DOI:
10.1039/C8RA08153K
(Paper)
RSC Adv., 2018,
8, 35850-35854
Rapid and visual readout of vitamin B1 based on the electrostatic interaction induced aggregation of gold nanoparticles†
Received
1st October 2018
, Accepted 11th October 2018
First published on 22nd October 2018
Abstract
In this work, a simple and rapid colorimetric assay for the quantitative detection of vitamin B1 (VB1) has been fabricated based on citrate-stabilized gold nanoparticles (AuNPs). The UV-Vis spectra of AuNPs varied and the relative color changed from red to purple with the sequential addition of VB1. The characterization results of AuNPs with and without the addition of VB1 confirmed that the observed phenomena were attributed to the aggregation of AuNPs induced by VB1 through electrostatic interaction. The assay was rapid and sensitive to VB1 with a detection limit of 10.9 nM ranging from 30 nM to 650 nM in 15 min. Meanwhile, the developed assay displayed excellent selectivity to VB1 since AuNPs showed negligible response to common metal ions and biological molecules. Moreover, the feasibility for the quantitative detection of VB1 in tablets and human urine samples has also been demonstrated.
Introduction
Vitamin B1 (VB1), also named as thiamine, contains an aminopyrimidine ring and a thiazole ring with methyl and hydroxyethyl side chains linked by a methylene bridge. As is known, VB1 is an essential water-soluble vitamin and natural nutrient present in our dairy foods1 with the average daily intake of 0.5–1 mg.2 It plays a crucial role in the metabolic processes of carbohydrate, amino acids and lipids in living systems.3,4 Additionally, as a biologically and pharmaceutically important compound, VB1 is necessary to maintain the proper functioning of the heart, nerves and cardiovascular system.5 VB1 deficiency causes numerous deleterious effects on human beings. Most notably, VB1 deficiency is responsible for beriberi (a chronic neurological and cardiovascular disease)6 and Wernicke–Korsakoff syndrome (caused brain abnormalities)7 due to malnutrition and chronic alcoholism. Moreover, the abnormal level of VB1 has also been implicated in Alzheimer's disease.8 Results from Ke's work indicated that VB1 malnutrition was associated with depression among older Chinese adults.9 Therefore, it is highly crucial to explore a simple, fast and accurate method to detect and monitor the levels of VB1 in these patients to promote a quick recovery.
Up to now, various methods have been employed to assay VB1, such as high performance liquid chromatography (HPLC),10 chemiluminescence (CL) method,11 absorption spectrometric assay,12,13 potentiometric assay,14 fluorimetry,15–20 resonance Rayleigh scattering spectral method21 and so on. Among these analytical techniques, the problems like inevitable use of organic solvents in HPLC and CL, poor reproducibility of potentiometric assay, expensive instruments for resonance Rayleigh scattering spectral method, special competitive reaction between VB1 and fluorescent materials with the metal ions in some extent limited their wide applications. Absorption spectrometric assay, by contrast, has aroused much attention due to its excellent attributes including simple and rapid operations without any complicated instruments, high reproducibility and low-cost and some even can be observed with the naked eye. Therefore, colorimetric assays with the aid of ultraviolet absorption spectra have aroused much attention from the researchers.
Gold nanoparticles (AuNPs) endow a tremendous extinction coefficient with several orders of magnitude greater than those for traditional organic chromophores,22 which promises the high sensitivity for AuNPs based methods. Moreover, the localized surface plasmon resonance (LSPR) of AuNPs has close relationship with their size, shape, composition, interparticle distance and the dielectric constant of their surrounding medium.23 And the shorten interparticle distance will cause a redshift in the LSPR band(s) with an increase in intensity and an observable visualization of color change from burgundy for individual AuNPs to blue for aggregated ones. Meanwhile, colorimetric assays exhibit excellent analytical performances in terms of simple operation, fast response and convenience without any expensive or complex instrumentation. Taking in the above objectives into consideration, colorimetric assays based on the mechanism that analytes induce dispersed AuNPs to aggregated ones have been proposed for the situ monitor of many different analytes in environmental and biological fields.11,24–28
Herein, we proposed a rapid, simple, highly sensitive and selective assay for the colorimetric detection of VB1 with citrate-stabilized AuNPs as a sensing probe. Not only a new absorption band appeared in the long wavelength field, but also an obvious and rapid color change from burgundy for individual AuNPs to purple for aggregated ones with the addition of VB1. The mechanism for the phenomena has been discussed with the aid of different characterization results and was attributed to the electrostatic interaction between AuNPs and VB1. The proposed method was rapid and highly sensitive, which could detect VB1 with the detection limit of 10.9 nM in the range of 30–650 nM within 15 min. Meanwhile, common ions and small biological molecules displayed negligible effect on the detection of VB1 indicating the high selectivity of the assay. Furthermore, the proposed colorimetric assay also has applied to the VB1 detection in tablets and human urine samples to prove its practicability.
Experimental
Materials and reagents
HAuCl4, sodium citrate, H3BO3, Na2B4O7, glucose, vitamin B3 (VB3) and folic acid (FA) were purchased from Shanghai Chemical Reagent Co., Ltd. (Shanghai, China). Vitamin B1 (VB1), ascorbic acid (Vc), tryptophan (Trp), dopamine (DA), uric acid (UA) were purchased from Aladdin Reagent Co., Ltd. (Shanghai, China). Vitamin B2 (VB2) was from Sinopharm Chemical Reagent Co., Ltd. (Shanghai, China). Vitamin B6 (VB6) was from Thermo Fisher Scientific Inc. (Fair Lawn, NJ, USA). All the other chemicals were of analytical grade except that VB2, VB3, FA and VB6 were biological reagents. Cation stock solutions used in this experiment were prepared from their chloride or nitrate salts. Cellulose membrane dialysis tubing (retained molecular weight: 1000 Da) was purchased from Sangon Biotech Co., Ltd (Shanghai, China). Ultrapure water (18.2 MΩ) from a Laboratory Ultrapure Water System (Kertone Water Treatment Co., Ltd, China) was used throughout the experiments.
Apparatus
Absorption spectra were obtained by a UV2600 spectrophotometer (Shimadzu, China) with a 1 cm quartz cell. Transmission electron microscopy (TEM) measurements were performed on a TECNAI F-20 electron microscope (JOEL 2100F, Netherlands) at an accelerating voltage of 200 kV. Fourier transform infrared (FTIR) spectra in the range of 400–4000 cm−1 were recorded on a Nicolet AVATAR 360 spectrophotometer. Zeta potential was detected using a Nano-ZS (Malvern Instruments, UK).
Synthesis of AuNPs
All the glassware was cleaned in a bath of freshly prepared aqua regia with volume ratio of HNO3
:
HCl to be 3
:
1, thoroughly rinsed with water, and dried in air. The AuNPs were prepared by employing sodium citrate as a stabilizer capped on the surface of AuNPs according to the previous reports.29 Briefly, in a 250 mL round-bottomed flask equipped with a condenser, 100 mL 1 mM HAuCl4 solution was added and heated to rolling boil with vigorous stirring. Then, 10 mL 38.8 mM sodium citrate was quickly added to the aboil solution and the mixture was kept boiling for another 30 min with sequential stirring. The color of mixed solution gradually changed from buff to colorless, then rapidly to grey and finally to burgundy, indicating the formation of AuNPs. Then the heating mantle was removed and let the solution cool to room temperature. At last, the AuNPs stock was obtained by filtering the solution through 0.22 μm membrane filter to remove the precipitate and stored in a refrigerator at 4 °C for future use.
Analytical procedure of VB1 detection
Typically, 200 μL H3BO3–Na2B4O7 buffer solution (10 mM, pH 7.0) was mixed with 400 μL AuNPs, and then different amounts of VB1 were added into the mixture. The mixed solution was diluted to 2 mL with water. The absorption spectra of the mixed solution were implemented in the range of 400 nm to 890 nm after reacting for 15 min at room temperature. The absorbances at the wavelength of 523 nm and 710 nm were recorded. The absorbance variation ΔA710 nm/A523 nm (= (A710 nm/A523 nm)with VB1 − (A710 nm/A523 nm)without VB1) was calculated. Meanwhile, the color changes were also recorded with photos. All experiments were performed in triplicate at room temperature.
Real sample analysis
According to the previous work,17 seven pharmaceutical VB1 tablets produced by Huazhong Pharmaceutical Co., Ltd. had been grinded into powder. Then 0.3898 g powder was dissolved with 10 mL water and sonicated for 20 min. Then the solution was centrifuged at a speed of 4000 rpm for 10 min to remove the precipitate. Finally, 2 mL supernatant was filtered with 0.22 μm membrane filter, collected and diluted to 100 mL as the stock solution.
Human urine samples were obtained from healthy adult volunteers at Fujian Agriculture and Forestry University Hospital (informed consent was obtained from all human subjects). According to the previous report,30 the urine samples were centrifuged at 8000 rpm for 10 min to eliminate the insoluble substance or any protein interference. Then, the supernatant was filtered with 0.22 μm membrane filter, and the filter were diluted 100-fold with ultrapure water for further analysis. All the spiked samples were prepared by adding different amounts of VB1 solutions to the diluted samples and analyzed according the experimental procedure described in section “Analytical procedure of VB1 detection”.
Results and discussion
UV-Vis absorption spectra of AuNPs-VB1 system
As illustrated in Fig. 1a and inserted photo in Fig. 1b, the color of dispersed AuNPs changed from burgundy to purple as VB1 was added into AuNPs. According to the previous report, the color of AuNPs solution changing from burgundy to purple or blue even to gray is due to the aggregation of AuNPs induced by the analytes.23 To investigate the reason for the phenomena, the UV-Vis spectra for the AuNPs in the absence and in the presence of VB1 have been scanned. As shown in Fig. 1b, the obtained AuNPs exhibited a strong LSPR band in the range of 450–850 nm with a characteristic peak at 523 nm in H3BO3–Na2B4O7 buffer solution (Fig. 1b, curve b). When 300 nM VB1 was added, the intensity of the absorption peak at 523 nm gradually decreased and a new absorption band in the range of 600–890 nm appeared with the intensity enhancing (Fig. 1b, curve c). In this work, it was thought that the above observations were attributed to the aggregation of AuNPs induced by the electrostatic interactions between negatively charged AuNPs due to the citrate capping on their surface and positively charged VB1 molecules under the experimental conditions.13,17
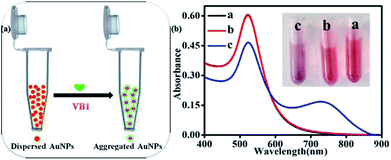 |
| Fig. 1 (a) Schematic illustration for colorimetric detection of VB1 with AuNPs; (b) UV-Vis absorption spectra of AuNPs-VB1 system (inset: relative color photos for each curve. The contents for each curve were as follows: (a) AuNPs, (b) AuNPs + H3BO3 − Na2B4O7, (c) AuNPs + H3BO3 − Na2B4O7 + VB1. The usage for each substance: 400 μL AuNPs, 200 μL 10 mM pH = 7 H3BO3–Na2B4O7 buffer solution and 60 μL 10 μM VB1). | |
Mechanism studies for the colorimetric detection of VB1 with AuNPs
In order to confirm the proposed assay mechanism, many characterizations have been carried out. Firstly, the size and morphologies of AuNPs in the absence and in the presence of VB1 had been explored with TEM. The TEM images displayed that AuNPs without the addition of VB1 were well dispersed (Fig. 2a) and roughly spherical in the range of 11–16 nm (inset in Fig. 2a) with an average size of 13.5 nm, while the obvious aggregation of AuNPs formed upon the addition of 300 nM VB1 (Fig. 2b). Moreover, the zeta potential of AuNPs was −46.7 eV indicating that AuNPs bear a negative charge due to the capping of citrate on their surface (Fig. S1a†). While the zeta potential decreased to −14.1 eV upon the addition of 300 nM VB1 into AuNPs (Fig. S1b†).
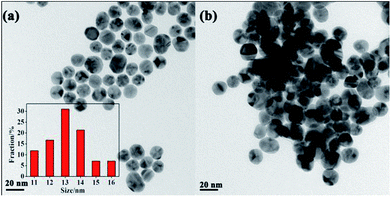 |
| Fig. 2 TEM images of AuNPs (a) in the absence and (b) in the presence of 300 nM VB1. | |
Furthermore, the FTIR spectra showed that the characteristic peaks for C
N+ (1665 cm−1), C
N (pyrimidine ring) (1618 cm−1) and NH2 stretching frequencies (3438 cm−1) for VB1 changed with notable decreasing intensity in the AuNPs-VB1 system (Fig. 3), which was similar to the previous report that the aggregation of glutathione modified silver nanoparticles come out with the introduction of VB1.13 Taking the above results into consideration, it came to the conclusion that the colorimetric detection of VB1 depended on the prevalence of electrostatic interaction between AuNPs and VB1.
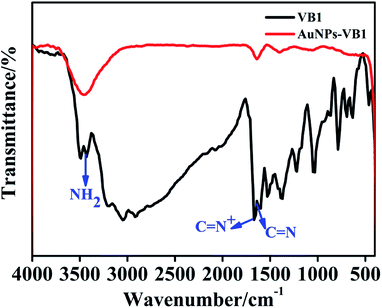 |
| Fig. 3 FT-IR spectra of pristine VB1 and AuNPs-VB1 system. | |
Optimization of experimental conditions
Effect of pH value. It has reported previously that the LSPR of AuNPs was close to the dielectric constant of their surrounding medium,23 the detection of VB1 was carried out in different pH values by adjusting with H3BO3–Na4B2O7 buffer solution. Seen from Fig. 4, the pH played great effect on the detection of VB1 with AuNPs. When pH was lower than 7.0, the ΔA710 nm/A523 nm gradually increased with the enhancement of pH, while ΔA710 nm/A523 nm gradually decreased as pH increased from 7.0 to 11.0. Since colorimetric detection of VB1 with AuNPs was realized mainly based on the electrostatic interaction between AuNPs and VB1, the ΔA710 nm/A523 nm closely related to the charge of AuNPs and VB1. On the one hand, the protonation of citrate reduced the negative charge on the surface of AuNPs when the pH was too low. On the other hand, the structure and charge of VB1 varies with the pH values.14,31 As depicted in Fig. 5, VB1 is positively charged on both the pyrimidine N1 nitrogen (pKa1 ≈ 4.8) and thiazole N3 nitrogen at low pH, and the VB1 presents with a cation that a positive charge appears on the thiazole N3 nitrogen at physiological pH. Finally, the VB1 passes through an uncharged pseudobase intermediate to display in negatively charged thiol form (pKa2 ≈ 9.2) with the pH further increasing. As a result, the same charge of AuNPs and VB1 repelled each other as pH was higher than 7. Therefore, pH = 7.0 was chosen as the experimental acidity in the following study.
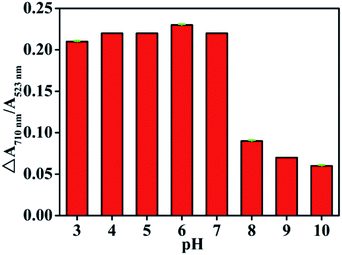 |
| Fig. 4 Effect of pH on the colorimetric detection of VB1 with AuNPs. | |
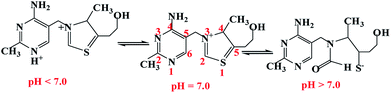 |
| Fig. 5 pH-dependent forms of VB1 from cationic forms to the pseudobase and thiol forms.14,31 | |
Stability of the detection system. To investigate the stability of AuNPs for the detection of VB1, the response kinetics of AuNPs towards to VB1 were investigated for different times. As shown in Fig. S2,† the absorbance of AuNPs was quickly reduced within 15 min upon the addition of VB1 and remained constant when the reaction time prolonged to 30 min. These results indicated that the response rate was fast and that the absorbance could remained stable for a long time, promising the feasibility for rapid and stable detection of VB1.
Interference and selectivity. To evaluate the selectivity of the colorimetric response of AuNPs toward to VB1, the interferences from metal ions (Ag+, Hg2+, Cu2+, Co2+, Ni2+, Pb2+, Cd2+, Fe2+ and Fe3+), the relative vitamin B complexes (VB2, VB3, FA, Vc and VB6) and common biological small molecules (Trp, DA, UA and glucose) were studied. It was delighted to find that there was negligible absorbance change in the metal ions (Fig. 6a and c) and interfering molecules (Fig. 6b and d), indicating the excellent selectivity of the proposed assay.
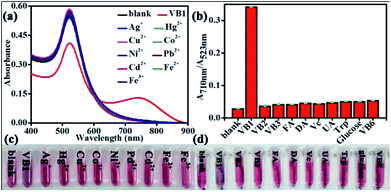 |
| Fig. 6 Response of AuNPs to different (a) metal ions and (b) biological molecules; photographic images of color changes observed from AuNPs in different (c) metal ions and (d) biological molecules. | |
Analytical performances of the VB1 detection. To explore the sensitivity of the VB1 detection, the A710 nm/A523 nm evolution of AuNPs in the absence of different concentrations of VB1 was investigated. As shown in Fig. 7, the A710 nm/A523 nm gradually increased up the addition of VB1 ranging from 30 nM to 2000 nM with the obvious color change observed by naked eyes. Meanwhile, the ΔA710 nm/A523 nm was in a distinct linear relationship with the VB1 concentration in the range 30–650 nM with a linear regression equation of ΔA710 nm/A523 nm = 0.0011CVB1 − 0.039, a correlation coefficient (R) of 0.9912 and a detection limit of 10.9 nM. And obvious color change could be observed with naked eyes when 75 nM VB1 was added into AuNPs solution. It was pleased to find that the detection limit was comparable with or superior to those obtained from other sensitive methods (Table S1†). Moreover, the proposed assay was rapid, simple and could be observed with naked eyes.
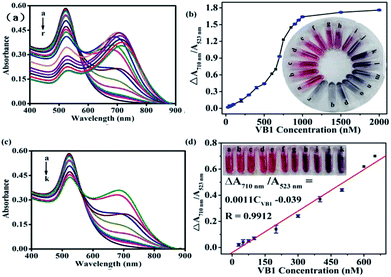 |
| Fig. 7 (a) UV-Vis spectra of AuNPs and (b) relationship of ΔA710 nm/A523 nm with different concentrations of VB1 under optimum experimental conditions (inset: relative photographs of AuNPs in the presence of VB1 with the concentration from a to r to be 0, 30, 50, 75, 100, 200, 300, 400, 500, 600, 650, 700, 750, 800, 950, 1000, 1500 and 2000 nM, respectively); (c) UV-Vis spectra of AuNPs and (d) linear relationship of ΔA710 nm/A523 nm with the concentrations of VB1 in the range of 30–650 nM (inset: relative photographs of AuNPs in the presence of VB1 with the concentration from a to k to be 0, 30, 50, 75, 100, 200, 300, 400, 500, 600, 650 nM, respectively). | |
Detecting VB1 in pharmaceutical tablets and urine samples. To demonstrate the practicability of the proposed method for the detection of VB1 in real samples, the proposed method has been applied to detection VB in pharmaceutical VB1 tablets and human urine samples. As presented in Table 1, the recoveries were in the range of 95.2–97.4% for VB1 tablets and 99.3–100.3% for urine samples, indicating the applicability of this colorimetric assay for VB1 detection in real samples.
Table 1 Analytical results of VB1 in real samples (n = 3)
Samples |
Detected/nM |
Spiked/nM |
Found/nM |
Recovery/% |
RSD/% |
VB1 tablets |
402.5 |
50 |
450.1 |
95.2 |
0.2 |
402.5 |
100 |
451.2 |
97.4 |
0.3 |
Human urine samples |
0 |
200 |
198.6 |
99.3 |
0.3 |
0 |
400 |
401.3 |
100.3 |
0.4 |
Conclusions
In this work, a rapid, simple, sensitive and selective colorimetric assay has been proposed for the detection of VB1 based on the aggregation of the AuNPs through electrostatic interaction between AuNPs and VB1. The assay was without any addition of any other metal salts or complex instrumentation. The proposed assay has been successfully applied to the quantitative detection of VB1 in urine samples and tablets, promising the potential application for monitoring VB1 related to biological filed.
Conflicts of interest
There are no conflicts to declare.
Acknowledgements
This research work was financially supported by the Nature Science Foundation from Science and Technology Department of Fujian Province (No. 2018J05013) and Education Research Project for Young and Middle-Aged Teachers of Fujian Province (JT180136), which were gratefully acknowledged.
References
- A. B. Tayade, P. Dhar, J. Kumar, M. Sharma, O. P. Chaurasia and R. B. Srivastava, Anal. Chim. Acta, 2013, 789, 65–73 CrossRef CAS PubMed.
- S. Shankar and S. A. John, RSC Adv., 2015, 5, 49920–49925 RSC.
- S. Manzetti, J. Zhang and D. van der Spoel, Biochemistry, 2014, 53, 821–835 CrossRef CAS PubMed.
- R. K. Gupta, S. K. Yadav, V. A. Saraswat, M. Rangan, A. Srivastava, A. Yadav, R. Trivedi, S. K. Yachha and R. K. Rathore, Clin. Nutr., 2012, 31, 422–428 CrossRef CAS PubMed.
- G. E. Gibson and J. P. Blass, Antioxid. Redox Signaling, 2007, 9, 1605–1619 CrossRef CAS PubMed.
- K. C. Whitfield, G. Smith, C. Chamnan, C. D. Karakochuk, P. Sophonneary, K. Kuong, M. A. Dijkhuizen, R. Hong, J. Berger, T. J. Green and F. T. Wieringa, PLoS Neglected Trop. Dis., 2017, 11, e0005814 CrossRef PubMed.
- E. Day, P. W. Bentham, R. Callaghan, T. Kuruvilla and S. George, Cochrane Database Syst. Rev., 2013, 7, CD004033 Search PubMed.
- G. E. Gibson, J. A. Hirsch, R. T. Cirio, B. D. Jordan, P. Fonzetti and J. Elder, Mol. Cell. Neurosci., 2013, 55, 17–25 CrossRef CAS PubMed.
- G. Zhang, H. Ding, H. Chen, X. Ye, H. Li, X. Lin and Z. Ke, J. Nutr., 2013, 143, 53–58 CrossRef CAS PubMed.
- K. Taguchi, E. Fukusaki and T. Bamba, J. Chromatogr. A, 2014, 1362, 270–277 CrossRef CAS PubMed.
- M. Kamruzzaman, A. M. Alam, S. H. Lee and T. D. Dang, Sens. Actuators, B, 2013, 185, 301–308 CrossRef CAS.
- J. Zhu, S. Liu, Z. Liu, Y. Li, M. Qiao and X. Hu, RSC Adv., 2014, 4, 5990–5994 RSC.
- R. Rajamanikandan and M. Ilanchelian, Sens. Actuators, B, 2017, 244, 380–386 CrossRef CAS.
- G. Perez-Caballero, J. F. Perez-Arevalo, E. A. Morales-Hipolito, M. E. Carbajal-Arenas and A. Rojas-Hernandez, J. Mex. Chem. Soc., 2011, 55, 126–131 CAS.
- A. Bayandori Moghaddam, F. Gudarzy and Y. Ganjkhanlou, J. Fluoresc., 2014, 24, 1025–1030 CrossRef CAS PubMed.
- Y. Li, P. Wang, X. Wang, M. Cao, Y. Xia, C. Cao, M. Liu and C. Zhu, Microchim. Acta, 2010, 169, 65–71 CrossRef CAS.
- Y. Luo, H. Miao and X. Yang, Talanta, 2015, 144, 488–495 CrossRef CAS PubMed.
- S. Shankar and S. A. John, RSC Adv., 2015, 5, 49920–49925 RSC.
- R. Purbia and S. Paria, Biosens. Bioelectron., 2016, 79, 467–475 CrossRef CAS PubMed.
- F. Nemati, R. Zare-Dorabei, M. Hosseini and M. R. Ganjali, Sens. Actuators, B, 2017, 255, 2078–2085 CrossRef.
- S. Liu, Y. Chen, Z. Liu, X. Hu and F. Wang, Microchim. Acta, 2006, 154, 87–93 CrossRef CAS.
- K. W. Huang, C. J. Yu and W. L. Tseng, Biosens. Bioelectron., 2010, 25, 984–989 CrossRef CAS PubMed.
- D. Vilela, M. C. Gonzalez and A. Escarpa, Anal. Chim. Acta, 2012, 751, 24–43 CrossRef CAS PubMed.
- N. Y. Chen, H. Y. Liu, Y. J. Zhang, Z. W. Zhou, W. P. Fan, G. C. Yu, Z. Y. She and A. G. Wu, Sens. Actuators, B, 2018, 255, 3093–3101 CrossRef CAS.
- Y. M. Leng, Y. L. Li, A. Gong, Z. Y. Shen, L. Chen and A. G. Wu, Langmuir, 2013, 29, 7591–7599 CrossRef CAS PubMed.
- Y. L. Li, Y. M. Leng, Y. J. Zhang, T. H. Li, Z. Y. Shen and A. G. Wu, Sens. Actuators, B, 2014, 200, 140–146 CrossRef CAS.
- T. H. Li, Y. L Lia, Y. J. Zhang, C. Dong, Z. Y. Shen and A. g. Wu, Analyst, 2015, 140, 1076–1081 RSC.
- G. H. Wu, C. Dong, Y. L. Li, Z. Q. Wang, Y. X. Gao, Z. Y. Shen and A. G. Wu, RSC Adv., 2015, 5, 20595–20602 RSC.
- K. C. Grabar, R. G. Freeman, M. B. Hommer and M. J. Natan, Anal. Chem., 1995, 67, 735–743 CrossRef CAS.
- P. K. Brahman, R. A. Dar and K. S. Pitre, Sens. Actuators, B, 2013, 117, 807–812 CrossRef.
- K. A. Edwards, N. Tu-Maung, K. Cheng, B. Wang, A. J. Baeumner and C. E. Kraft, ChemistryOpen, 2017, 6, 178–191 CrossRef CAS PubMed.
Footnote |
† Electronic supplementary information (ESI) available. See DOI: 10.1039/c8ra08153k |
|
This journal is © The Royal Society of Chemistry 2018 |
Click here to see how this site uses Cookies. View our privacy policy here.