DOI:
10.1039/C8RA07841F
(Paper)
RSC Adv., 2018,
8, 35056-35061
Substrate switchable Suzuki–Miyaura coupling for benzyl ester vs. benzyl halide†
Received
21st September 2018
, Accepted 8th October 2018
First published on 12th October 2018
Abstract
Two reaction conditions were developed to accomplish the substrate switchable Suzuki–Miyaura coupling of benzyl derivatives and arylboronic acid derivatives. Under conditions for esters, benzyl esters such as carbonates and acetates reacted with arylboronic acids to afford the corresponding diarylmethanes. However, the benzyl halides did not react under the same conditions. On the other hand, benzyl halides such as bromides and chlorides furnished diarylmethanes under conditions for halides, under which benzyl ester substrates did not react, in which water was found to play an important role. This switching system was tested using the intermolecular/intramolecular competitive reactions, during which the desired products could be synthesized by selecting the appropriate reaction conditions.
Introduction
Diarylmethanes are an important class of compounds because they are widely used as a framework for functional materials including polymers,1 dyes,2 and biologically active compounds.3 Various methods have been reported for their synthesis, including the Suzuki–Miyaura coupling reaction, which has become our focus.4–6 In a typical Suzuki–Miyaura coupling, benzyl esters (carbonates or acetates) are reacted with arylboronic acids in the presence of a Pd catalyst under air atmosphere to efficiently afford the corresponding diarylmethanes.5 In our protocol, the Pd catalyst is prepared from inexpensive and commercially available PdCl2 and bidentate bis(phosphine) ligands. The ability to use an accessible catalyst improved the practicality of the reaction. Moreover, the reactivity of the benzyl esters was found to be between that of chloro- and bromobenzenes. This property facilitated the selective synthesis of benzyl(biphenyl)s by successive Suzuki–Miyaura coupling reactions (Scheme 1). These results prompted us to investigate a more challenging project; controlling chemoselectivity for benzyl esters and benzyl halides. In this study, we managed to successfully switch the substrate favored for coupling by changing the reaction conditions.
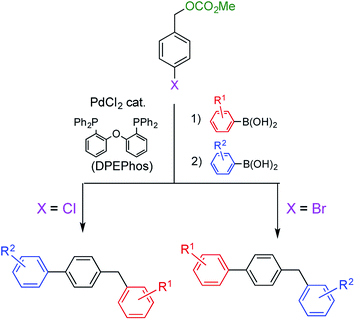 |
| Scheme 1 Chemoselective synthesis of benzyl(biphenyl)s. | |
Results and discussion
When benzyl carbonate 1A was reacted with phenylboronic acid (5a) (1.25 equiv.) in the presence of the Pd catalyst derived from PdCl2 (5 mol%) and DPEPhos (5.5 mol%) in ethanol, the coupling reaction proceeded to afford diarylmethane 6Aa4f in a 76% yield after heating at 80 °C for 1 d (Table 1, entry 1). These conditions are referred to as conditions for esters, in which lack of any reagent did not undergo the reaction at all. Although benzyl acetate 2A reacted similarly, its reactivity was lower than that of carbonate 1A (entry 2). On the other hand, benzyl halides 3A and 4A did not react under the same conditions (entries 3 and 4). After surveying several reaction condition options, the choice of ligand, base, and solvent was found to be crucial to switching the reactivity (Table S1 in the ESI†). A combination of triphenylphosphine and Na2CO3 facilitated the reaction of benzyl chloride 4A (entry 5). A complete switch was made to afford 6Aa efficiently while 1A did not react (entries 6–10; successful reactions were performed in a mixed THF/water (v/v = 1/1) solvent at 40 °C, referred to conditions for halides). Benzyl bromide 3A showed similar behavior to 4A to undergo the coupling reaction efficiently only under conditions for halides (entry 8). Usually, THF and water is miscible; however, a mixed solvent (v/v = 1/1) was separated into two layers in this reaction. On the other hand, the yields of 6Aa were considerably lower in reactions performed using a homogeneous mixed solvent of THF and water (v/v = 4/1), THF and ethanol (v/v = 1/1) or THF alone (entries 11–16). In addition, triethylamine was not an effective base for this reaction (entry 17). To the contrary, the reaction proceeded more efficiently when a mixed solvent of toluene and water (v/v = 1/1) was employed (entries 18 and 19). Based on these results, the presence of water was found to be crucial for this reaction. Although there is a report that aqueous conditions are effective for the reaction using DPEPhos, exact role of water was not mentioned.7
Table 1 Assessment of switchable reaction conditions depending on the substrate

|
Entry |
Substrate |
Ligand |
Base |
Solv. |
Temp./°C |
Conversion of substrate/% |
Yielda/% |
X |
|
Determined by 1H NMR. v/v = 1/1 (heterogeneous). v/v = 4/1 (homogeneous). |
1 |
OCOOMe |
1A |
DPEPhos |
NaHCO3 |
EtOH |
80 |
89 |
76 |
2 |
OAc |
2A |
DPEPhos |
NaHCO3 |
EtOH |
80 |
65 |
59 |
3 |
Br |
3A |
DPEPhos |
NaHCO3 |
EtOH |
80 |
6 |
0 |
4 |
Cl |
4A |
DPEPhos |
NaHCO3 |
EtOH |
80 |
4 |
0 |
5 |
Cl |
4A |
PPh3 |
Na2CO3 |
EtOH |
80 |
13 |
10 |
6 |
OCOOMe |
1A |
PPh3 |
Na2CO3 |
THF/H2Ob |
80 |
100 |
23 |
7 |
Cl |
4A |
PPh3 |
Na2CO3 |
THF/H2Ob |
80 |
100 |
92 |
8 |
Br |
3A |
PPh3 |
Na2CO3 |
THF/H2Ob |
80 |
100 |
89 |
9 |
OCOOMe |
1A |
PPh3 |
Na2CO3 |
THF/H2Ob |
40 |
4 |
0 |
10 |
Cl |
4A |
PPh3 |
Na2CO3 |
THF/H2Ob |
40 |
85 |
81 |
11 |
OCOOMe |
1A |
PPh3 |
Na2CO3 |
THF/H2Oc |
40 |
0 |
0 |
12 |
Cl |
4A |
PPh3 |
Na2CO3 |
THF/H2Oc |
40 |
78 |
74 |
13 |
OCOOMe |
1A |
PPh3 |
Na2CO3 |
THF/EtOHb |
40 |
8 |
4 |
14 |
Cl |
4A |
PPh3 |
Na2CO3 |
THF/EtOHb |
40 |
16 |
12 |
15 |
OCOOMe |
1A |
PPh3 |
Na2CO3 |
THF |
40 |
0 |
0 |
16 |
Cl |
4A |
PPh3 |
Na2CO3 |
THF |
40 |
9 |
4 |
17 |
Cl |
4A |
PPh3 |
Et3N |
THF |
40 |
30 |
14 |
18 |
OCOOMe |
1A |
PPh3 |
Na2CO3 |
PhMe/H2Ob |
40 |
0 |
0 |
19 |
Cl |
4A |
PPh3 |
Na2CO3 |
PhMe/H2Ob |
40 |
67 |
62 |
In order to obtain insights for the role of water, density functional theory (DFT) calculation using B3LYP was performed for two plausible intermediate complexes [PdBnCl(PPh3)2] (+H2O) and [PdBnCl(PPh3)(OH2)] (+PPh3) (Table 2, Fig. 1 and 2). By comparing the ΔGtot values of these complexes, the latter is thermodynamically favored by −ΔGtot = 0.032521 a.u. (20.4 kcal mol−1).
Table 2 Thermodynamic parameters of [PdBnCl(PPh3)L]
Compound |
E0a/a.u. |
Gcorra/a.u. |
Gtota/a.u. |
E0: total electronic energy, Gcorr: thermal correction to Gibbs free energy, Gtot: sum of electronic and thermal free energies. |
[PdBnCl(PPh3)(OH2)] |
−1970.727043 |
+0.356655 |
−1970.370388 |
PPh3 |
−1036.322130 |
+0.227447 |
−1036.094683 |
[Pd(Bz)Cl(PPh3)(OH2)] (+PPh3) |
|
|
−3006.465071 |
[PdBnCl(PPh3)2] |
−2930.603622 |
+0.587757 |
−2930.015865 |
H2O |
−76.419737 |
+0.003052 |
−76.416685 |
[PdBnCl(PPh3)2] (+H2O) |
|
|
−3006.432550 |
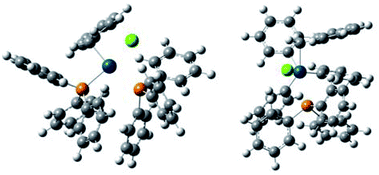 |
| Fig. 1 Optimized geometry of [PdBnCl(PPh3)2]: top view (left) and side view (right). | |
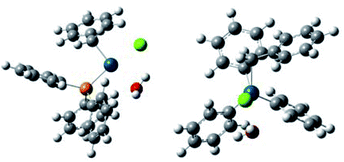 |
| Fig. 2 Optimized geometry of [PdBnCl(PPh3)(OH2)]: top view (left) and side view (right). | |
It is considered that different reactivity between benzyl esters and benzyl halides are brought about different mode during the oxidative addition steps in the catalytic cycle. When benzyl carbonate 1A undergoes oxidative addition to Pd0 species, π-complex 7 is formed accompanied by decarboxylation (Scheme 2, eqn (1)). Benzyl acetate 2A also affords similar complex intermediately. On the other hand, in cases of benzyl chloride 4A, σ-complex 8 is formed, and benzyl bromide 3A also affords similar complex. One of the phosphine ligands in 8 is easily exchanged with water leading to complex 9, which undergoes the subsequent coupling reaction efficiently (eqn (2)).
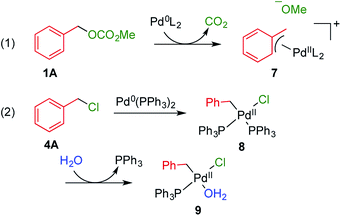 |
| Scheme 2 Plausible intermediate complexes in the coupling reactions using benzyl carbonate 1A and benzyl chloride 4A. | |
Other benzyl derivatives 1–4 were coupled under the conditions optimized for 4-methylbenzyl carbonate 1A (conditions for esters) or 4-methylbenzyl chloride 4A (conditions for halides), and each reaction proceeded in high conversion yield (Table 3). Under conditions for esters, benzyl carbonates 1 and benzyl acetates 2 exhibited similar reactivity to afford the corresponding diarylmethanes 6 in comparable yields; however, benzyl halides 3 and 4 did not react except for highly reactive methoxy derivatives 3B and 4B (Table S2 in ESI†). To the contrary, only benzyl halides 3 and 4 coupled under conditions for halides, and no reaction was observed when benzyl esters 1 and 2 were employed (Table S3 in ESI†). In cases of the less reactive, electron-deficient benzyl chlorides 4C–F, yields of diarylmethanes 6Ca–Fa were higher than those obtained in the reactions using 1C–F under conditions for esters.
Table 3 Chemoselective coupling reactions using benzyl carbonates 1 and benzyl chlorides 4a

|
Reactions of esters 1b |
Entry |
Benzyl carbonate |
Conversion of 1/% |
Product |
Ar |
|
|
Yield/% |
Each reaction was conducted using boronic acid (1.25 equiv.), PdCl2 (5 mol%). Conditions for esters: DPEPhos (5.5 mol%), NaHCO3 (2.5 equiv.) in ethanol, at 80 °C for 1 d. Conditions for halides: Ph3P (11 mol%), Na2CO3 (2.5 equiv.) in THF/H2O (v/v = 1/1), at 40 °C for 1 d. |
1 |
4-MeOC6H4 |
1B |
100 |
6Ba4c |
92 |
2 |
4-CF3C6H4 |
1C |
70 |
6Ca4i |
58 |
3 |
4-NO2C6H4 |
1D |
49 |
6Da4f |
41 |
4 |
2-MeC6H4 |
1E |
40 |
6Ea4i |
34 |
5 |
2-Pyridyl |
1F |
81 |
6Fa8 |
60 |
6 |
2-Furyl |
1G |
68 |
6Ga4c |
51 |
7 |
2-Thienyl |
1H |
77 |
6Ha4c |
64 |
Reactions of halides 4c |
Entry |
Benzyl chloride |
Conversion of 4/% |
Product |
Ar |
|
|
Yield/% |
8 |
4-MeOC6H4 |
4B |
90 |
6Ba |
87 |
9 |
4-CF3C6H4 |
4C |
74 |
6Ca |
70 |
10 |
4-NO2C6H4 |
4D |
62 |
6Da |
54 |
11 |
2-MeC6H4 |
4E |
48 |
6Ea |
45 |
12 |
2-Pyridyl |
4F |
73 |
6Fa |
67 |
13 |
2-Furyl |
4G |
64 |
6Ga |
52 |
14 |
2-Thienyl |
4H |
69 |
6Ha |
64 |
When other boronic acids, 5b–d, were reacted under conditions for esters, carbonate 1A and acetate 2A afforded diarylmethanes 6Ab–Ad while halides 3A and 4A did not afford 6 (Tables 4 and S4 in ESI†). The coupling reactions were not influenced by the electronic properties of the substituents on the boronic acids 5. On the other hand, 1A and 2A were unreactive under conditions for halides. Instead, bromide 3A and chloride 4A furnished 6Ab–Ad in higher yields (Tables 4 and S5 in ESI†). The substrates (benzyl esters or benzyl halides) were successfully switched by changing reaction conditions. Furthermore, the switching mode was not influenced by substituents on the benzene ring of either coupling partner.
Table 4 Switchable coupling reaction using other boronic acida
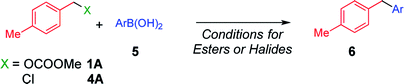
|
Reactions of ester 1Ab |
Entry |
Boronic acid |
Conversion of 1/% |
Product |
Ar |
|
|
Yield/% |
Each reaction was conducted using boronic acid (1.25 equiv.), PdCl2 (5 mol%). Conditions for esters: DPEPhos (5.5 mol%), NaHCO3 (2.5 equiv.) in ethanol, at 80 °C for 1 d. Conditions for halides: Ph3P (11 mol%), Na2CO3 (2.5 equiv.) in THF/H2O (v/v = 1/1), at 40 °C for 1 d. |
1 |
4-MeOC6H4 |
5b |
87 |
6Ab6d |
79 |
2 |
4-CF3C6H4 |
5c |
86 |
6Ac9 |
75 |
3 |
2-MeC6H4 |
5d |
85 |
6Ad10 |
70 |
Reactions of halide 4Ac |
Entry |
Boronic acid |
Conversion of 4/% |
Product |
Ar |
|
|
Yield/% |
4 |
4-MeOC6H4 |
5b |
70 |
6Ab |
62 |
5 |
4-CF3C6H4 |
5c |
67 |
6Ac |
60 |
6 |
2-MeC6H4 |
5d |
66 |
6Ad |
52 |
Next, we conducted directly competitive reactions of the two substrates (Scheme 3). A mixture of equimolar of benzyl carbonate 1I and benzyl chloride 4A was reacted with phenylboronic acid 5a. When the reaction was conducted under conditions for esters, only carbonate 1I was consumed to form 6Ia;9 4A remained unreacted. To the contrary, only chloride 4A was consumed under conditions for halides. Hence, this switching system was useful even when the reaction mixture contains both benzyl esters and halides.
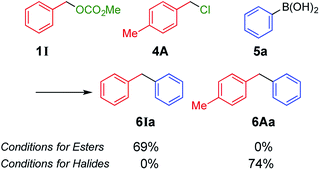 |
| Scheme 3 Competitive reactions using 1I and 4A. | |
Compound 10, which possesses both a benzyl ester and benzyl halide moiety was subjected to each of the two reaction conditions (Scheme 4). Compound 1111 was selectively obtained under conditions for esters. On the other hand, compound 13 was the product when the reaction was carried out under conditions for halides. Product 11 was further converted to benzyl cyanide 1212 upon treatment with sodium cyanide, and product 13 was transformed to benzyl carbamate 14 by nucleophilic substitution with methylamine.13 As a result, two different classes of compound were synthesized from common starting material 10.
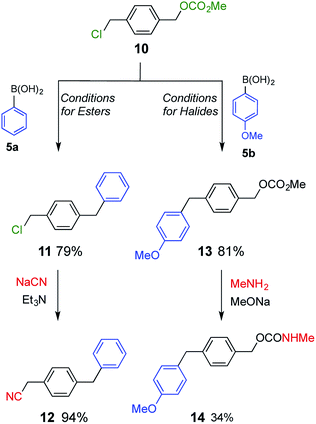 |
| Scheme 4 Intramolecular chemoselective coupling reactions of differently substituted compound 10. | |
Conclusions
A chemoselective Suzuki–Miyaura coupling reaction was achieved by switching reaction conditions. Benzyl carbonates 1 and benzyl acetates 2 underwent the coupling reaction with arylboronic acids 5 to afford the corresponding diarylmethanes 6 using a Pd catalyst derived from PdCl2 and DPEPhos in ethanol in the presence of NaHCO3 (conditions for esters). Benzyl halides 3 and 4 did not react under the same conditions. On the other hand, when benzyl halides 3 and 4 were reacted using a Pd catalyst derived from PdCl2 and Ph3P in THF/water in the presence of Na2CO3 (conditions for halides), the coupling reaction efficiently proceeded to afford diarylmethanes 6 while benzyl esters 1 and 2 did not react. This substrate selectivity depends on the different mode of the oxidative addition, and water displaces one of the phosphine ligands. These switchable conditions facilitated the chemoselectivity even when benzyl esters and benzyl halides coexist in a reaction mixture or molecule. The present system will be a useful tool in elaborate syntheses.
Experimental
General
All the reagents and solvents were commercially available and used as received. The 1H NMR spectra were measured on a JEOL 400 spectrometer at 400 MHz with TMS as an internal standard. The 13C NMR spectra were measured on a JEOL 400 spectrometer at 100 MHz. The IR spectra were recorded on a JASCO FT/IR-4100 spectrometer. The melting points were determined on an As-one melting-points apparatus ATM-02, and were uncorrected. High-resolution mass spectra were obtained on an AB SCEIX Triplet TOF 4600 mass spectrometer. Gas chromatography (GC) was performed with Shimadzu GC 8A. Flash column chromatography was performed with Wako-gel C-200 (100–200 mesh, Wako).
Theoretical calculations for the complexes were carried out with 16W software.14 Optimizations of the ground-state geometries of the complexes were performed by using the this pro density functional theory (DFT).15 The LanL2DZ16 and 6-31G(d,p)17 basis sets were used to treat the palladium and all other atoms, respectively. Optimized geometries of the complexes were plotted using GaussView 6.0.18
All diarylmethanes 6, 11 and 12 are commercially available. Hence, the structures of these products were confirmed by comparison of spectral data with those of authentic samples.
General procedure of the Suzuki–Miyaura coupling reaction under conditions for esters
To a solution of PdCl2 (1.8 mg, 10 μmol), DPEPhos (5.9 mg, 11 μmol), NaHCO3 (42.0 mg, 0.5 mmol), and phenylboronic acid 5a (30.5 mg, 0.25 mmol) in EtOH (1.0 mL), 4-methylbenzyl carbonate 1A (36.0 mg, 0.2 mmol) was added, and the resultant mixture was heated in a screw-capped sealed tube at 80 °C for 1 d. After treatment with flash column chromatography using hexane to afford 6Aa (26.6 mg, 0.146 mmol, yield 73%), which is confirmed to be not so different from the yield (76%) determined by 1H NMR. When other conditions and substrates were employed, the experiments were conducted in a similar way.
General procedure of the Suzuki–Miyaura coupling reaction under conditions for halides
To a solution of PdCl2 (1.8 mg, 10 μmol), PPh3 (5.8 mg, 22 μmol), Na2CO3 (53.0 mg, 0.5 mmol), and phenylboronic acid 5a (30.5 mg, 0.25 mmol) in THF and water (v/v = 1/1) (1.0 mL), 4-methylbenzyl chloride 4A (28.1 mg, 0.2 mmol) was added, and the resultant mixture was heated in a screw-capped sealed tube at 40 °C for 1 d. After treatment with flash column chromatography using hexane to afford 6Aa (28.8 mg, 0.158 mmol, yield 79%), which is confirmed to be not so different from the yield (81%) determined by 1H NMR. When other conditions and substrates were employed, the experiments were conducted in a similar way.
Intermolecular competitive reaction
Under conditions for esters. To a solution of PdCl2 (1.8 mg, 10 μmol), DPEPhos (5.9 mg, 11 μmol), NaHCO3 (42.0 mg, 0.5 mmol), and phenylboronic acid 5a (30.5 mg, 0.25 mmol) in EtOH (0.5 mL), a solution of benzyl carbonate 1I (30.0 mg, 0.2 mmol) and 4-methylbenzyl chloride 4A (28.1 mg, 0.2 mmol) in EtOH (0.5 mL) was added, and the resultant mixture was heated in a screw-capped sealed tube at 80 °C for 1 d. After treatment with flash column chromatography using hexane to afford 6Ia (23.2 mg, 0.138 mmol, yield 69%) without any detectable 6Aa.
Under conditions for halides. To a solution of PdCl2 (1.8 mg, 10 μmol), PPh3 (5.8 mg, 22 μmol), Na2CO3 (53.0 mg, 0.5 mmol), and phenylboronic acid 5a (30.5 mg, 0.25 mmol) in THF and water (v/v = 1/1) (0.5 mL), a solution of benzyl carbonate 1I (30.0 mg, 0.2 mmol) and 4-methylbenzyl chloride 4A (28.1 mg, 0.2 mmol) in THF and water (v/v = 1/1) (0.5 mL) was added, and the resultant mixture was heated in a screw-capped sealed tube at 40 °C for 1 d. After treatment with flash column chromatography using hexane to afford 6Aa (26.9 mg, 0.148 mmol, yield 74%) without any detectable 6Ia.
Chemical conversion of diphenylmethanes
4-[(4-Methoxyphenyl)methyl]phenylmethyl methyl carbonate (13). White solid; mp 176–177 °C. 1H NMR (CDCl3, 400 MHz) δ 7.31 (d, J = 8.4 Hz, 2H), 7.23 (d, J = 8.4 Hz, 2H), 7.15 (d, J = 8.4 Hz, 2H), 6.88 (d, J = 8.8 Hz, 2H), 5.08 (s, 2H), 4.21 (s, 2H), 3.79 (s, 3H), 3.76 (s, 3H); 13C NMR (CDCl3, 100 MHz) δ 159.9, 156.0, 137.2, 130.4, 129.2, 127.5, 127.2, 114.0, 113.7, 69.6, 55.3, 54.9, 40.6; IR (neat) 3473, 2958, 1747, 1613, 1515, 1442, 1377, 1247 cm−1; HRMS (ESI–TOF) m/z [M + Na]+ calcd for C17H18O4Na 309.1097; found 309.1099.
Cyanation of benzyl chloride 11. To a solution of benzyl chloride 11 (216 mg, 1.0 mmol) in DMSO (2 mL), were added sodium cyanide (147 mg, 3.0 mmol) and triethylamine (304 mg, 3.0 mmol), and the resultant mixture was heated at 80 °C for 1 d. After filtration using a Celite pad, the filtrate was extracted with ethyl acetate (10 mL × 3). The combined organic layer was washed with brine (10 mL × 1), dried over MgSO4, and concentrated under reduced pressure. The residue was treated with flash column chromatography (EtOAc/hexane = 20/80) to afford benzyl cyanide 12 (194 mg, 0.94 mmol, yield 94%).
4-[(4-Methoxyphenyl)methyl]phenylmethyl methyl carbamate (14). To a solution of carbonate 13 (286 mg, 1.0 mmol) in toluene (2 mL), were added methylamine (31.1 mg, 1.0 mmol) and sodium methoxide (54 mg, 1.0 mmol), and the resultant mixture was heated at 80 °C for 1 d. After filtration using a Celite pad, the filtrate was extracted with ethyl acetate (10 mL × 3). The combined organic layer was washed with brine (10 mL × 1), dried over MgSO4, and concentrated under reduced pressure. The residue was treated with flash column chromatography (EtOAc/hexane = 50/50) to afford carbamate 14 (96 mg, 0.34 mmol, yield 34%). White solid; mp 94–95 °C. 1H NMR (CDCl3, 400 MHz) δ 7.28 (d, J = 8.8 Hz, 2H), 7.20 (d, J = 8.4 Hz, 2H), 7.11 (d, J = 8.0 Hz, 2H), 6.85 (d, J = 8.8 Hz, 2H), 6.1–6.3 (br, 1H), 5.04 (s, 2H), 4.19 (s, 2H), 3.72 (s, 3H), 2.69 (s, 3H); 13C NMR (CDCl3, 100 MHz) δ 159.9, 155.9, 137.1, 130.4, 129.2, 127.5, 127.2, 114.3, 113.7, 68.4,54.9, 40.8, 21.2; IR (neat) 3319, 2957, 1747, 1657, 1515, 1443, 1273 cm−1; HRMS (ESI–TOF) m/z [M + Na]+ calcd for C17H19NO3Na 308.1257, found 308.1258.
Conflicts of interest
There are no conflicts to declare.
Notes and references
- W. Li, F. Liu, L. Wei and T. Zhao, J. Appl. Polym. Sci., 2007, 104, 3903 CrossRef CAS; T. Akiike and K. Kakutani, Jpn. Kokai Tokkyo Koho, JP Pat, JP 2006143765, 2006 CrossRef PubMed; S. I. Nikitenko, Y. Koltypin, D. M. Pickup, E. R. H. Van-Eck and A. Gedanken, Ultrason. Sonochem., 2003, 10, 11 CrossRef PubMed.
- B.-B. Xie, S.-H. Xia, X.-P. Chang and G. Cui, Phys. Chem. Chem. Phys., 2016, 18, 403 RSC; P. Prosposito, H. Zhang and M. Glasbeek, J. Sol-Gel Sci. Technol., 2011, 60, 347 CrossRef CAS.
- M. Nambo, D. Kurihara, T. Yamada, T. Nishiwaki-Ohkawa, N. Kadofusa, Y. Kimata, K. Kuwata, M. Umeda and M. Ueda, Plant Cell Physiol., 2016, 57, 2255 CrossRef CAS PubMed; G. Chiellini, G. Nesi, S. Sestito, S. Chiarugi, M. Runfola, S. Espinoza, M. Sabatini, L. Bellusci, A. Laurino, E. Cichero, R. R. Gainetdinov, P. Fossa, L. Raimondi, R. Zucchi and S. Rapposelli, J. Med. Chem., 2016, 59, 9825 CrossRef PubMed; T. T. M. Pham and M. Sylvestre, J. Bacteriol., 2013, 195, 3563 CrossRef PubMed; W. Eisenreich, N. S. Ladyzhynsky, D. Li, L. Schultz, Z. Wang, S. Macha and A. Barta, PCT Int. Appl., WO Pat, WO, 2010092126, 2010.
- From benzyl halides:
(a) R. B. Bedford, P. B. Brenner, E. Carter, T. W. Carvell, P. M. Cogswell, T. Gallagher, J. N. Harvey, D. M. Murphy, E. C. Neeve, J. Nunn and D. R. Pye, Chem.–Eur. J., 2014, 20, 7935 CrossRef CAS PubMed;
(b) Y.-Y. Sun, J. Yi, X. Lu, Z.-Q. Zhang, B. Xiao and Y. Fu, Chem. Commun., 2014, 50, 11060 RSC;
(c) Y.-Q. Zhang, J. Chem. Res., 2013, 37, 375 CrossRef CAS;
(d) N. Henry, C. Enguehard-Gueiffier, I. Thery and A. Gueiffier, Eur. J. Org. Chem., 2008, 4824 CrossRef CAS;
(e) M. J. Burns, I. J. S. Fairlamb, A. R. Kapdi, P. Sehnal and R. J. K. Taylor, Org. Lett., 2007, 9, 5397 CrossRef CAS PubMed;
(f) S. M. Norbe and A. L. Monterio, Tetrahedron Lett., 2004, 45, 8225 CrossRef;
(g) H. Doucet, M. Santelli and L. Chahen, Synlett, 2003, 1668 CrossRef From benzyl carbonates:
(h) Y. Ohtake, T. Emura, M. Nishimoto, K. Takano, K. Yamamoto, S. Tsuchiya, S.-Y. Yeu, Y. Kito, N. Kimura, S. Takeda, M. Tsukazaki, M. Murakata and T. Sato, J. Org. Chem., 2016, 81, 2148 CrossRef CAS PubMed;
(i) R. Kuwano and M. Yokogi, Org. Lett., 2005, 7, 945 CrossRef CAS PubMed From benzyl acetates:
(j) G. W. Stewart, P. E. Maligres, C. A. Baxter, E. M. Junker, S. W. Krska and J. P. Scott, Tetrahedron, 2016, 72, 3701 CrossRef CAS;
(k) R. Kuwano and M. Yokogi, Chem. Commun., 2005, 5899 RSC.
- M. Ohsumi and N. Nishiwaki, ACS Omega, 2017, 2, 7767 CrossRef CAS.
- Suzuki–Miyaura coupling using other benzylic systems were also reported; however, selective coupling reaction using different reactivity has not been studied. From benzyl pivalates:
(a) Q. Chen, X.-H. Fan, L. P. Zhang and L.-M. Yang, RSC Adv., 2015, 5, 15338 RSC From benzyl carbamates:
(b) X.-X. Wang, M.-J. Luo and J.-M. Lu, Org. Biomol. Chem., 2015, 13, 11438 RSCFrom benzyl ethers:
(c) M. Tobisu, J. Zhao, H. Kinuta, T. Furukawa, T. Igarashi and N. Chatani, Adv. Synth. Catal., 2016, 358, 2417 CrossRef CAS;
(d) M. Tobisu, A. Yasutome, H. Kinuta, K. Nakamura and N. Chatani, Org. Lett., 2014, 16, 5572 CrossRef CAS PubMed; From benzyl sulfonates:
(e) G. Wu, S. Xu, Y. Deng, C. Wu, X. Zhao, W. Ji, Y. Zhang and J. Wang, Tetrahedron, 2016, 72, 8022 CrossRef CAS;
(f) X.-X. Wang, B.-B. Xu, W.-T. Song, K.-X. Sun and J.-M. Lu, Org. Biomol. Chem., 2015, 13, 4925 RSC From benzyl sulfones:
(g) M. Nambo, E. C. Keske, J. P. G. Rygus, J. C.-H. Yim and C. M. Crudden, ACS Catal., 2017, 7, 1108 CrossRef CAS From benzyl phosphates:
(h) K. Liu, W. Rao, H. Parikh, Q. Li, T. L. Guo, S. Grant, G. E. Kellogg and S. Zhang, Eur. J. Med. Chem., 2012, 47, 125 CrossRef CAS PubMed;
(i) M. McLaughlin, Org. Lett., 2005, 7, 4875 CrossRef CAS PubMed; From phenylacetate:
(j) K. Muto, J. Yamaguchi, D. G. Musaev and K. Itami, Nat. Commun., 2015, 6, 7508 CrossRef PubMed.
- K. Das, R. Shibuya, Y. Nakahara, N. Germain, T. Ohshima and K. Mashima, Angew. Chem., Int. Ed., 2012, 51, 150 CrossRef CAS PubMed.
- B. R. P. Reddy, S. Chowdhury, A. Auffrant and C. Gosmini, Adv. Synth. Catal., 2018, 360, 3026 CrossRef CAS.
- R. J. Procter, J. J. Dunsford, P. J. Rushworth, D. G. Hulcoop, R. A. Layfield and M. J. Ingleson, Chem.–Eur. J., 2017, 23, 15889 CrossRef CAS PubMed.
- F. Forster, T. T. Metsänen, E. Irran, P. Hrobárik and M. Oestreich, J. Am. Chem. Soc., 2017, 139, 16334 CrossRef CAS PubMed.
- G. Schäfer and J. W. Bode, Angew. Chem., Int. Ed., 2011, 50, 10913 CrossRef PubMed.
- Y. Sabnis, P. J. Rosenthal, P. Desai and M. A. Avery, J. Biomol. Struct. Dyn., 2002, 19, 765 CrossRef CAS PubMed.
- P. Tundo, C. R. McElroy and F. Aricò, Synlett, 2010, 10, 1567 CrossRef.
- M. J. Frisch, G. W. Trucks, H. B. Schlegel, G. E. Scuseria, M. A. Robb, J. R. Cheeseman, G. Scalmani, V. Barone, G. A. Petersson, H. Nakatsuji, X. Li, M. Caricato, A. V. Marenich, J. Bloino, B. G. Janesko, R. Gomperts, B. Mennucci, H. P. Hratchian, J. V. Ortiz, A. F. Izmaylov, J. L. Sonnenberg, D. Williams-Young, F. Ding, F. Lipparini, F. Egidi, J. Goings, B. Peng, A. Petrone, T. Henderson, D. Ranasinghe, V. G. Zakrzewski, J. Gao, N. Rega, G. Zheng, W. Liang, M. Hada, M. Ehara, K. Toyota, R. Fukuda, J. Hasegawa, M. Ishida, T. Nakajima, Y. Honda, O. Kitao, H. Nakai, T. Vreven, K. Throssell, J. A. Montgomery Jr, J. E. Peralta, F. Ogliaro, M. J. Bearpark, J. J. Heyd, E. N. Brothers, K. N. Kudin, V. N. Staroverov, T. A. Keith, R. Kobayashi, J. Normand, K. Raghavachari, A. P. Rendell, J. C. Burant, S. S. Iyengar, J. Tomasi, M. Cossi, J. M. Millam, M. Klene, C. Adamo, R. Cammi, J. W. Ochterski, R. L. Martin, K. Morokuma, O. Farkas, J. B. Foresman and D. J. Fox, Gaussian 16, Revision A.03, Gaussian, Inc., Wallingford, CT, 2016 Search PubMed.
- A. D. Becke, J. Chem. Phys., 1993, 98, 5648 CrossRef CAS.
- C. Lee, W. Yang and R. G. Parr, Phys. Rev. B, 1988, 37, 785 CrossRef CAS.
- P. J. Hay and W. R. Wadt, J. Chem. Phys., 1985, 82, 299 CrossRef CAS.
- R. Dennington, T. Keithand, and J. Millam, GaussView, Version 6, Semichem Inc, Shawnee Mission, KS, 2016 Search PubMed.
Footnote |
† Electronic supplementary information (ESI) available: Experimental data for optimization of the reaction conditions, for chemoselective coupling reactions using other substrates; copies of 1H and 13C NMR spectra. See DOI: 10.1039/c8ra07841f |
|
This journal is © The Royal Society of Chemistry 2018 |
Click here to see how this site uses Cookies. View our privacy policy here.