DOI:
10.1039/C8RA07858K
(Paper)
RSC Adv., 2018,
8, 41858-41871
Renoprotective effect of JinQi-JiangTang tablet on high-fat diet and low-dose streptozotocin-induced type 2 diabetic rats
Received
21st September 2018
, Accepted 6th November 2018
First published on 14th December 2018
Abstract
JinQi-JiangTang tablet (JQ), a traditional Chinese patent medicine, have been commonly applied to clinical therapy in type 2 diabetic patients. The present study was undertaken to investigate the renoprotective effect of JQ on type 2 diabetic rats. The type 2 diabetic rat model was successfully induced by a high-fat and high-sugar diet combined with a single low-dose of streptozotocin. Intervention with JQ could significantly diminish the body weight loss, reduce the levels of fasting blood glucose, 24 hour urinary protein, blood urea nitrogen and serum creatinine in STZ-induced diabetic rats. JQ improved the creatinine clearance in diabetic rats. What's more, the levels of total cholesterol, triglyceride and low-density lipoprotein cholesterol were markedly reduced following JQ treatment, while the level of high-density lipoprotein cholesterol was elevated. Moreover, JQ significantly improved the activity of superoxide dismutase, catalase and glutathione peroxidase, whereas decreased the level of lipid peroxidation malondialdehyde in renal tissue of diabetic rats. Furthermore, immunohistochemical analysis showed that JQ significantly downregulated the expression of Bax, Caspase-3 and Cytochrome c and upregulated Bcl-2 protein expression in the renal tissue of diabetic rats, which was considered as the major pathogeneses of apoptosis. These data demonstrated that JQ exhibited a renoprotective effect through blood glucose control, alleviating lipid metabolism, anti-oxidative stress and anti-apoptosis activities.
Introduction
Type 2 diabetes mellitus (T2DM) is a metabolic disorder caused by peripheral insulin resistance accompanied with hyperglycemia and hyperlipidemia. According to the International Diabetes Federation conducted in 2017, there were more than 114 million adults with diabetes mellitus and more than 212 million diagnosed with prediabetes in China.1 Diabetic nephropathy is one of the most serious microvascular complications of T2DM, which is the principle cause of end-stage renal disease all over the world.2 About 30–40% of patients who diagnosed with T2DM developed diabetic nephropathy, which was characterized as increased permeability to macromolecules, proteinuria, glomerular basement membrane thickening, glomerulosclerosis, glomerular hypertrophy, podocyte injury and progressive renal failure.3–5 This is a warning sign for us to be vigilant not only for critical care but also for concerning the risk factors of diabetic nephropathy. Therefore, intervention for preventing and retarding the development of diabetic nephropathy is not only a medical concern but also a social problem.
The pathogenesis of diabetic nephropathy is complex, multifactorial and involves many signal pathways. Despite much work being done, the underlying molecular mechanism is still not fully clear. However, it has been widely accepted that hyperglycemia-induced oxidative stress and apoptosis is one of the major contributing factors in the pathophysiology of this disease.6 It has been reported that persistent hyperglycemia-induced increased production of reactive oxygen species (ROS) can activate various pathways of oxidative stress, growth factors and liberate numerous cytokines, which in turn lead to cell membrane injury, mitochondrial dysfunction and renal damage.7 What's more, chronic accumulation of ROS can easily oxidize lipids, protein, carbohydrate and DNA leading to the inactivation of endogenous antioxidant, production of peroxynitrite, cellular structure damage, altering gene expression and apoptosis.8,9 Therefore, alleviating the hyperglycemia-induced oxidative stress and apoptosis can prevent initiation and promotion of diabetic nephropathy.
Traditional Chinese medicine and Chinese herbals have been widely used for the management of T2DM and its complications for thousands of years.10 JinQi-JiangTang tablet (JQ), a well-known traditional Chinese patent medicine formula, has been approved by the State Food and Drug Administration of China (state medical license No. Z10920027) for the treatment of mild and moderate T2DM (called Xiaoke disease in traditional Chinese medical science) in clinical.11 It has been used to improve insulin resistance and alleviate lipid metabolism in type 2 diabetic patients.12 JQ is consisted of Coptis chinensis (rhizome of Ranunculaceae Coptis chinensis Franch), Astragalus membranaceus (root of Leguminosae Astragalus membranaceus Moench), and Lonicera japonica (flower buds of Caprifoliaceae Lonicera japonica Thunb). The Coptis chinensis, Astragalus membranaceus and Lonicera japonica have been widely used in China and other Asia countries. It has been documented that chronic administration of berberine, a component extracted from Coptis chinensis, may ameliorate renal injury caused by T2DM through regulating the expression of β-arrestins, intercellular adhesion molecule-1 and vascular cell adhesion molecule-1.13 Experimental research has reported that there are several alkaloids and phenolic acids in JQ and the main bioactive compounds include palmatine, epiberberine, chlorogenic acid et al.14 The previous study has indicated that palmatine exerted renoprotective effects against streptozotocin-induced diabetic nephropathy rats via reducing the oxidative stress.15 Therefore, we hypothesized that JQ has a renoprotective activity. In this present study, we aimed to evaluate the renoprotective effect of JQ and detect its underlying mechanism from two aspects, the levels of hyperglycemia induced oxidative stress and apoptosis in the kidney of rats with T2DM.
Materials and methods
Chemicals and reagents
JinQi-JiangTang tablet was donated by Tianjin Zhongxin Pharmaceutical Group Co., Ltd (Tianjin, China). High-fat and high-sugar diet (HFD) (10% lard oil, 20% sucrose, 1% choline chloride, 2.5% cholesterol and 66.5% normal diet) was obtained from Beijing HFK bioscience Co., Ltd (Beijing, China). Streptozotocin (STZ) was produced by Sigma-Aldrich Company (St. Louis, USA). Sodium carboxymethylcellulose (CMC-Na) was obtained from Tianjin Fengchuan Chemical Reagent Science and Technology Co., Ltd (Tianjin, China). Superoxide dismutase (SOD), malondialdehyde (MDA), catalase (CAT) and glutathione peroxidase (GSH-Px) assay kits, urinary protein quantification, blood urea nitrogen (BUN) determination and creatinine estimation kits were purchased from Nanjing Jiancheng Bioengineer Institute (Nanjing, China). Rat anti-Bax, anti-Bcl-2, anti-Caspase-3 and anti-Cytochrome c (anti-Cyt-c) antibodies were obtained from Abcam (Cambridge, UK). Biotinylated goat anti-rabbit secondary antibody and 3,3-diaminobenzidine tetrahydrochloride (DAB) were purchased from Beijing Bioss Biotechnology Co., Ltd (Beijing, China).
UPLC-Q-TOF-MS/MS analysis condition
JQ was pulverized into powder. Powder (0.5 g) was accurately weighed and sonicated extracted with 50 mL methanol for 60 min. The weight loss was made up with methanol after extraction, and the supernatant was filtered with a 0.45 μm syringe filter before Ultra Performance Liquid Chromatography (UPLC) analysis. Chemical profile analysis was performed on an Agilent 1200 series Ultra Performance Liquid Chromatography system (Agilent Technologies, USA) connected with the MicrOTOF-Q-II (Q-TOF-MS, Bruker Daltonics Inc., USA). The UPLC system equipped with a binary bump, an online degasser, an auto-sampler, and a thermostatically controlled column compartment. Chromatographic separation was performed at 30 °C on an Agilent C18 column (4.6 mm × 250 mm, 5 μm). The mobile phase consisted of water containing 0.3% formic acid (A) and acetonitrile (B) under gradient condition as follows: from 5% to 15% B at first 5 min, followed by 15–24% B during 5–8 min, 24–40% B during 8–17 min, and 40–80% B during 17–40 min. The injection volume was 5 μL and flow rate was 1.0 mL min−1. As for Q-TOF/MS analysis, it was worked using full-scan mode and the mass range was recorded from m/z 100 to 3000 both in positive mode and negative mode. The acquisition parameters of the MS detection were as follows: drying gas (N2) flow rate, 8.0 L min−1; drying gas temperature, 320 °C; nebulizer, 35 psig; capillary voltage, 3000 V; octopole radio frequency voltage, 750 V; fragmentor voltage adjusted from 120 V to 150 V; skimmer voltage 65 V. Data acquisition and operated under Bruker Compass Data Analysis software Version 4.3 (Bruker Daltonics GmbH, Bremen, Germany).
Animal experiment
Male Sprague-Dawley (SD) rats weighting 200 ± 20 g were obtained from Beijing HFK Bioscience Co., Ltd (Beijing, China). All rats were allowed free access to food and distilled water and housed in normal cages (10 rats per cage) under control laboratory condition (23 ± 2 °C with a relative humidity at 45%, 12 h light/12 h dark cycle). All experiment procedures were approved in accordance with the Animal Ethics Committees of the Faculty of Medicine, Tianjin Medical University, Tianjin, China, and performed in accordance with “Principles of Laboratory Animal Care and Use in Research” (State Council of China, 1988). After one week adaptation of rats with the environment, the rats were randomly divided into two groups: normal control group and diabetic group. The rats in diabetic group were fed with high-sugar and high-fat diet, while the rats in control group were fed with standard diet for four weeks. After four weeks later, diabetic rats were induced by a single tail vein injection of 35 mg kg−1 freshly prepared STZ solution (dissolved in 0.1 M citrate buffer, pH = 4.5). Control rats were only given the citrate buffer. 72 hours after injection of STZ, the fasting blood glucose was measured via taking a drop of blood from tail of rat. Only the rats with a fasting blood glucose level higher than 16.7 mM, and urinary total protein two times higher than in normal group were used for the next experiment procedure.
Afterward, the diabetic rats were assigned into three groups by stratified randomization, based on the blood glucose level. Experimental groups were as follows: diabetic rats treated with 0.5% CMC-Na (model group), diabetic rats treated with JQ-L (1.01 g kg−1 d−1) group and diabetic rats treated with JQ-H (4.04 g kg−1 d−1) group. Each group contained 10 rats. JQ was dissolved in 0.5% CMC-Na. Ten age-matched normal rats with the equal 0.5% CMC-Na served as control. All animals were intragastric administration once per day for six weeks. During the treatment period, all diabetic rats were maintained on high-sugar and high-fat diet. The body weight of rats was measured with an electronic weighing scale in every week from week 0 to week 6 (last day of treatment).
One day before the end of the experiment, the urine samples were collected throughout 24 hours, with each rat housed individually in a metabolic cage. Post-collection, the urine volume was detected using a volumetric cylinder. Before killing, the fasting blood glucose was detected by a digital blood glucose meter. The experimental protocol is shown in Fig. 1.
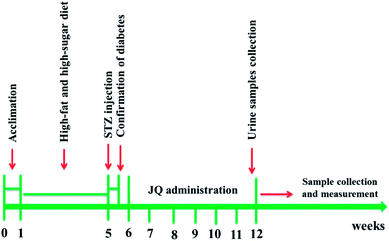 |
| Fig. 1 Schematic diagram of experimental protocol. | |
Samples collection and preparation
In order to examine the renal histopathological change, explore the underlying molecular biological mechanism and do biochemical studies, the rats were anesthetized by chloral hydrate. Blood samples were collected from arteria cruralis into non-heparinised tubes. Then the serums of samples were extracted by centrifuge at 3500 rpm for 15 min and stored at −80 °C for later analysis. After sacrificed, both kidneys were removed immediately, rinsed with isotonic saline and weighed. The left kidney was fixed in 4% paraformaldehyde for Masson staining, HE staining, periodic acid-Schiff (PAS) staining and immunohistochemistry staining, while another kidney was washed with phosphate buffer and then homogenized in a homogenizing buffer using homogenizer. Afterward, the kidney tissue homogenate was centrifuged at the speed rate of 4000 rpm for 10 min. Then the supernatant was collected and stored at −80 °C for various biochemical assays. The kidney index was calculated with the ratio of kidney weight to body weight.
Fasting blood glucose determination
Fasting blood glucose (FBG) was measured every week. The level of FBG was carried out using lateral tail vein blood samples and a digital blood glucose meter after all rats has been fasted for 10 hours (food withdrawn but water ad libitum).
Assessment of plasma lipid profile
At the end of experiment, the content of the plasma lipid metabolism, including triglyceride (TG), total cholesterol (TC), low-density lipoprotein cholesterol (LDL-C) and high-density lipoprotein cholesterol (HDL-C) were detected by using a fully Glamour 3000 Automatic Biochemical Analyzer.
Determination of oxidative stress biomarker
In this experiment, the content of MDA in renal tissue homogenates was determined by reacting with thiobarbituric acid (TBA) as an index of lipid peroxidation. The SOD activity was measured according to the nitrous acid method, which was based on the inhibition of nitrite formation from hydroxylamine in the presence of superoxide generators. The activities of CAT and GSH-Px in the renal tissue samples were detected by using commercially available colorimetric assay kits (Nanjing Jiancheng Bioengineer Institute, Nanjing, China) according to their manufacture's instruction. Bicinchoninic acid (BCA) method was used to detect the concentration of total protein in kidney tissue, following the introduction of BCA kit.
Evaluation of kidney function
Serum and urine obtained as described above were used to detect the content of BUN, serum creatinine (SCr) and urine creatinine by using standard kits obtained from Nanjing Jiancheng Bioengineer Institute (Nanjing, China), and the creatinine clearance rate (CCr) was calculated using the formula: CCr (mL min−1) = [urine creatinine (μmol L−1) × volume of urine (mL)/serum creatinine (μmol L−1)]/1440 (min).16 Moreover, the 24 hour urinary total protein concentration was measured by using coomassie brilliant blue (CBB).
Kidney histopathological examination
For histopathological examination, formalin preserved kidney tissue were processed consecutively for dehydration and clearing by using graded alcohol series and xylene, respectively. Then the dehydrated tissues were embedded in paraffin blocks. 5 μm thickness sections were cut from paraffin-embedded kidney tissue, and stained with Mayer's hematoxylin and eosin. Moreover, the tissue slices were also stained with PAS staining and Masson's trichrome staining for saccharides and collagen detection. In PAS staining, saccharides will be stained red and the nucleus will be stained blue. Collagen will be stained blue, nucleus stained dark brown, while muscle fiber stained red in Masson's trichrome. Renal pathological changes were detected by morphological analysis under an optical microscope.
Immunohistochemistry analysis
The protein expression levels of Cyt-c, Bax, Bcl-2 and Caspase-3 in kidney tissue were detected by immunochemistry. Briefly, the 4 μm thick kidney paraffin sections were deparaffinized by xylene and rehydrated with alcohol of different concentration. To retrieve antigens, the kidney sections were heated with 0.01 M citrate buffer (pH = 6.0) for 10 min, then cooled and washed by PBS. The kidney tissue slices were incubated overnight with the primary antibodies of Cyt-c (1
:
200), Bax (1
:
200), Bcl-2 (1
:
200) and Caspase-3 (1
:
200) at 4 °C, then washed thrice with PBS. After that, the sections were incubated with secondary antibody at 37 °C for 20 min, and then rinsed with PBS for three times. After washed with PBS, incubation was done with supersensitive polymerhorseradish peroxidase immunochemistry detection system. Samples were washed with PBS and reacted with diaminobenzidine for six min. Subsequently, counterstained with hematoxylin. The percentage of positive staining area in kidney tissue was calculated with Image-Pro Plus 6 software.
Statistical analysis
Data were expressed as mean ± standard error of the mean (SEM). All statistical analyses were performed by one-way analysis of variance (ANOVA), and the difference among two groups was analyzed by Dunnett's test or Fisher's protected LSD multiple comparison test using SPSS 20.0 system. The P values less than 0.05 were considered statistically significant. The graphs were plotted by using GraphPad Prism software (version 5).
Results
The UPLC-Q-TOF-MS analysis of JQ
In order to characterize the chemical constituents of JQ, an UPLC-Q-TOF-MS method was established. The typical MS total ion current profiles of JQ were presented in the positive and negative mode (Fig. 2A and C). A total of sixteen compounds (Table 1) were identified or characterized by comparing the MS fragmentation behaviors and retention time with reference standards (Fig. 2B and D) and literature information.17–21 The majority of these sixteen identified compounds of JQ (Fig. 3) have been reported as the main bioactive components of each individual herb.
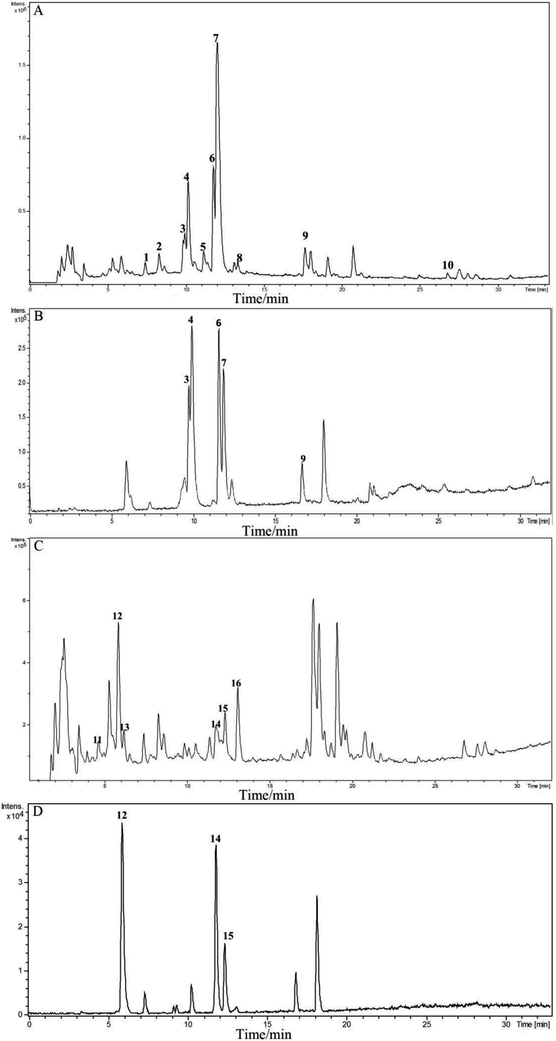 |
| Fig. 2 Chromatograms of reference standards and JQ by UPLC-Q-TOF/MS. (A) Total ion current (TIC) chromatogram of JQ in positive ion mode; (B) total ion current (TIC) chromatogram of reference standards in positive ion mode; (C) total ion current (TIC) of JQ in negative ion mode; (D) total ion current (TIC) of reference standards in negative ion mode. | |
Table 1 Chromatographic and mass spectral data of the 16 compounds of JQ analyzed by UPLC-Q-TOF-MS
No. |
tR (min) |
Identified compound |
Elemental composition |
Theoretical m/z |
Experimental m/z |
Proposed ions |
Common fragment ions/(m/z) |
Plant source |
1 |
7.4 |
Sweroside17 |
C16H22O9 |
359.1337 |
359.1182 |
[M + H]+ |
197.0718, 179.0616, 151.0562, 127.0328 |
Lonicera japonica |
2 |
8.3 |
Secoxyloganin18 |
C17H24O11 |
405.1391 |
405.1210 |
[M + H]+ |
243.0745, 225.0648, 211.0499, 193.0406, 165.0467, 151.0318, 123.0384 |
Lonicera japonica |
3 |
10.0 |
Epiberberine |
C20H18NO4 |
336.1230 |
336.1111 |
M+ |
320.0748, 308.0818 |
Coptis chinensis |
4 |
10.2 |
Coptisine |
C19H14NO4 |
320.0917 |
320.0829 |
M+ |
318.0766, 292.0821, 290.0573, 262.0829 |
Coptis chinensis |
5 |
11.2 |
Berberubine19 |
C19H16NO4 |
322.1074 |
322.0946 |
M+ |
307.0683, 292.0433, 279.0874 |
Coptis chinensis |
6 |
11.8 |
Palmatine |
C21H22NO4 |
352.1543 |
352.1452 |
M+ |
336.1048, 322.0918, 320.0795, 308.1060 |
Coptis chinensis |
7 |
12.0 |
Berberine |
C20H18NO4 |
336.1230 |
336.1148 |
M+ |
320.0764, 292.0843, 278.0811 |
Coptis chinensis |
8 |
13.4 |
13-Methylberberine20 |
C21H20NO4 |
350.1387 |
350.1242 |
M+ |
335.0989, 334.0932, 320.0781 |
Coptis chinensis |
9 |
17.7 |
Dipsacoside B |
C53H86O22 |
1075.5684 |
1075.5158 |
[M + H]+ |
943.4796, 929.4657, 913.5113, 781.4373, 767.4212, 751.4279, 635.3851, 619.3913, 603.1852, 587.3672, 473.3409, 455.3303, 441.1399, 437.3205, 409.3246, 405.1203, 325.0958, 309.1036, 295.0894, 279.0933, 163.0420, 147.0585 |
Lonicera japonica |
10 |
26.8 |
Sapindoside B21 |
C46H74O16 |
883.5050 |
883.4617 |
[M + H]+ |
751.4260, 619.3898, 473.3396, 455.3284, 437.3183, 409.3251, 279.0906, 147.0577 |
Lonicera japonica |
11 |
4.7 |
5-O-Caffeoylquinic acid18 |
C16H18O9 |
353.0878 |
353.0923 |
[M − H]− |
191.0575, 179.0375, 135.0494 |
Lonicera japonica |
12 |
5.9 |
3-O-Caffeoylquinic acid |
C16H18O9 |
353.0878 |
353.0945 |
[M − H]− |
191.0593 |
Lonicera japonica |
13 |
6.2 |
4-O-Caffeoylquinic acid18 |
C16H18O9 |
353.0878 |
353.0930 |
[M − H]− |
191.0581, 179.0379, 173.0481, 161.0353, 135.0499 |
Lonicera japonica |
14 |
11.8 |
4,5-O-Dicaffeoylquinic acid |
C25H24O12 |
515.1195 |
515.1299 |
[M − H]− |
353.0946, 335.0953, 191.0602, 179.0537, 173.0481, 161.0298.135.0471 |
Lonicera japonica |
15 |
12.3 |
3,5-O-Dicaffeoylquinic acid |
C25H24O12 |
515.1195 |
515.1296 |
[M − H]− |
353.0947, 191.0581, 179.0436, 135.0478 |
Lonicera japonica |
16 |
13.1 |
3,4-O-Dicaffeoylquinic acid18 |
C25H24O12 |
515.1195 |
515.1321 |
[M − H]− |
353.0941, 191.0579, 179.0408, 173.0487, 135.0486 |
Lonicera japonica |
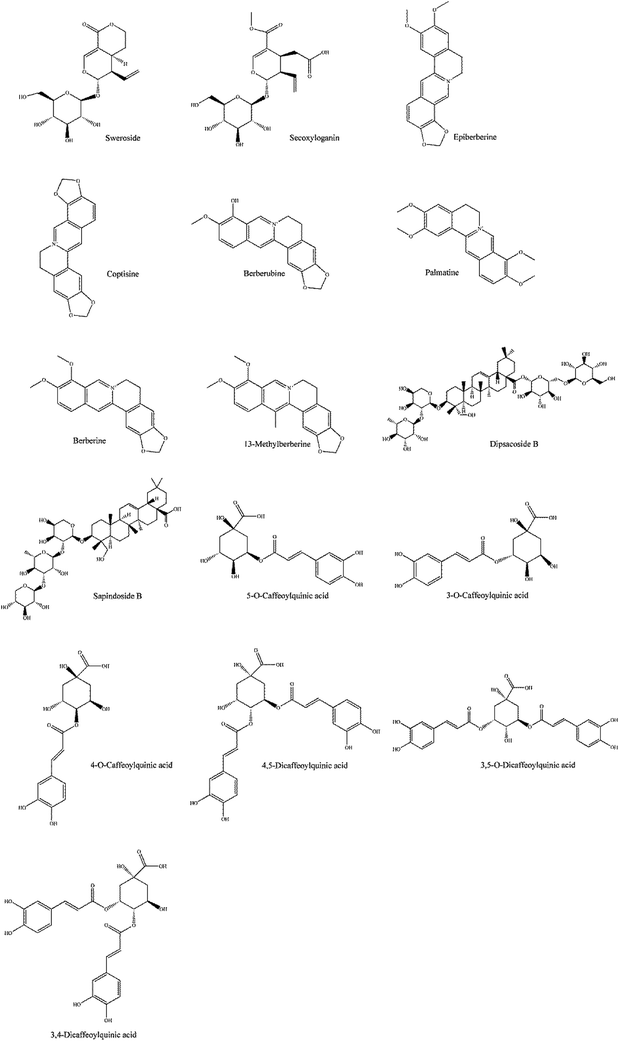 |
| Fig. 3 The chemical structure of the identified compounds in JQ. | |
The general condition of rats
During the treatment period, all rats in normal control group were in good shape, had sensitive response, glossy coat color, good mental status, normal weight increase and no uncomfortable signs were observed. However, the diabetic rats had low spirit, withered coat color, lagged in response and typical characteristics of diabetes mellitus (polyphagia, polyuria, polydipsia, piloerection and emaciated body). In all JQ-treated groups, the rats were in normal mental status, and the phenomenon of polyphagia, polyuria, polydipsia were ameliorated. As shown in Fig. 4, the diabetic rats showed a significant (P < 0.001) decrease in body weight gain during the experiment period as compared with normal control group. After six weeks treatment of JQ, high-dose JQ treated group showed a significant increase of body weight from 323 ± 20 g to 357 ± 15 g (P < 0.05) while there was no significant difference between low-dose JQ treated group and model group. The results implied that treatment with JQ could prevent the body weight loss in STZ-induced diabetic rats.
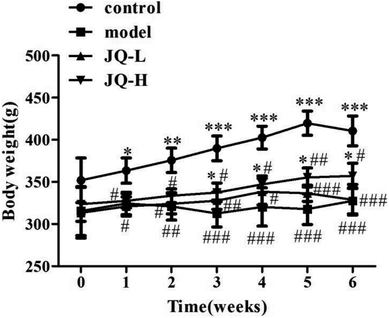 |
| Fig. 4 Effect of JQ on body weight during the treatment period. Data were expressed as mean ± SEM (n = 10, each group). *P < 0.05, **P < 0.01, ***P < 0.001, compared with model group; #P < 0.05, ##P < 0.01, ###P < 0.001, compared with normal control group. | |
Effect of JQ on fasting blood glucose in diabetic rats
The FBG level was measured in a drop of blood from the tails by using a digital blood glucose meter every week. It was found that FBG in diabetic rats was significantly higher than that in normal control group, and it was also higher than the maximum normal value of FBG (16.7 mM), indicating that the STZ-induced diabetic model was successful. After six weeks treatment of JQ, FBG in both JQ-treated groups were significantly decreased when compared with untreated diabetic rats, although there were still higher than that in control group. As shown in Fig. 5, both the dose levels of JQ markedly ameliorated the level of FBG. Interestingly, the FBG of the JQ-treated group at a dose of 4.04 g kg−1 body weight was found to be more effective. The results indicated that long term treatment of JQ helped via a sustained effect on the reduction of FBG in diabetic rats.
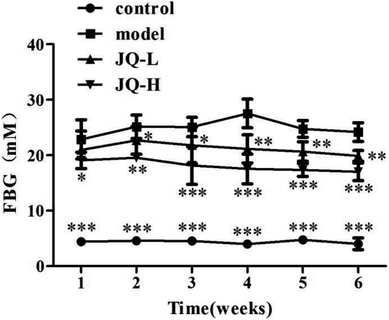 |
| Fig. 5 Effect of JQ on fasting blood glucose in diabetic rats. Data were expressed as mean ± SEM (n = 10, each group). *P < 0.05, **P < 0.01, ***P < 0.001, compared with model group. Control: the rats were treated with vehicle; model: the STZ-induced diabetic rats were treated with vehicle; JQ-L: the STZ-induced diabetic rats were treated at dose of 1.01 g kg−1 JQ; JQ-H: the STZ-induced diabetic rats were treated at dose of 4.04 g kg−1 JQ. | |
Effect of JQ on lipid profile in diabetic rats
As expected, the value of lipid metabolic parameters summarized in Fig. 6 indicated that the diabetic rats exhibited higher levels of TC (4.90 ± 0.27 mM, Fig. 6A), TG (4.38 ± 0.33 mM, Fig. 6B) and LDL-C (3.54 ± 0.24 mM, Fig. 6C) when compared with normal control group (1.77 ± 0.14 mM, 0.92 ± 0.15 mM and 1.55 ± 0.35 mM, respectively). Meanwhile, the level of HDL-C (0.59 ± 0.17 mM, Fig. 6D) was significantly reduced in diabetic rats. After six weeks treatment of JQ (1.01 g kg−1, 4.04 g kg−1), the levels of TC, TG and LDL-C were significantly decreased as compared with model group. However, there was no significant difference in HDL-C level between the low dose (1.01 g kg−1) of JQ group and model group, while the decreased level of HDL-C was significantly reversed in high-dose JQ treated group (4.04 g kg−1). These results denoted that JQ could effectively ameliorate the lipid metabolic parameters and improve blood–lipid profiles.
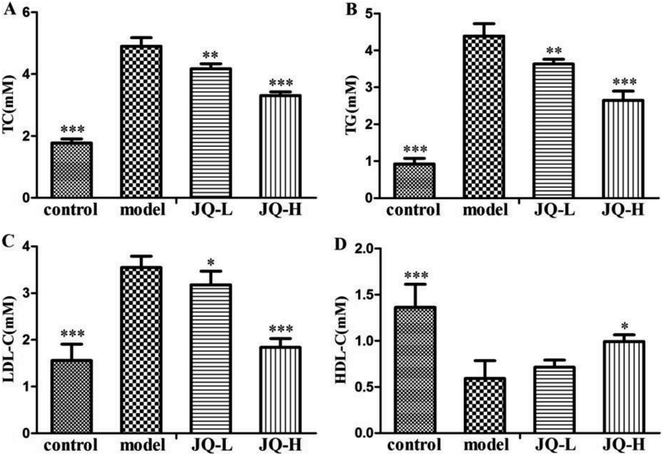 |
| Fig. 6 Effect of JQ on lipid profiles in STZ-induced diabetic rats. (A) Blood total cholesterol level; (B) blood triglyceride level; (C) blood low-density lipoprotein cholesterol level; (D) blood high-density lipoprotein cholesterol level in different rats groups. Data were expressed as mean ± SEM (n = 10, each group). *P < 0.05, **P < 0.01, ***P < 0.001, compared with model group. | |
Effect of JQ on renal functional parameters in diabetic rats
The renal functional parameters of serum and urine used as indices were summarized in Fig. 7. The levels of kidney weight to body weight ratio (Fig. 7A), 24 hour urinary protein (Fig. 7B), BUN (Fig. 7C) and SCr (Fig. 7D) were significantly increased in diabetic rats, while creatinine clearance (Fig. 7E) was found to be reduced markedly as compared with those in normal control group. During six weeks treatment period, JQ (1.01 g kg−1, 4.04 g kg−1) showed a tendency to significantly decreased the levels of 24 hour urinary protein, BUN and SCr. Creatinine clearance rate was 3.45 ± 0.14 mL min−1 in normal control group rats and was significantly decreased in diabetic rats (1.56 ± 0.19 mL min−1). However, this phenomenon was markedly reversed in JQ-treated (1.01 g kg−1 and 4.04 g kg−1) groups. The data showed that JQ could improve renal functional parameters effectively. What's more, kidney weight to body weight ratio was significantly increased as compared with normal control group. Treatment with JQ tend to significantly decrease in the kidney weight to body weight ratio (P < 0.05 and P < 0.01, respectively), which indicated that JQ could ameliorate kidney hypertrophy of diabetic rats.
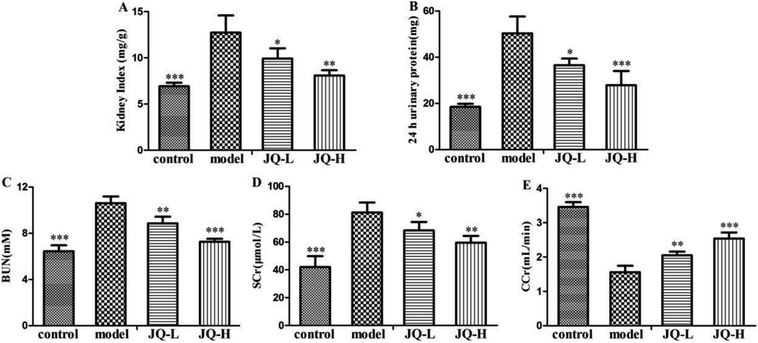 |
| Fig. 7 Effect of JQ on renal functional parameters in STZ-induced diabetic rats. (A) Kidney weight to body weight ratio (kidney index); (B) 24 hour urinary protein; (C) blood urea nitrogen (BUN); (D) serum creatinine (SCr); (E) creatinine clearance (CCr) in different groups. Data were expressed as mean ± SEM (n = 10, each group). *P < 0.05, **P < 0.01, ***P < 0.001, compared with model group. | |
JQ prevents kidney damage in diabetic rats
In the present study, the histological morphology changes of kidney were evaluated by the HE staining, periodic acid-Schif (PAS) reagent and Masson staining, respectively (Fig. 8). HE staining has been observed that the STZ-induced diabetic rats exhibited glomerular capillary basement membrane thickening and mesangial area broadening. In PAS staining, increased glomerulus, thickening of the glomerular basement membrane and accumulation of glycogen (dyed deeply prunosus) were found in STZ-induced diabetic rats. Masson's trichrome staining revealed a significant deposition of collagen fiber (dyed blue) in model group compared with control group. After six weeks intervention of JQ, all renal histological changes induced by STZ were obviously reversed in treated groups.
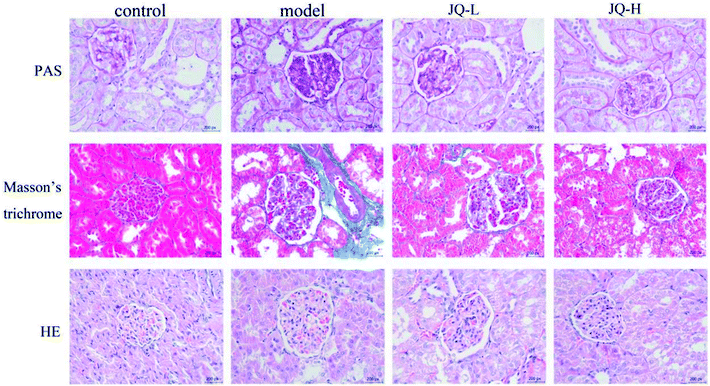 |
| Fig. 8 Representative histological changes in renal sections of periodic acid-Schiff staining, Masson's trichrome staining and Hematoxylin and eosin staining are shown (original magnification, ×400). | |
JQ ameliorates oxidative stress in the kidney of diabetic rats
It has been reported that some fractions of JQ exhibited anti-oxidative activity, it seems possible that JQ could ameliorate oxidative stress in STZ-induced diabetic rats.22,23 To assess this, we evaluated the biomarkers of oxidative stress such as the levels of SOD, MDA, CAT and GSH-Px in renal tissue after six weeks treatment of JQ. As shown in Fig. 9, the diabetic rats displayed significantly decreased the activities of SOD (8.94 ± 0.89 vs. 15.17 ± 1.21, Fig. 9A) and CAT (22.34 ± 1.18 vs. 36.54 ± 3.81, Fig. 9C), which was accompanied by a significant increase in the level of MDA (7.25 ± 0.99 vs. 4.21 ± 0.25, Fig. 9B) in renal tissue, when compared with those in normal control group. However, this phenomenon was gradually recovered after six weeks treatment, the level of renal MDA (1.01 g kg−1, P < 0.01; 4.04 g kg−1, P < 0.001) significantly decreased and renal SOD (1.01 g kg−1, P < 0.05; 4.04 g kg−1, P < 0.001) and CAT (1.01 g kg−1, P < 0.05; 4.04 g kg−1, P < 0.01) activities markedly elevated in all dose levels of JQ treated groups when compared with those in model group.
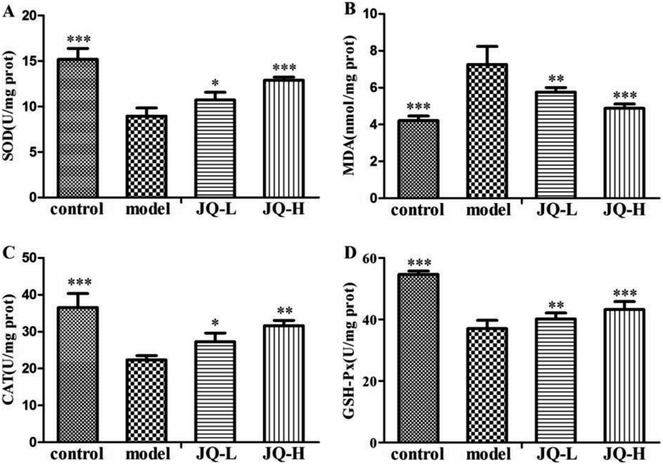 |
| Fig. 9 Effect of JQ on activity of antioxidant enzymes in STZ-induced diabetic rats. (A) Effect of JQ on SOD activity; (B) effect of JQ on MDA activity; (C) effect of JQ on CAT activity; (D) effect of JQ on GSH-Px activity in different rats groups. Data were expressed as mean ± SEM (n = 10, each group). *P < 0.05, **P < 0.01, ***P < 0.001, compared with model group. | |
In diabetic rats, the level of GSH-Px (Fig. 9D) in renal tissue decreased about 32.24% when compared with the value acquired from normal control group. However, JQ-treated diabetic rats prevented the decrease in renal GSH-Px level. The values in two JQ-treated groups were significantly elevated when compared with diabetic rats. And the high dose (4.04 g kg−1) of JQ increased renal GSH-Px level more than in lower dose (1.01 g kg−1) of JQ.
Effect of JQ on renal apoptosis in diabetic rats
In this study, we further determined the expression of apoptosis related protein, such as Caspase-3, Cyt-c, pro-apoptotic protein Bax and anti-apoptotic protein Bcl-2 in order to explore the molecular mechanism of hyperglycemia-induced apoptosis in the renal tissue of all groups (Fig. 10A). As shown by immunochemistry of renal section, the untreated diabetic rats showed a significant increase in the expression of the pro-apoptotic protein Bax when compared with the normal control group (Fig. 10B). On the other hand, the positive areas of anti-apoptotic protein Bcl-2 decreased markedly in the renal of model group (Fig. 10C). After six weeks intervention of JQ, all JQ-treated groups showed a significant decrease in the expression of Bax while increased the expression of Bcl-2 in the renal of diabetic rats.
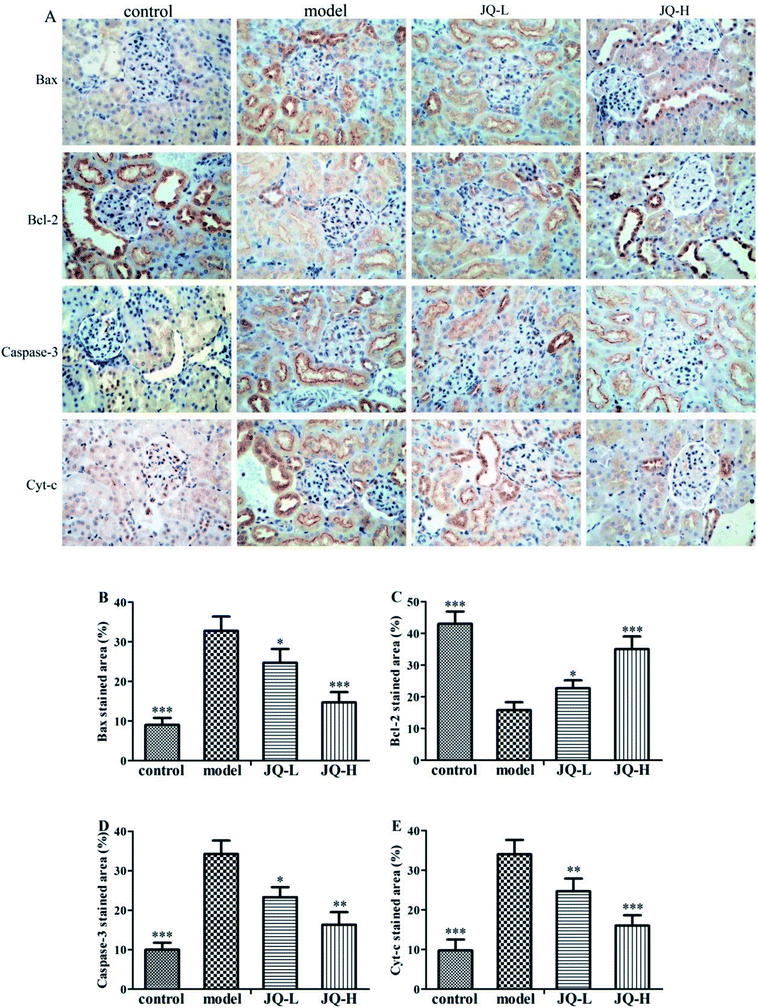 |
| Fig. 10 JQ downregulated the expression of Bax, Caspase-3 and Cyt-c and upregulated the expression of Bcl-2 in the renal tissue of diabetic rats. (A) Photomicrographs of Bax, Bcl-2, Caspase-3 and Cyt-c immune-stained renal sections of different groups (original magnification, ×400). Image analysis of (B) Bax, (C) Bcl-2, (D) Caspase-3 and (E) Cyt-c immune-staining represented as positive areas (%). Data were expressed as mean ± SEM (n = 10, each group). *P < 0.05, **P < 0.01, ***P < 0.001, compared with model group. | |
The positive areas of Caspase-3 (Fig. 10D) and Cyt-c (Fig. 10E) showed a significant increase in the model group as compared with the normal control group. Interestingly, after six weeks treatment with JQ, the increased expression of apoptosis related protein was markedly reduced compared with the model group in both two JQ-treated groups.
Discussion
To evaluate the therapeutic effect of JQ on kidney injury related diabetes mellitus, it is critical to establish a suitable animal model that similar to the process of diabetic nephropathy in human. As is known to all, STZ is widely used in studies to investigate diabetes mellitus and its complications because of it can targetedly destroy beta cells, lead to diabetic renal damage, hyperglycemia and mimic the pathology of diabetes mellitus.24,25 In this study, insulin resistance was induced via high-fat and high-sugar diet feeding, followed by low dose STZ injection to induce diabetic rat model. Higher level of blood glucose in diabetic rats successfully leads to hyperglycemia, hyperlipidemia, oxidative stress, apoptosis and kidney damage. The present results showed that JQ could significantly decrease FBG, improve blood–lipid profiles, ameliorate oxidative stress, regulate the expression of apoptosis related protein and alleviate the renal lesions. Whereupon, we have reason to demonstrate that JQ exhibit renoprotective effect in STZ-induced T2DM, and its underlying mechanism might be partially associated with anti-oxidative stress and anti-apoptosis activities.
The management of FBG is the critical approach to control T2DM and its complication. In this study, STZ-induced diabetic rats showed a decrease in body weight gain and hyperglycemia as previous study.26 Diabetic rats treated with JQ implied that it could significantly improve body weight and decrease the level of FBG. Accordingly, the previous studies have indicated the beneficial effect of JQ on FBG level in type 2 diabetic KKAy mice.27 In addition, JQ could protect insulin secretion, reduce insulin resistance and improve insulin sensitivity.28 These protective activities may explain the FBG reducing effect of JQ.
Hyperlipidemia is a common symptom of diabetic mellitus.29 It is also considered as a risk factor for diabetes mellitus because of it may worsen kidney function through the activation of various signal pathways.30 Diabetic rats that were fed with high-fat and high-sugar diet were characterized with dyslipidemia, such as elevated the levels of TC, TG, LDL-C and decreased the level of HDL-C. These changes may due to the increased mobilization of free fatty acids from peripheral deposits to central blood circulation. What's more, increased cholesterol is associated with diabetic nephropathy.31 In the present study, the levels of TC, TG and LDL-C were significantly increased, while the level of HDL-C was decreased in model group when compared with the control group. Administration of the JQ to the STZ-induced diabetic rats markedly decreased the levels of TC, TG, LDL-C, and elevated the level of HDL-C. These results implied that JQ might ameliorate diabetic symptoms via adjusting lipid metabolism and alleviating the dyslipidemia.
BUN, creatinine, 24 hour urinary protein and kidney weight to body weight ratio are usually considered as renal functional markers.32–34 The STZ-induced diabetic rat is featured with kidney hypertrophy, higher levels of 24 hour urinary protein, SCr and BUN. Additionally, creatinine clearance is another important sign of kidney function to assess kidney damage because of its positive interrelation with the glomerular filtration rate.35 The model group exhibited decreased level of creatinine clearance. What's more, increase in the kidney weight to body weight ratio was observed in STZ-induced diabetic rats, and the previous study has been indicated that an increase in the rate of protein synthesis and reduce in the degradation of renal extracellular components could lead to kidney hypertrophy.36 In this study, treatment with JQ could increase the creatinine clearance rate in diabetic rats. Meanwhile, it decreased the levels of BUN, SCr, 24 hour urinary protein and the kidney weight to body weight ratio. These results suggested that JQ ameliorated renal dysfunctions biomarkers and reversed kidney hypertrophy in STZ-induced diabetic rats.
The pathogenesis of diabetic mellitus is complicated, involving various factors and signal pathways. Kidney is one of the most sensitive organs to oxidative stress. Oxidative stress, induced by the excessive generation of ROS, reflects the disturbance in the balance between ROS production and antioxidant defense system.37 GSH-Px is an enzyme with selenium, it plays a primary part in protecting cells against oxidative damage. GSH-Px often works together with glutathione to reduce H2O2, organic and lipid hydroperoxides.38 The SOD and CAT are two major antioxidant enzymes that remove free radicals.39 MDA, a marker of oxidative stress, is a product of lipid peroxidation. In the present study, increased lipid peroxidation, reduced activities of these antioxidant enzymes and GSH-Px in kidney tissue were observed in model group. All these changes of renal tissue in STZ-induced diabetic rats demonstrated oxidative stress. Treatment with JQ reduced lipid peroxidation and boosted the activities of GSH-Px, SOD and CAT in the renal tissue of diabetic rats. The results of our study were in line with a previous study that chlorogenic acid suppressed hyperglycemia caused oxidative damages.40 These results indicated that JQ exhibited renoprotective effect by preventing the depletion of antioxidants, reducing lipid peroxidation and attenuating oxidative stress.
It has been reported that oxidative stress, mediated by ROS, is recognized as a strong mediator of apoptosis.41 Oxidative stress might lead to the imbalance between pro-apoptotic protein Bax and anti-apoptotic protein Bcl-2, caused an excessive generation of pro-apoptotic protein, which is sensitive to apoptosis. The ratio of pro-apoptotic protein Bax to anti-apoptotic protein Bcl-2 is considered as an important sign to determine cell survival versus cell death.42 And the ratio of Bax and Bcl-2 is changed in the condition of hyperglycemia in a way that is propitious to apoptosis. The increased ratio of Bax/Bcl-2 may damage the mitochondrial membrane integrity, which can lead to the release of Cyt-c from mitochondria. The increased release of Cyt-c can cause the activation of Caspase-3, and active Caspase-3 is involved in a variety of forms of apoptotic pathways.43 Oxidative stress caused apoptosis was assessed by using Bax, Bcl-2, active Caspase-3 and Cyt-c. In the present study, decreased Bcl-2 expression, and increased expression of Bax, Cyt-c, and active Caspase-3 were found in the kidney of STZ-induced diabetic rats. Interestingly, treatment with JQ significantly increased the expression of Bcl-2, decreased the expression of Bax and Cyt-c and inhibited the activation of Caspase-3. Therefore, the renoprotective effect of JQ was confirmed by ameliorating the expression of apoptosis related protein.
Conclusion
In conclusion, the present study has demonstrated that JQ exhibited renoprotective effect in HFD and STZ-induced diabetic rats. Based on these data, we hypothesized that the underlying mechanism of renoprotective effect of JQ was inhibiting the hyperglycemia-induced oxidative stress and regulating the expression of apoptosis related protein (summarized mechanistic pathways are depicted in Fig. 11). These results provide a rationale for future clinical use of JQ in protecting renal functions related T2DM. What's more, further studies are necessary to evaluate the exact underlying mechanism of JQ at the cellular and molecular level.
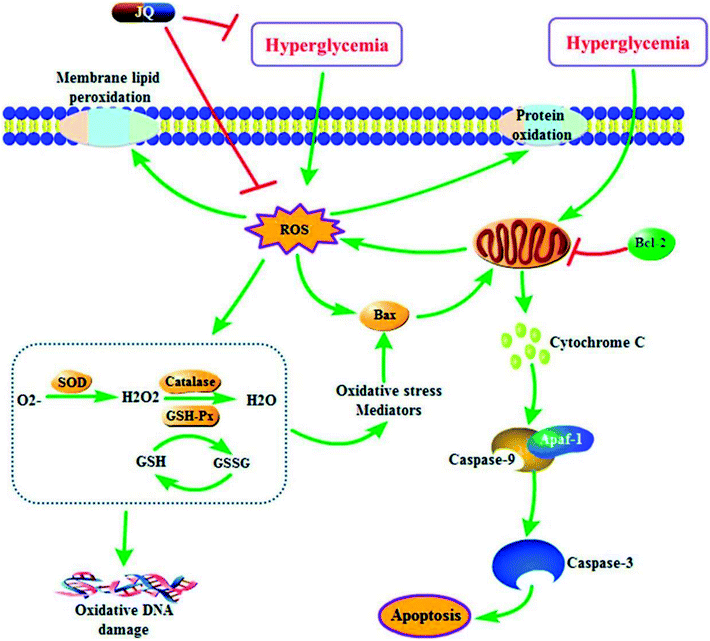 |
| Fig. 11 A proposed schematic diagram for the renoprotective mechanism of JQ on STZ-induced type 2 diabetic rats. ROS, reactive oxygen species; Bcl-2, B cell lymphoma 2; Bax, Bcl-2 associate X protein; SOD, superoxide dismutase; GSH, glutathione; GSH-Px, glutathione peroxidase; GSSG, oxidized glutathione. | |
Conflicts of interest
The authors declare they have no conflicts of interests.
Abbreviation
BUN | Blood urea nitrogen |
CAT | Catalase |
CCr | Creatinine clearance rate |
CMC-Na | Sodium carboxymethylcellulose |
Cyt-c | Cytochrome c |
FBG | Fasting blood glucose |
GSH-Px | Glutathione peroxidase |
HDL-C | High-density lipoprotein cholesterol |
HFD | High-fat and high-sugar diet |
JQ | JinQi-JiangTang tablet |
LDL-C | Low-density lipoprotein cholesterol |
MDA | Malondialdehyde |
PAS | Periodic acid-Schiff |
ROS | Reactive oxygen species |
SCr | Serum creatinine |
SOD | Superoxide dismutase |
STZ | Streptozotocin |
TC | Total cholesterol |
T2DM | Type 2 diabetes mellitus |
TG | Triglyceride |
UPLC | Ultra performance liquid chromatography |
Acknowledgements
This research was supported by grants from TCM, standardization and quality promotion of 4 of TCM pieces (No. ZYBZH-Y-GS-11), the Science and Technology Ministry program of China (No. 2014FY111100); National Natural Science Foundation of China Youth Fund Project (No. 81503467).
References
- R. C. W. Ma, Diabetologia, 2018, 61, 1249–1260 CrossRef.
- K. R. Tuttle, G. L. Bakris, R. W. Bilous, J. L. Chiang, I. H. de Boer, J. Goldstein-Fuchs, I. B. Hirsch, K. Kalantar-Zadeh, A. S. Narva, S. D. Navaneethan, J. J. Neumiller, U. D. Patel, R. E. Ratner, A. T. Whaley-Connell and M. E. Molitch, Am. J. Kidney Dis., 2014, 64, 510–533 CrossRef.
- G. Wolf, J. Am. Soc. Nephrol., 2003, 14, 1396–1405 CrossRef.
- J. A. Jefferson, S. J. Shankland and R. H. Pichler, Kidney Int., 2008, 74, 22–36 CrossRef CAS.
- M. Guha, Z. G. Xu, D. Tung, L. Lanting and R. Natarajan, FASEB J., 2007, 21, 3355–3368 CrossRef CAS.
- S. Yamagishi, K. Fukami, S. Ueda and S. Okuda, Curr. Drug Targets, 2007, 8, 952–959 CrossRef CAS.
- L. Gnudi, R. J. M. Coward and D. A. Long, Trends Endocrinol. Metab., 2016, 27, 820–830 CrossRef CAS.
- Y. Park, H. Kim, L. Park, D. Min, J. Park, S. Choi and M. H. Park, PLoS One, 2015, 10, e0130815 CrossRef.
- S. L. Habib, World J. Diabetes, 2013, 4, 27–30 CrossRef.
- B. Dai, Q. Wu, C. Zeng, J. Zhang, L. Cao, Z. Xiao and M. Yang, J. Ethnopharmacol., 2016, 192, 382–389 CrossRef PubMed.
- H. Cao, M. Ren, L. Guo, H. Shang, J. Zhang, Y. Song, H. Wang, B. Wang, X. Li, J. Hu, X. Wang, D. Wang, J. Chen, S. Li and L. Chen, Trials, 2010, 11, 27–34 CrossRef.
- Z. Zhou, J. Tradit. Chin. Med., 2002, 18, 3–4 Search PubMed.
- L. Q. Tang, W. J. Ni, M. Cai, H. H. Ding, S. Liu and S. T. Zhang, J. Diabetes, 2016, 8, 693–700 CrossRef CAS.
- Y. X. Chang, A. H. Ge, X. A. Yu, X. C. Jiao, J. Li, J. He, J. Tian, W. Liu, J. T. Azietaku, B. L. Zhang and X. M. Gao, J. Pharm. Biomed. Anal., 2016, 117, 1–10 CrossRef CAS.
- S. Ogechi Ekeuku, P. Nwabueze Okechukwu, G. Akyirem Akowoah, T. Swee Sen, S. Namatama Siyumbwa and G. Ruth Anisah Froemming, Curr. Bioact. Compd., 2015, 11, 256–263 CrossRef.
- T. Yokozawa, T. Nakagawa, K. Wakaki and F. Koizumi, Exp. Toxicol. Pathol., 2001, 53, 359–363 CrossRef CAS PubMed.
- G. Mahendran and V. Narmatha Bai, Acta Physiol. Plant., 2013, 36, 589–603 CrossRef.
- Y. Boyang and G. Qing, J. Tradit. Chin. Med., 2015, 24, 809–815 Search PubMed.
- L. Liu, Z. B. Wang, Y. Song, J. Yang, L. J. Wu, B. Y. Yang, Q. H. Wang, L. Q. Wang, R. X. Wang and C. J. Yang, Molecules, 2016, 21, 913–927 CrossRef.
- Q. Liu, S. Qiu, H. Yu, Y. Ke, Y. Jin and X. Liang, Analyst, 2011, 136, 4357–4365 RSC.
- D. Saxena, R. Pal, A. K. Dwivedi and S. Singh, J. Sci. Ind. Res., 2004, 63, 181–186 CAS.
- M. R. Shi, C. X. Zhao, X. H. Ge, H. Y. Yang, L. Q. Ge, G. J. Zhu and W. W. Xu, Neuropsychiatry, 2018, 8, 24–33 CrossRef.
- A. M. Mahmoud, M. M. Abdel-Rahman, N. A. Bastawy and H. M. Eissa, J. Appl. Pharm. Sci., 2017, 7, 1–10 CAS.
- T. Szkudelski, Physiol. Res., 2001, 50, 537–546 CAS.
- J. M. Forbes and M. E. Cooper, Physiol. Rev., 2013, 93, 137–188 CrossRef CAS.
- H. Zhou, Z. Qu, J. Zhang, Y. Liu, H. Yang, H. Chen, Y. Li, C. Liu and W. Gao, RSC Adv., 2016, 6, 109132–109142 RSC.
- Q. Liu, S. Liu, L. Gao, S. Sun, Y. Huan, C. Li, Y. Wang, N. Guo and Z. Shen, Acta Pharm. Sin. B, 2017, 7, 461–469 CrossRef PubMed.
- L. H. Gao, Q. Liu, S. N. Liu, Z. Y. Chen, C. N. Li, L. Lei, S. J. Sun, L. Y. Li, J. L. Liu and Z. F. Shen, J. Ethnopharmacol., 2014, 151, 675–685 CrossRef PubMed.
- Y. Ma, Y. Wang, Q. Huang, Q. Ren, S. Chen, A. Zhang, L. Zhao, Q. Zhen and Y. Peng, J. Diabetes Res., 2014, 2014, 493039 Search PubMed.
- R. F. Rosario and S. Prabhakar, Curr. Diabetes Rep., 2006, 6, 455–462 CrossRef CAS.
- K. N. Nesbitt, J. Pharm. Pract., 2016, 17, 75–79 CrossRef.
- A. M. Salem, A. S. Ragheb, M. G. A. Hegazy, M. Matboli and S. Eissa, Indian J. Clin. Biochem., 2018, 1–8 CAS.
- S. T. Ma, D. L. Liu, J. J. Deng, R. Niu and R. B. Liu, Phytother. Res., 2013, 27, 1474–1480 CAS.
- Y. Zhao, W. Huang, J. Wang, Y. Chen, W. Huang and Y. Zhu, Am. J. Transl. Res., 2018, 10, 1205–1210 Search PubMed.
- K. D. G. Edwards and H. M. Whyte, Australas. Ann. Med., 1959, 8, 218–224 CrossRef CAS.
- M. Zafar and S. N. U. H. Naqvi, Int. J. Morphol., 2010, 28, 135–142 Search PubMed.
- S. P. D. Dwivedi J, Int. J. Appl. Biol. Pharm. Technol., 2010, 4, 104–107 Search PubMed.
- S. S. Gill and N. Tuteja, Plant Physiol. Biochem., 2010, 48, 909–930 CrossRef CAS.
- K. B. Kim and B. M. Lee, Cancer Lett., 1997, 113, 205–212 CrossRef CAS.
- H. Y. Ye, Z. Y. Li, Y. Zheng, Y. Chen, Z. H. Zhou and J. Jin, Arch. Pharmacal Res., 2016, 39, 989–997 CrossRef CAS.
- S. P. A. Buttke T M, Immunol. Today, 1994, 15, 7–10 CrossRef.
- Z. N. Oltvai, C. L. Milliman and S. J. Korsmeyer, Cell, 1993, 74, 609–619 CrossRef CAS PubMed.
- S. Desagher and J. C. Martinou, Trends Cell Biol., 2000, 10, 369–377 CrossRef CAS PubMed.
|
This journal is © The Royal Society of Chemistry 2018 |
Click here to see how this site uses Cookies. View our privacy policy here.