DOI:
10.1039/C8RA09575B
(Paper)
RSC Adv., 2018,
8, 41355-41357
Enantioselective Nozaki–Hiyama–Kishi allylation-lactonization for the syntheses of 3-substituted phthalides†
Received
21st November 2018
, Accepted 23rd November 2018
First published on 12th December 2018
Abstract
We report the synthesis of bipyridyl ligands and these ligands were applied in the chromium-catalyzed enantioselective Nozaki–Hiyama–Kishi allylation of substituted (2-ethoxycarbonyl)benzaldehydes and subsequently lactonized to synthesize phthalides with an optimal enantioselectivity of 99%. This approach was applied to synthesize (S)-cytosporone E at a 71% yield in three steps.
The addition of organochromium reagents to carbonyl compounds was pioneered by Nozaki et al.1 and is a versatile reaction forming carbon–carbon bonds for use with various building blocks to synthesize complex natural products and pharmaceuticals.2 Organochromium reagents are formed in situ through the oxidative addition of Cr(II) species to allyl, propargyl halides, and triflates (aryl, vinyl halides, and triflates requiring catalytic amounts of Ni(II) salt)3a,b and can be added to aldehydes and ketones to produce the corresponding alcohols.3 The major disadvantage of the original NHK allylation method is the excessive amount of Cr(II) salts necessary for the formation of the organochromium reagent.1,4 Fürstner and Shi5 reported NHK allylation that requires only catalytic amounts of active Cr(II) species; Mn is used as the reducing agent, and trimethylchlorosilane prolongs the catalytic cycle. These improvements have driven chemists to improve the enantioselectivity of this reaction. Several chiral ligands have been used as enantioselective catalysts for the allylation of aldehydes.6 A chiral bipyridyldiol was used as the efficient enantioselective catalyst in an asymmetric NHK reaction to provide the corresponding homoallylic alcohol and simultaneously form lactone in high enantiomeric excess (90% ee).6n The successful application of these ligand systems motivated us to investigate the NHK allylation of (2-ethoxycarbonyl)benzaldehydes and simultaneous lactonization to produce phthalides. This approach was then employed to enantioselectively synthesize (S)-cytosporone E.
Pineno-bipyridyl alcohols are readily obtainable from (+)-α-pinene in four steps (Scheme 1); these steps include the stereoselective alkylation of chiral bipyridine with ketones to obtain desired products 4–7.6o In a previous work, we used benzaldehyde, allyl chloride, and ligand 6 under Fürstner's reaction conditions to obtain the corresponding product with 70% yield and 75% ee.6n In the same study, a bipyridyldiol was applied under Fürstner's reaction conditions to prepare phthalide 8 with 92% yield and 90% ee. To improve the enantioselectivity of the NHK reaction using ligand 6 under Fürstner's reaction conditions, we obtained the optimal reaction conditions using benzaldehyde, allyl bromide, CrCl3 (10 mol%), ligand 6 (30 mol%), and Et3N (60 mol%) to provide a product with 83% yield and 90% ee.6o
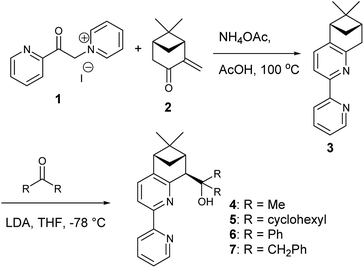 |
| Scheme 1 Ligand synthesis.6o | |
Initial evaluation of non-symmetrical bipyridine ligands (4–7) in the Cr(II)-catalyzed addition of allyl bromide to (2-ethoxycarbonyl)-3,5-dimethoxybenzaldehyde revealed that ligand 6 has excellent reactivity and enantioselectivity (Table 1, entry 7). The resulting homoallylic alcohols can lactonize to give the phthalide 8. Allylation of (2-ethoxycarbonyl)-3,5-dimethoxybenzaldehyde using Cr–6 complex was optimized by performing the reaction in various solvents and at various reaction temperatures. (See ESI.†) Reactions performed at 0 °C in THF afforded better results than all of screened conditions (Table 1).
Table 1 Optimization of chiral bipyridyl alcohol ligands for the chromium-catalyzed addition of allyl bromide to (2-ethoxycarbonyl)-3,5-dimethoxybenzaldehyde
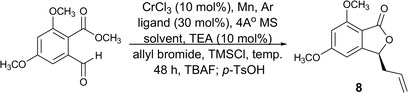
|
Entry |
Ligand |
Solvent |
Temp [°C] |
Yielda [%] |
eeb [%] |
Isolated yield. The ee% were determined on a Chiracel OJ HPLC column. |
1 |
4 |
THF |
r.t. |
86 |
83 |
2 |
5 |
THF |
r.t. |
89 |
89 |
3 |
6 |
THF |
r.t. |
89 |
93 |
4 |
7 |
THF |
r.t. |
85 |
90 |
5 |
4 |
THF |
0 |
88 |
87 |
6 |
5 |
THF |
0 |
89 |
89 |
7 |
6 |
THF |
0 |
86 |
97 |
8 |
7 |
THF |
0 |
87 |
92 |
9 |
6 |
CH2Cl2 |
r.t. |
58 |
86 |
10 |
6 |
DMF |
r.t. |
36 |
84 |
11 |
6 |
Toluene |
r.t. |
NR |
— |
12 |
6 |
Ether |
r.t. |
NR |
— |
13 |
6 |
CH2Cl2 |
0 |
68 |
89 |
14 |
6 |
DMF |
0 |
15 |
82 |
15 |
6 |
Toluene |
0 |
NR |
— |
16 |
6 |
Ether |
0 |
NR |
— |
Ligands 4 and 5, which had less steric hindrance, showed slightly lower enantioselectivity than ligands 6 and 7. The reaction conducted at room temperature resulted in a product with less enantioselectivity than the reaction at 0 °C. Table 1 shows the results of allylation using various solvents. Using toluene and diethyl ether did not result in formation of the product. Dichloromethane and DMF exhibited some transformation with low yield. THF exhibited the most desirable result, with high yield and enantioselectivity. Considering the optimized reaction conditions, we investigated the generality of the NHK allylation followed by transesterification to produce the corresponding phthalides by using various aldehydes (Table 2). All reactions were completed within 48 h and produced adducts with good to excellent yields (70%–90%) and enantioselectivities (94%–99% ee). The position and electronic properties of substituents on the aromatic ring exerted a weak influence on the enantioselectivity of the reaction. Substrates with electron-withdrawing (Table 2, entries 8 and 9), electron-donating (Table 2, entries 1–3, 5–7, and 10), and neutral (Table 2, entry 4) groups in various substitution patterns participated in this reaction efficiently (general procedure for preparation of phthalides can be found in the ESI†).
Table 2 Catalytic enantioselective synthesis of phthalides
This highly enantioselective catalyst was explored to determine its promise as an enantioselective catalyst of NHK allylation–lactonization. A natural product, (R)-cytosporone E, was synthesized through asymmetric allylation–lactonization. Ohzeki and Mori synthesized (S)-cytosporone E with 11.5% yield (nine steps) and 45% ee using AD-mix-β® as an asymmetric dihydroxylation reagent.7 The enantiomers of a tris(tert-butyldimethylsilyl)-protected derivative was separated by preparative HPLC on Chiralcel® OD to obtain pure (S)- cytosporone E (98.4% ee). Wyatt et al. synthesized racemic cytosporone E at 41.4% yield (seven steps).8 Therefore, asymmetric NHK allylation–lactonization was used in this study as an efficient allylation reaction using ligand 6 as the enantioselective catalyst to provide the corresponding homoallylic alcohol and simultaneously form lactone 10 in a high yield (89%) and enantiomeric excess (98.8%). Lactone 10 was converted into compound 18 through treatment with 9-BBN, Cs2CO3(aq), bromobutane, Pd(OAc)2, and PCy3 (Scheme 2). Finally, the methoxyl groups of 18 were cleaved using BBr3 to obtain (S)-cytosporone E (19) at 71% yield (three steps).
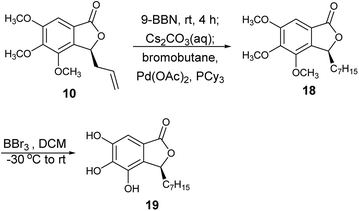 |
| Scheme 2 Synthesis of (S)-cytosporone E. | |
In summary, a chiral Cr(II) complex prepared using bipyridine alcohol and CrCl3 efficiently catalyzed the enantioselective NHK allylation–lactonization of substituted (2-ethoxycarbonyl)-benzaldehyde to produce phthalides with excellent enantioselectivity (up to 99% ee). This approach was used to synthesize (S)-cytosporone E and obtained excellent yield and enantioselectivity.
Conflicts of interest
There are no conflicts to declare.
Acknowledgements
The authors thank Ms. L. M. Hsu, at the Instruments Center, National Chung Hsing University, for her help in obtaining mass spectral data, and the Ministry of Science and Technology, Taiwan, for financially supporting this research under the contract: MOST 103-2113-M-277-001-MY3.
Notes and references
-
(a) Y. Okude, S. Hirano, T. Hiyama and H. Nozaki, J. Am. Chem. Soc., 1977, 99, 3179 CrossRef CAS;
(b) Y. Okude, T. Hiyama and H. Nozaki, Tetrahedron Lett., 1977, 18, 3829 CrossRef.
- For reviews, see:
(a) M. Avalos, R. Babiano, P. Cintas, J. L. Jimenez and J. C. Palacios, Chem. Soc. Rev., 1999, 28, 169 RSC;
(b) K. Takai and H. Nozaki, Proc. Jpn. Acad., Ser. B, 2000, 76, 123 CrossRef;
(c) G. C. Hargaden and P. J. Guiry, Adv. Synth. Catal., 2007, 349, 2407 CrossRef CAS;
(d) E. T. da Penha, J. A. Forni, A. F. P. Biajoli and C. R. D. Correia, Tetrahedron Lett., 2011, 52, 6342 CrossRef CAS;
(e) H.-Y. Wang, A. Kato, K. Kinami, Y.-X. Li, G. W. J. Fleetd and C.-Y. Yu, Org. Biomol. Chem., 2016, 14, 4885 RSC;
(f) A. Gil, F. Albericio and M. Álvarez, Chem. Rev., 2017, 117, 8420 CrossRef CAS PubMed;
(g) B. Mi and R. E. Maleczka, Org. Lett., 2001, 3, 1491 CrossRef CAS PubMed;
(h) D. K. Mohapatra, P. P. Das, M. R. Pattanayak, G. Gayatri, G. N. Sastry and J. S. Yadav, Eur. J. Org. Chem., 2010, 4775 CrossRef CAS;
(i) B. Wang, Y. Xie, Q. Yang, G. Zhang and Z. Gu, Org. Lett., 2016, 18, 5388 CrossRef CAS PubMed.
-
(a) H. Jin, J.-I. Uenishi, W. J. Christ and Y. Kishi, J. Am. Chem. Soc., 1986, 108, 5644 CrossRef CAS;
(b) K. Takai, M. Tagashira, T. Kuroda, K. Oshima, K. Utimoto and H. Nozaki, J. Am. Chem. Soc., 1986, 108, 6048 CrossRef CAS PubMed ; For reviews see;
(c) A. Fürstner, Chem. Rev., 1999, 99, 991 CrossRef;
(d) L. A. Wessjohann and G. Scheid, Synthesis, 1999, 1 CrossRef CAS;
(e) A. S. K. Hashmi, J. Prakt. Chem., 1996, 338, 491 CrossRef;
(f) A. Fürstner, Chem.–Eur. J., 1998, 4, 567 CrossRef.
-
(a) T. Hiyama, Y. Okude, K. Kimura and H. Nozaki, Bull. Chem. Soc. Jpn., 1982, 55, 561 CrossRef CAS;
(b) T. Hiyama, K. Kimura and H. Nozaki, Tetrahedron Lett., 1981, 22, 1037 CrossRef CAS;
(c) K. Takai, K. Kimura, T. Hiyama and H. Nozaki, Tetrahedron Lett., 1983, 24, 5281 CrossRef CAS.
-
(a) A. Fürstner and N. Shi, J. Am. Chem. Soc., 1996, 118, 2533 CrossRef;
(b) A. Fürstner and N. Shi, J. Am. Chem. Soc., 1996, 118, 12349 CrossRef.
-
(a) M. Bandini, P. G. Cozzi, P. Melchiorre and A. Umani-Ronchi, Angew. Chem, 1999, 111, 3558 (Angew. Chem. Int. Ed., 1999, 38, 3357) CrossRef;
(b) H. Choi, K. Nakajima, D. Demeke, F. Kang, H. Jun, Z. Wan and Y. Kishi, Org. Lett., 2002, 4, 4435 CrossRef CAS PubMed;
(c) A. Berkessel, D. Menche, C. A. Sklorz, M. Schroder and I. Paterson, Angew. Chem, 2003, 115, 1062 (Angew. Chem. Int. Ed, 2003, 42, 1032) CrossRef;
(d) M. Inoue, T. Suzuki and M. Nakada, J. Am. Chem. Soc., 2003, 125, 1140 CrossRef CAS PubMed;
(e) K. Namba and Y. Kishi, Org. Lett., 2004, 6, 5031 CrossRef CAS PubMed;
(f) M. Kurosu, M. Lin and Y. Kishi, J. Am. Chem. Soc., 2004, 126, 12248 CrossRef CAS PubMed;
(g) H. S. Schrekker, K. Micskei, C. Hajdu, T. Patonay, M. W. G. de Bolster and L. A. Wessjohann, Adv. Synth. Catal., 2004, 346, 731 CrossRef CAS;
(h) J. Lee, J. J. Miller, S. S. Hamilton and S. S. Sigman, Org. Lett., 2005, 7, 1837 CrossRef CAS PubMed;
(i) G. Xia and H. Yamamoto, J. Am. Chem. Soc., 2006, 128, 2554 CrossRef CAS PubMed;
(j) H. A. McManus, P. G. Cozzi and P. J. Guiry, Adv. Synth. Catal., 2006, 348, 551 CrossRef CAS;
(k) J. Miller and M. S. Sigman, J. Am. Chem. Soc., 2007, 129, 2752 CrossRef CAS PubMed;
(l) G. C. Hargaden and P. I. Guiry, Adv. Synth. Catal., 2007, 349, 2407 CrossRef CAS;
(m) G. C. Hargaden, T. P. O'Sullivan and P. J. Guiry, Org. Biomol. Chem., 2008, 6, 562 RSC;
(n) X.-R. Huang, X.-H. Pan, G.-H. Lee and C. Chen, Adv. Synth. Catal., 2011, 353, 1949 CrossRef CAS;
(o) R.-Y. Chen, A. P. Dhondge, G. H. Lee and C. Chen, Adv. Synth. Catal., 2015, 357, 961 CrossRef CAS.
- T. Ohzeki and K. Mori, Biosci., Biotechnol., Biochem., 2003, 67, 2584 CrossRef CAS.
- J. D. Hall, N. W. Duncan-Gould, N. A. Siddiqi, J. N. Kelly, L. A. Hoeferlin, S. J. Morrison and J. K. Wyatt, Bioorg. Med. Chem., 2005, 13, 1409 CrossRef CAS PubMed.
Footnote |
† Electronic supplementary information (ESI) available. See DOI: 10.1039/c8ra09575b |
|
This journal is © The Royal Society of Chemistry 2018 |
Click here to see how this site uses Cookies. View our privacy policy here.