept before the corresponding workshop. The answer key was not given to peer leaders or students as emphasized in the PLTL model (Varma-Nelson and Coppola, 2005). Since it was very important to understand their role in this study, it was stressed that they should behave as guides or facilitators rather than as teachers.
Organizational arrangement.
The organizational arrangement of the PLTL program was vital and should be handled before the implementation especially related to issues of time, group size, location, and teaching resources for providing an effective discussion environment. Thus, firstly, the workshops were arranged to be operated during class time. One lecture session (50 minutes) of three teacher-centered lectures was transformed into a chemistry workshop and 20 minutes were added to this session, so students participated in total 70 minutes peer-led chemistry workshops once in two weeks as part of the course during the study. The twenty-minute difference is due to the arrangement of the classroom setting and distributing the course material to the groups.
A workshop group generally consisted of four to six students plus a peer leader so the average size was five students per group. Students’ gender, success, and major were also taken into account when the teams were formed. As a result, a heterogeneous structure was created by ensuring that each team had students from all departments, both gender and different achievement levels, based on entrance scores to the university (LYS scores). The workshops were conducted in the student study hall in the engineering building so they were familiar with the location and had the opportunity to study as a team because of its physical structure.
Peer-led chemistry workshops
At the beginning of the term, students enrolled in the course were assigned to groups of 6 or 7 students and each group is assigned a number ranging from 1 to 14 and a guiding team leader. Firstly, leaders were trained on the content knowledge and content specific pedagogic knowledge to have necessary background information to handle problems easily. Then, after the content was discussed in the course, students worked with their group members in every workshop in the student study hall under the guidance of their leaders to solve the assigned problems. During the semester, six workshops were conducted, and the topics are given in Table 3. At the workshops, each leader wore an identification card that showed their name and title as a leader. Also, peers wrote their names in a name tag until leaders learned their names and took attendance. Later, leaders started discussing the team problems by means of the teaching techniques taught in the leader training. In this section, leaders gave time to their peers to read and analyze the problems, and then asked some questions to the students and guided them with the tips on how to solve them. It was expected that they were influenced by each other and reached a conclusion with the feedback from their leaders. The understanding of the problem for each peer within the given time period was checked by the leaders. Finally, after all materials were discussed, each leader distributed a quiz related to the topic and gave 10 minutes to answer the questions individually. The workshops and leader training sessions took place simultaneously and continued throughout the semester.
Treatment in the control group: TCI
The first section of the CEAC 105 course was taken as a control group in this study. This group was taught using traditional college instruction (TCI) with weekly three 50 minute lecture periods and a three laboratory hour once a two week but they did not attend the workshops. In the lecture, the same professor teaches the contents like what she did in the experimental group (PLTL). Hence, both groups had the same teacher-centered instruction in the lecture by the course professor who started introducing the concepts and then solved some exercises. The lecture hour in the control group was longer so the instructor solved more problems individually and students followed her to write the notes to their notebooks. The methods used here were mainly lecturing, questioning by the teachers and sometimes discussions between a few students and teachers.
Data analysis
To evaluate the effect of the implementation, quantitative data analysis was conducted in terms of descriptive and inferential statistics using IBM Statistical Package for the Social Sciences (SPSS) 24. All variables and subjects were controlled in terms of missing values. The missing value was 3.9% for Pre-GCCT, and 9.4% for Pre-STAI-S, Pre-SAQ, and LYS scores. Dealing with missing data in pre-tests, a dummy variable adjustment (Allison, 2001) was carried out. Since there was no evidence to have a pattern of missing values in pre-tests, the missing values were replaced with a series of means. Furthermore, descriptive statistics were presented as scores of experimental and control groups’ means and standard deviations. For the inferential statistics, one-way multivariate analysis of covariance (MANCOVA) was performed to determine the effect of PLTL over TCI (independent variable of the study) on students’ conceptual understanding of general chemistry concepts, situational anxiety and social anxiety (dependent variables of the study) after controlling some pre-existing differences due to pre-test scores, trait anxiety scores and university entrance examination scores (covariates). Additionally, all variables were checked for assumptions of MANCOVA, which were sample size, normality, outliers, linearity, multicollinearity, the homogeneity of variances, the homogeneity of regression, and the reliability of covariates, and they were met. All statistical analyses were conducted at the 0.05 significance level.
Results and discussion
The analysis concerning descriptive statistics for the pre-test scores and potential covariates was performed for both experimental and control groups. Table 4 summarizes these descriptives. Furthermore, descriptive statistics for the post-tests were presented in Table 7. The determination of covariates and the effects of treatment on both collective dependent variables and each dependent variable were also discussed in further sections respectively.
Table 4 Descriptive statistics for pre-tests
Tests |
N
|
TCI |
N
|
PLTL |
Mean |
SD |
Mean |
SD |
LYS |
60 |
296.7 |
39.92 |
68 |
293.71 |
39.84 |
STAI-T |
60 |
44.40 |
11.08 |
68 |
42.60 |
11.90 |
Pre-GCCT |
60 |
5.27 |
3.40 |
68 |
5.10 |
3.25 |
Pre-STAI-S |
60 |
48.04 |
6.11 |
68 |
49.85 |
8.01 |
Pre-SAQ |
60 |
92.60 |
13.75 |
68 |
97.38 |
15.29 |
Determination of covariates
If there are pre-existing differences between groups, we cannot conclude that the difference was due to the intervention effect. For this reason, Tabachnick and Fidell (2013) emphasized that it was needed to determine potential covariates that are significantly correlated with dependent variables. The possible covariates in the study were Pre-GCCT, Pre-STAI-S, Pre-SAQ, Pre-STAI-T, and LYS scores. Because a majority of the participants were freshman students, they did not have GPA. Therefore, university entrance scores (LYS scores) of the students were obtained to adjust the pre-existing difference in their achievement.
Prior to main analysis, correlation analysis was performed to check whether they were appropriate to be used as covariates. The assumptions of correlation analysis (the level of measurement, related pairs, normality, linearity, and homoscedasticity) were satisfied before the analysis. According to the results of correlation indicated in Table 5, LYS, STAI-T, Pre-GCCT, and Pre-STAI-S were significantly correlated with at least one of the dependent variables (Post-GCCT, Post-STAI-S, and Post-SAQ) so they were suitable to be used as covariates.
Table 5 Correlations between potential covariates and dependent variables
|
Post-GCCT |
Post-STAI-S |
Post-SAQ |
Note: *Correlation is significant at the 0.05 level (2-tailed). **Correlation is significant at the 0.01 level (2-tailed). |
LYS |
0.195* |
−203* |
−135 |
STAI-T |
−0.178* |
0.768** |
0.181* |
Pre-GCCT |
0.193* |
−0.100 |
0.046 |
Pre-STAI-S |
−0.210* |
0.053 |
0.140 |
Pre-SAQ |
−0.029 |
−0.160 |
0.059 |
Effect of treatment on collective dependent variables
After satisfying all the assumptions, one-way between-group MANCOVA was run for testing the research question of the study at a 0.05 significance level. Table 6 depicts the MANCOVA results of the study.
Table 6 Results of one-way MANCOVA for collective dependent variables
Effect |
Wilks’ lambda |
F
|
df |
Error df |
Sig. |
Partial eta squared |
Observed power |
STAI-T |
0.591 |
57.75 |
3 |
120 |
0.000 |
0.591 |
1.000 |
LYS |
0.937 |
2.68 |
3 |
120 |
0.050 |
0.063 |
0.641 |
Pre-GCCT |
0.965 |
1.46 |
3 |
120 |
0.229 |
0.035 |
0.378 |
Pre-STAI-S |
0.951 |
2.05 |
3 |
120 |
0.110 |
0.049 |
0.515 |
Treatment |
0.764 |
5.77 |
3 |
120 |
0.000 |
0.126 |
0.998 |
The results revealed that there was a statistically significant mean difference in post-test scores between PLTL and TCI with respect to the combined dependent variables of conceptual understanding, state anxiety, and social anxiety in the general chemistry course after controlling the effect of LYS, STAI-T, Pre-GCCT and Pre-STAI-S scores: F(3, 120) = 5.77, p < 0.05, Wilks’ lambda = 0.764. The observed power was with a high value of 0.940. Besides, partial eta squared and Cohen's d values were found to be 0.126 and 0.76, respectively, indicating a nearly high magnitude of the difference among the groups (Cohen, 1988; DeCoster, 2012).
This indicates that 12.6% of the multivariate variance on collective dependent variables was associated with the treatment effect. These results showed that the difference between the PLTL group and TCI groups resulted from the effect of treatment and this difference had practical importance.
Based on the results given in Table 6, there is no statistically significant contribution of the pre-test scores of the General Chemistry Concept Test (F(3, 120) = 1.46, Wilk's lambda = 0.965, p < 0.05) and the pre-test scores of state anxiety (F(3, 120) = 2.05, Wilk's lambda = 0.951, p < 0.05) to their combined dependent variables. In contrast, students’ trait anxiety scores (F(3, 120) = 5.77, Wilk's lambda = 0.764, p < 0.05) and LYS scores (F(3, 120) = 2.68, Wilk's lambda = 0.937, p < 0.05) were significant contributors of their combined dependent variables.
Effect of treatment on each dependent variable
A follow-up ANCOVA was conducted in order to determine the effect of treatment on each dependent variable. The descriptive statistics of dependent variables for both groups are presented in Table 7. Accordingly, the mean differences between groups are slightly different from each other with respect to conceptual understanding (MCG = 9.23, SDCG = 3.38, MEG = 9.53, SDEG = 3.15) and much different with respect to state anxiety (MCG = 45.03, SDCG = 11.57, MEG = 42.54, SDEG = 12.69) and social anxiety (MCG = 82.30, SDCG = 21.11, MEG = 87.03, SDEG = 19.29).
Table 7 Descriptive statistics for post-tests
Tests |
N
|
TCI |
N
|
PLTL |
Mean |
SD |
Adjusted mean |
SE |
Mean |
SD |
Adjusted mean |
SE |
Note: SE: standard error. |
Post-GCCT |
60 |
9.23 |
3.38 |
9.14 |
0.40 |
68 |
9.53 |
3.15 |
9.60 |
0.38 |
Post-STAI-S |
60 |
45.03 |
11.57 |
44.22 |
1.02 |
68 |
42.54 |
12.69 |
43.26 |
0.95 |
Post-SAQ |
60 |
82.30 |
21.11 |
82.31 |
2.58 |
68 |
87.03 |
19.29 |
87.02 |
2.42 |
The results of multiple univariate ANCOVAs are displayed in Table 8. Before checking the p values, firstly, Bonferroni's adjustment was applied to the alpha value by dividing 0.05 by the number of dependent variables (3) to decrease Type I error in the separate univariate analysis (Tabachnick and Fidell, 2013). Consequently, treatment had a significant main effect on all dependent variables based on the new adjusted alpha level of 0.017. The findings given in Table 8 indicated a statistically significant mean difference in students’ conceptual understanding between the PLTL group and the TCI group in favor of PLTL when LYS, STAI-T, Pre-GCCT and Pre-STAI-S were controlled (F(1, 122) = 5.378, p < 0.017). The adjusted mean scores of the General Chemistry Concept Post-test in Table 6 pointed out that the students in the PLTL group had significantly higher mean scores (Madj = 9.60, SE = 0.38) than those in the TCI group (Madj = 9.14, SE = 0.40). In other words, the PLTL group performed better in the General Chemistry Concept Test. The guidelines proposed by Cohen for eta squared can also be implemented for interpreting the strength of partial eta squared where 0.01 is considered small, 0.06 is medium and 0.14 is large (Pallant, 2011). The partial eta squared value for conceptual understanding was found to be 0.081, which means medium effect size. This value implied that the proportion of variance in the students’ conceptual understanding explained by the treatment was 8.1%. Similarly, Cohen's d value was also calculated as the medium effect size of 0.59 for conceptual understanding (Cohen, 1988; DeCoster, 2012). The power value was also found to be 0.835. Consequently, it can be implied that the difference among the groups arose from the treatment effect and had practical significance.
Table 8 Results of follow-up ANCOVAs
Source |
Dependent variable |
F
|
Sig. |
Partial eta squared |
Observed power |
Treatment |
Post-GCCT |
5.378 |
0.006 |
0.081 |
0.835 |
Post-STAI-S |
4.665 |
0.011 |
0.071 |
0.776 |
Post-SAQ |
7.060 |
0.001 |
0.104 |
0.924 |
These findings supported that the PLTL model is likely to lead to better conceptual learning in the general chemistry course than traditional college instruction (TCI) did. Therefore, the current study provides further empirical support for previous studies showing the effectiveness of peer-led team learning over traditional instruction (Cracolice and Deming, 2004; Varma-Nelson and Coppola, 2005; Gafney and Varma-Nelson, 2008; Bramaje and Espinosa, 2013). The probable underlying causes that PLTL instruction was effective in improving students’ conceptual understanding in general chemistry can be related to the characteristics of the model. Cracolice and Deming (2004) state that the PLTL model provides an environment where students can have the opportunity to become active participants in their learning process. For example, students easily asked questions, worked together and discussed and negotiated the ideas in the PLTL group of this study. Such active learning environments can help students to gain a deeper understanding of the subject matter, thus promoting the level of student meaningful learning (Duit and Treagust, 2003; Duit et al., 2008). Additionally, the reasoning ability and problem-solving skills might have played a role in the difference between the PLTL and the TCI group in terms of students’ understanding of concepts.
Deming et al. (2003) point out that there is a significant direct relationship between the reasoning ability and students’ capacity to solve conceptual problems in general chemistry. Since students are challenged to use their problem-solving skills, thinking skills, and reasoning abilities in PLTL workshops, they are able to improve their reasoning abilities and develop strong conceptual understanding (Cracolice and Deming, 2004).
Although the PLTL approach had a significant positive impact on conceptual understanding, the magnitude of the mean difference among the groups was not as much as expected. This less effective outcome may result from the duration of the PLTL workshops. The suggested length of the workshop time is weekly 90–120 minutes (Gafney and Varma-Nelson, 2008). However, in this study, the workshop took place biweekly and lasted 70 minutes. Gafney and Varma-Nelson (2008) claim that if workshops are implemented in a shorter time than the recommended duration such as 60 or 75 minutes, the same effect on student performance as longer ones cannot be observed.
Another significant mean difference was detected between PLTL and TCI in terms of students’ state anxiety after controlling LYS, STAI-T, Pre-GCCT, and Pre-STAI-S, F(1, 122) = 4.665, p < 0.017. The adjusted mean scores on the state anxiety post-test indicated significantly lower mean scores in the PLTL group (Madj = 43.26, SE = 0.95) compared to the TCI group (Madj = 42.22, SE = 1.02). This means that the PLTL group had a lower level of state anxiety than the TCI group. The partial eta squared value was found to be 0.071, which means medium effect size and the value of power was found to be 0.776, suggesting that treatment leads to a meaningful effect on students’ anxiety. Besides, Cohen's d value was also calculated as the medium effect size of 0.55 for state anxiety (Cohen, 1988; DeCoster, 2012). Consequently, it can be implied that along with increasing students’ conceptual understanding, the PLTL model appears to be an effective way of decreasing students’ anxiety. This finding might support the fact that while students feel anxious, threatened, or stressed in lecture hours, they feel more relieved, comfortable and content in a PLTL workshop environment which focuses on the supportive and small-group discussion under the guidance of a peer leader instead of a course instructor or assistants, as stated by Gafney and Varma-Nelson (2008). In other words, students may feel more comfortable about sharing their ideas in smaller peer groups than speak up in front of the whole class. On the other hand, the result is inconsistent with the findings of Chan and Bauer (2015) who report no significant difference in students’ anxiety between students of the PLTL group and students of the non-PLTL group (alternative learning activities: self-organized study group, instructor-led review sessions, drop-in tutorials led by graduate or undergraduate chemistry majors, and instructor office hours) and a slightly significant increase in students’ anxiety and fear of chemistry throughout the semester.
Finally, the results of the follow-up ANOVA revealed that after adjusting LYS, STAI-T, Pre-GCCT and Pre-STAI-S scores, there was a statistically significant mean difference in students’ social anxiety among the groups, F(1, 122) = 7.060, p < 0.017, and partial eta squared = 0.104. The adjusted mean scores of the students in the PLTL group (Madj = 87.02, SE = 2.42) were higher than those in the TCI group (Madj = 82.31, SE = 2.58) with regard to the social anxiety post-test. The power value was 0.924. In addition, the partial eta squared value of 0.104 emphasizes the strong and meaningful difference among the groups due to the effect of treatment. Also, Cohen's d value was found to be 0.68, which indicates medium effect size for social anxiety (Cohen, 1988; DeCoster, 2012). From this result, it can be claimed that although the PLTL model assisted students to improve their conceptual understanding of general chemistry concepts and reduce situational anxiety, it was not successful in decreasing students’ social anxiety. This negative result was surprising and it contrasts with the study of Johnson and Johnson (1994) that advocates the advantages of collaborative small group work on students’ self-esteem and social skills.
However, the reason why PLTL instruction was not effective in alleviating students’ anxiety about social situations may be explained by the fact that control group students did not participate in any other activity. Therefore, they did not engage in any situation which causes them to express themselves, and explain some ideas and knowledge as a member of any social group consisting of opposite sex and relative strangers. Furthermore, the treatment was applied only for one semester so students from the PLTL group met only six times. As a result, students may see the group work as a new experience or challenge for them and need time to get used to working together with other students and overcome any problems that occur in the group. It is possible to get a more accurate result by examining the effect of the PLTL model on social anxiety after a long-term practice. In addition, since the biweekly schedule of workshops, the students generally tried to handle the concepts of two chapters during one workshop so they concentrated on problem-solving and learning new information. The dialogue between students and a leader was commonly much more compared to social interaction and dialogue between students. In that time, they discussed and solved the problems, and continued with the next questions. Thus, the ability to interact with each other may not be well established in order to develop strong relationships and interpersonal skills. For such reasons, the students in the PLTL group may not have overcome social anxiety.
Conclusion
In conclusion, the current study approves and expands many findings related to the effectiveness of a relatively new evidence-based approach, peer-led team learning, on students’ conceptual understanding in the context of undergraduate general chemistry after controlling their LYS score, trait anxiety score and pre-test score of the General Chemistry Concept Test and state anxiety. This study also sustains a body of evidence that the PLTL model is better to alleviate students’ situational anxiety in general chemistry, while it is not effective at reducing social anxiety when compared to traditional college instruction (TCI) after adjusting those variables. Accordingly, the results of this study support the chemistry education literature in such a way that PLTL can be implemented in postsecondary chemistry classes to promote the cognitive and affective development of students despite its adverse effect on social anxiety. A long-term successful application of the PLTL model may help students to ease their social anxiety because team leaders get more experience with time and can create a more productive environment for students to develop and sustain positive social interactions with their peers.
This study has several implications for educators and researchers not only in chemistry but also in other STEM courses. Based on the literature about effective college teaching and learning, it is clear that evidence-based teaching strategies emphasizing active and collaborative instruction are more effective in fostering deep and meaningful learning than traditional instruction (Kuh et al., 2005; Pascarella and Terenzini, 2005). Peer-led team learning, as an evidence-based teaching strategy, enables students to involve themselves actively in the learning process through working as a team under the guidance of a peer-leader. The present study provides not only evidence about the instructional effectiveness of the PLTL model on the conceptual understanding of general chemistry but also sufficient information regarding six essential principles for the adaptation of PLTL in other STEM courses. Consequently, this study can be used as a guideline for college teachers to design more effective instruction in STEM courses, particularly in a general chemistry context.
It is important to note that students’ affective development is as significant as their cognitive development since they have an impact on students’ learning too (Chandran et al., 1987; Rennie and Punch, 1991; Berberoglu and Demircioglu, 2000; Osborne et al., 2003). If students are anxious, nervous, and uncomfortable in a classroom environment, they are unlikely to participate actively in their learning process, and thus, such a situation can influence their performance negatively. According to the findings of this study, instructors can lessen their students’ anxiety with the implementation of peer-led chemistry workshops in which students feel more comfortable through collaborative teamwork.
Related to the limitations, the study was performed in only one semester. In addition, participants were not selected by random sampling; instead, groups did. This can be a problem for the independence of observation because they were instructed with different teaching methods as a group within a classroom, and in the PLTL group, students were working in small groups (Gravetter and Wallnau, 2007; Stevens, 2009). Consequently, they were likely to interact with each other in some way, and each member of the group may have influenced the general chemistry performance or anxiety level of all other group members. Therefore, depending on the extent of the dependence of observations, this study may present inflated estimates of effect sizes and deflated estimates of p-values. Besides, it was tried to minimize the threats to the internal validity of the results by controlling some extraneous variables in the study.
To sum up, not only for the US context but also for many other countries, initiatives to improve the quality of education at the tertiary level are more limited when compared to other levels. This study is one of the first systematic attempts to test the impact of the PLTL model on cognitive and affective aspects in the context where the study is conducted. The findings promise benefits of the model for improving conceptual learning while alleviating state anxiety in freshman students in General Chemistry.
Conflicts of interest
There are no conflicts to declare.
Appendix
Sample items of the GCCT
A 100 g sample of a metal was heated to 100 °C and then quickly transferred to an insulated container holding 100 g of water at 22 °C. The temperature of the water increased to reach a final temperature of 35 °C. Which of the following can be concluded?‡
(a) The metal temperature changed more than the water temperature did; therefore the metal lost more thermal energy than the water gained.
(b) The metal temperature changed more than the water temperature did, but the metal lost the same amount of thermal energy as the water gained.*
(c) The metal temperature changed more than the water temperature did; therefore the heat capacity of the metal must be greater than the heat capacity of the water.
(d) The final temperature is less than the average starting temperature of the metal; therefore the total energy of the metal and water decreases.
(e) The final temperature is higher than the average starting temperature of the water; therefore the total energy of the metal and water increases.
According to Pauling's electronegativity scale, the electronegativity of hydrogen is 2.1 and the electronegativity of chloride is 3.0. What is the type of bond between hydrogen and chloride in hydrogen chloride, HCl. (1H, 1A; 17Cl, 7A)
(a) ionic bond
(b) covalent bond*
(c) hydrogen bond
(d) metallic bond
What is the reason for your answer to the previous question?
(a) The hydrogen atom and chlorine atom each share one electron in the compound.*
(b) Hydrogen is bonded to highly electronegative atoms such as F, Cl and O
(c) HCl is a strong acid, and it decomposes to its ions when it dissolves in water
(d) Hydrogen transfers one electron to chlorine to form a compound.
The correct answer is presented with an * sign.
Acknowledgements
We would like to thank to CEAC faculty members, head of the department, Prof. Seniz Ozalp-Yaman, and each team leader for their continued support throughout the project.
References
- Al-Balushi S. M., Ambusaidi A. K., Al-Shuaili A. H. and Taylor N., (2012), Omani twelfth grade students’ most common misconceptions in chemistry, Sci. Educ. Int., 23(3), 221–240.
- Alger T. D. and Bahi S., (2004), An experiment in improving scores on ACS course-specific examinations at Southern Utah University, Progressions: The PLTL Project Newsletter, 5(2), 7–10.
- Allison P. D., (2001), Missing Data, Sage University Papers Series on Quantitative Applications in the Social Sciences, 07–136, Thousand Oaks, CA: Sage.
- American Chemical Society [ACS], (2014), National chemistry olympiad local section exam, retrieved from https://www.acs.org/content/dam/acsorg/education/students/highschool/olympiad/pastexams/2014-usnco-local-exam.pdf.
- American Chemical Society (ACS), (2016), Chemistry olympiad exams, retrieved from https://www.acs.org/content/acs/en/education/students/highschool/olympiad/pastexams.html.
- American Psychiatric Association, (2000), Diagnostic and statistical manual of mental disorders – text revision (DSM-IV-TR), Washington, DC: American Psychiatric Association.
- Astin A. W., (1993), What matters in college? Four critical years revisited, San Francisco, CA: Jossey-Bass.
- Ayyıldız Y. and Tarhan L., (2012), The effective concepts on students’ understanding of chemical reactions and energy, Hacet. Univ. J. Educ., 42, 72–83.
- Báez-Galib R., Colón-Cruz H., Resto W. and Rubin M., (2005), Chem-2-chem: a one-to-one supportive learning environment for chemistry, J. Chem. Educ., 82, 1859–1863.
- Berberoglu G. and Demircioglu H., (2000), Factors affecting achievement in general chemistry courses among science major students, Educ. Sci., 25(118), 35–42.
- Bozkoyun Y., (2004), Facilitating conceptual change in learning rate of reaction concepts, Master's thesis, Middle East Technical University, Ankara, Turkey.
- Bramaje G. P. and Espinosa A. A., (2013), Peer-led team learning approach: effects on students’ conceptual understanding and attitude towards chemistry, Int. J. Learn. Teach., 5(2), 55–77.
- Brown T. E., LeMay H. E., Bursten B. E., Murphy C., Patrick Woodward P. and Stoltzfus M. E., (2013), Chemistry: the central science, 13th edn, Upper Saddle River, NJ: Pearson Education.
- Caballo V. E., Salazar I. C., Irurtia M. J., Arias B. and Hofmann S. G., (2010a), Measuring social anxiety in 11 countries: development and validation of the social anxiety questionnaire for adults, Eur. J. Psychol. Assess., 26, 95–107 DOI:10.1027/1015-5759/a000014.
- Caballo V. E., Salazar I. C., Arias B., Irurtia M. J., Calderero M. and CISO-A Research Team Spain, (2010b), Validation of the social anxiety questionnaire for adults (SAQ-A30) with Spanish university students: similarities and differences among degree subjects and regions, Behav. Psychol., 18, 5–34.
- Chan J. Y. K. and Bauer C. F., (2015), Effect of peer-led team learning (PLTL) on student achievement, attitude, and self-concept in college general chemistry in randomized and quasi-experimental designs, J. Res. Sci. Teach., 52(3), 319–346.
- Chandran S., Treagust D. and Tobin K., (1987), The role of cognitive factors in chemistry achievement, Journal of Research in Science Teaching, 24(2), 145–160.
- Cohen J., (1988), Statistical power analysis for the behavioral sciences, 2nd edn, Hillsdale, NJ: Erlbaum.
- College Board, (2014), AP chemistry: course and exam description, retrieved from http://media.collegeboard.com/digitalServices/pdf/ap/ap-chemistry-course-and-exam-description.pdf.
- College Board, (2016), AP chemistry: The exam, retrieved from https://apcentral.collegeboard.org/courses/ap-chemistry/exam?course=ap-chemistry.
- Cracolice M. S., (2000), Vygotsky's zone of proximal development: a theory base for peer-led team learning, Progressions: the PLTL Project Newsletter, 1(2), retrieved from http://pltlis.org/wp-content/uploads/2012/10/PLTL-and-Vygotsky-Vygotsky-ZPD-Cracolice.pdf.
- Cracolice M. S. and Deming J. C., (2004), Peer-led team learning: Promoting conceptual understanding and reasoning ability, published online for the Winter 2005 CONFCHEM: Trends and New Ideas in Chemical Education, retrieved from https://confchem.ccce.divched.org/sites/confchem.ccce.divched.org/files/2005WinterConfChemP2.pdf.
- Crocker L. and Algina J., (2008), Introduction to classical and modern test theory, Mason, Ohio: Cengage Learning.
- Czerniak C. and Chiarelott L., (1984), Science anxiety: an investigation of science achievement, sex and grade level factors, Paper presented at the 68th Annual Meeting of the American Educational Research Association, New Orleans, LA.
- DeCoster J., (2012), Converting effect sizes, retrieved from http://www.stat-help.com/spreadsheets/Convertingeffectsizes2012-06-19.xls.
- Deming J. C., Ehlert B. E. and Cracolice M. S., (2003), Algorithmic and conceptual understanding differences in general chemistry: a link to reasoning ability, Paper presented at the American Chemical Society National Meeting, New York, NY.
- Drane D., Micari M. and Light G., (2014), Students as teachers: effectiveness of a peer-led STEM learning programme over 10 years, Educ. Res. Eval., 20(3), 210–230.
- Driver R. A., Asoko H., Leach J., Mortimer E. and Scott P., (1994), Constructing scientific knowledge in the classroom, Educ. Res., 23, 5–12.
- Duit R. and Treagust D. F., (2003), Conceptual change: a powerful framework for improving science teaching and learning, Int. J. Sci. Educ., 25, 671–688.
- Duit R., Treagust D. and Widodo A., (2008), in Teaching science for conceptual change: theory and practice, S. Vosniadou et al., (ed.), International handbook of research on conceptual change, New York: Routledge, pp. 629–646.
- Fraenkel J. R. and Wallen N. E., (2006), How to design and evaluate research in education, 6th edn, New York: The McGraw-Hill.
- Gafney L., (2001a), Evaluating student performance, Progressions: The PLTL Project Newsletter, 2(2), 3–4.
- Gafney L., (2001b), in Evaluation: specific practice, Gosser D. K., Cracolice M. S., Kampmeier J. A., Roth V., Strozak V. S. and Varma-Nelson P. (ed.), Peer-led team learning: a guidebook, Upper Saddle River, NJ: Prentice Hall, pp. 129–133.
- Gafney L., (2001c), in Workshop evaluation, Gosser D. K., Cracolice M. S., Kampmeier J. A., Roth V., Strozak V. S. and Varma-Nelson P. (ed.), Peer-led team learning: a guidebook, Upper Saddle River, NJ: Prentice Hall, pp. 75–92.
- Gafney L. and Varma-Nelson P., (2007), Evaluating peer-led team learning:
a study of long-term effects on former workshop peer leaders, J. Chem. Educ., 84, 535–539.
- Gafney L. and Varma-Nelson P., (2008), Peer-led team learning: evaluation, dissemination, and institutionalization of a college level initiative, Innovations in science education and technology, vol. 16, Weston, MA: Springer.
- Gravetter F. J. and Wallnau L. B., (2007), Statistics for the behavioral sciences, 7th edn, Belmont, CA: Wadsworth.
- Gredler M. E., (2009), Learning and Instruction: Theory into Practice, 6th edn, Upper Saddle River, NJ: Merrill Pearson.
- Gosser D. K. and Roth V., (1998), The workshop chemistry project: peer-led team learning, J. Chem. Educ., 75 (2), 185–187.
- Higher Education Research Institute, (2010), Degrees of success: bachelor's degree completion rates among initial STEM majors (HERI/CIRP Research Brief), Los Angeles, CA: Author.
- Hockings S. C., DeAngelis K. A. and Frey R. F., (2008), Peer-led team learning in general chemistry: implementation and evaluation, J. Chem. Educ., 85(7), 990–996.
- Holme T. A., Luxford C. J. and Brandriet A., (2015), Defining conceptual understanding in general chemistry, J. Chem. Educ., 92, 1477–1483.
- Johnson R. T. and Johnson D. W., (1994), in An overview of cooperative learning, J. Thousand, A. Villa and A. Nevin (ed.), Creativity and collaborative Learning, Baltimore: Brookes Press.
- Kampmeier J. A. and Varma-Nelson P., (2009), in Peer-led team learning: organic chemistry, N. J. Pienta, M. M. Cooper and T. J. Greenbowe (ed.), Chemists’ guide to effective teaching, vol. 2, Upper Saddle River, NJ: Pearson Prentice Hall.
- Kaya E., (2011), The effect of conceptual change based instruction on students’ understanding of rate of reaction concepts, Doctoral dissertation, Middle East Technical University, Ankara, Turkey.
- Kuh G., Kinzie J., Schuh J. and Witt E., (2005), Student success in college: Creating conditions that matter, Washington, DC: Association for the Study of Higher Education.
- Lewis S. E., (2011), Retention and Reform: an evaluation of peer-led team learning, J. Chem. Educ., 88, 703–707.
- Lovitts B. E., (2001), Leaving the ivory tower: the causes and consequences of departure from doctoral study, Lanham, MD: Rowman & Littlefield.
- Lyon D. C. and Lagowski J. J., (2008), Effectiveness of facilitating small-group learning in large lecture classes: a general chemistry case study, J. Chem. Educ., 85(11), 1571–1576.
- Mallow J. V. and Greenburg S. L., (1982), Science anxiety: causes and remedies, J. Coll. Sci. Teach., 11, 356–358.
- Mitchell Y. D., Ippolito J. and Lewis S. E., (2012), Evaluating peer-led team learning across the two semester general chemistry sequence, Chem. Educ. Res. Pract., 13, 378–383.
- Mulford D. R. and Robinson W. R., (2002), An inventory for alternate conceptions among first-semester general chemistry students, J. Chem. Educ., 79(6), 739–744.
- Osborne J., Simon S. and Collins S., (2003), Attitudes towards science: a review of the literature and its implications, Int. J. Sci. Educ., 25(9), 1049–1079.
- Pabuccu A., (2004), Effect of conceptual change texts accompanied with analogies on understanding of chemical bonding concepts, Master's thesis, Middle East Technical University, Ankara, Turkey.
- Pabuccu A. and Geban O., (2006), Remediating misconceptions concerning chemical bonding through conceptual change text, Hacet. Univ. J. Educ., 30, 184–192.
- Pallant J., (2011), SPSS survival manual: a step by step guide to data analysis using SPSS, 4th edn, Crows Nest, NSW: Allen & Unwin.
- Pascarella E. and Terenzini P., (2005), How college affects students: a third decade of research, San Francisco: Jossey-Bass.
- Phillips D. C., (2000), An opinionated account of the constructivist landscape, in Constructivism in education: ninety-ninth yearbook of the National Society of the Study of Education, D. C. Phillips (ed.), Chicago: University of Chicago Press, pp. 1–16.
- Pyburn D. T., Pazicni S., Benassi V. A., and Tappin E. M., (2014), The testing effect: an intervention on behalf of low-skilled comprehenders in general chemistry, J. Chem. Educ., 91(12), 2045–2057.
- Rennie L. J. and Punch K. F., (1991), The relationship between affect and achievement in science, J. Res. Sci. Teach., 28(2), 193–209.
- Roth V., Cracolice M. S., Goldstein E. and Snyder V., (2001a), Workshop leader training, in Peer-led team learning: a guidebook, Gosser D. K., Cracolice M. S., Kampmeier J. A., Roth V., Strozak V. S. and Varma-Nelson P. (ed.), Upper Saddle River, NJ: Prentice Hall, pp. 35–60.
- Roth V., Goldstein E. and Marcus G. (ed.), (2001b), Peer-led team learning: a handbook for team leaders, Upper Saddle River, NJ: Prentice Hall.
- Seymour E. and Hewitt N. M., (1997), Talking about leaving: why undergraduates leave the sciences, Boulder, CO: Westview.
- Shields S. P., Hogrebe M. C., Spees W. M., Handlin L. B., Noelken G. P., Riley J. M. and Frey R. F., (2012), A transition program for underprepared students in general chemistry: diagnosis, implementation, and evaluation, J. Chem. Educ., 89, 995–1000.
- Spielberger C. D., (1972), Anxiety as an emotional state, in Anxiety: current trends in theory and research, Spielberger C. D. (ed.), New York: Academic Press, vol. 1, pp. 33–46.
- Spielberger C. D., Gorsuch R. L., Lushene R., Vagg P. R. and Jacobs G. A., (1983), Manual for the State-Trait Anxiety Inventory (Form Y), Palo Alto, CA: Consulting Psychologists Press.
- Sridevi K. V., (2013), A study of relationship among general anxiety, test anxiety and academic achievement of higher secondary students, J. Educ. Pract., 4(1), 122–130.
- Stevens J., (2009), Applied multivariate statistics for the social sciences, 5th edn, Mahwah, NJ: Lawrence Erlbaum.
- Stewart B. N., Amar F. G. and Bruce M. R. M., (2007), Challenges and rewards of offering peer led team learning (PLTL) in a large general chemistry course, Aust. J. Educ. Chem., 67, 31–36.
- Strozak V. S. and Wedegaertner D. K., (2001), Writing workshop materials, in Peer-led team learning: a guidebook, Gosser D. K., Cracolice M. S., Kampmeier J. A., Roth V., Strozak V. S. and Varma-Nelson P. (ed.), Upper Saddle River, NJ: Prentice Hall, pp. 20–34.
- Tabachnick B. G. and Fidell L. S, (2013), Using multivariate statistics, 6th edn, Upper Saddle River, NJ: Pearson.
- Tien L. T., Roth V. and Kampmeier J. A., (2002), Implementation of a peer-led team learning instructional approach in an undergraduate organic chemistry course, J. Res. Sci. Teach., 37, 606–632.
- Tinto V., (1975), Dropout from higher education: a theoretical synthesis of recent research, Rev. Educ. Res., 45(1), 89–125.
- Tobias S., (1990), They’re not dumb, they’re just different—stalking the second tier, Tucson, AZ: Research Corporation.
- Tobias S., (1992), Revitalizing College Education, Tucson: Research Corporation.
- Treagust D. F., (1986), Evaluating students’ misconceptions by means of diagnostic multiple choice items, Res. Sci. Educ., 16, 199–207.
- Uzuntiryaki E., (2003), Effectiveness of constructivist approach on students’ understanding of chemical bonding concepts, Doctoral dissertation, Ankara, Turkey: Middle East Technical University.
- Varma-Nelson P., (2006), Peer-led team learning: the model, its dissemination and its future, Metropol. Univ. J., 14(4), 19–29.
- Varma-Nelson P. and Coppola B. P., (2005), Team learning, in Chemists’ guide to effective teaching, N. J. Pienta M. M. Cooper and T. J. Greenbowe (ed.), Upper Saddle River, NJ: Pearson, vol. I, pp. 155–169.
- Varma-Nelson P., Cracolice M. and Gosser D., (2004), Peer-led team learning: a student-faculty partnership for transforming the learning environment, in Invention and impact: building in undergraduate science, technology, engineering, and mathematics education, Cunningham S. and George Y. (ed.), Washington, DC: American Association for the Advancement of Science, pp. 43–48.
- Vitasari P., Wahab M. N. A., Othman A., Herawan T. and Sinnadurai S. K., (2010), The relationship between study anxiety and academic performance among engineering students, Proc. Soc. Behav. Sci., 8, 490–497 DOI:10.1016/j.sbspro.2010.12.067.
- Vygotsky L. S., (1978), Mind in society: the development of higher psychological processes, Cambridge, MA: Harvard University Press.
- Wilson S. B. and Varma-Nelson P., (2016), Small groups, significant impact: a review of peer-led team learning research with implications for STEM education researchers and faculty, J. Chem. Educ., 93, 1686–1702.
- Yalcınkaya E., (2010), Effect of case based learning on 10th grade students’ understanding of gas concepts, their attitude and motivation, Doctoral dissertation, Ankara, Turkey, Middle East Technical University.
Footnotes |
† This study is part of the first author's doctoral dissertation. |
‡ Note: © 2014. The College Board. Advanced Placement Program, AP. |
|
This journal is © The Royal Society of Chemistry 2018 |