DOI:
10.1039/C8RA07834C
(Paper)
RSC Adv., 2018,
8, 34088-34093
Thioether-functionalized trifluoromethyl-alkynes, 1,3-dienes and allenes: divergent synthesis from reaction of 2-trifluoromethyl-1,3-conjugated enynes with sulfur nucleophiles†
Received
20th September 2018
, Accepted 27th September 2018
First published on 4th October 2018
Abstract
A divergent synthesis of thioether-functionalized trifluoromethyl-alkynes, 1,3-dienes and allenes via regioselective nucleophilic addition of sulfur nucleophiles to 2-trifluoromethyl-1,3-conjugated enynes was developed. The addition patterns depend on the type of enyne, sulfur nucleophile and reaction conditions used. 1,4-Addition leading to thioether-functionalized trifluoromethyl-allenes was realized when enynes possessing electron-withdrawing aryl groups on the alkyne moiety were used as reaction partners and alkanethiols were used as nucleophiles, whereas solvent-controlled construction of thioether-functionalized 1,3-dienes and alkynes was realized, respectively, via a 3,4-addition pattern or 1,2-addition pattern if thiophenols were applied as nucleophiles. The three types of compounds containing both sulfur and fluorine elements are valuable building blocks for synthesis of multifunctional fluorinated vinyl sulfides and thiophene derivatives.
Introduction
Owing to their unique structural properties and reactivity, allene chemistry,1 alkyne chemistry,2 as well as 1,3-diene chemistry,3 have attracted particular attention by chemists in the past few decades. They have proven themselves to be important and valuable building blocks toward complex molecular targets in organic synthesis. As a consequence, substantial efforts have been made over the years by synthetic organic chemists in order to design and develop methods allowing efficient access to a variety of functionalized allenes,4 alkynes5 and 1,3-dienes.6 Meanwhile, the widespread use of fluorinated, especially trifluoromethylated, compounds in pharmaceutical chemistry, agrochemistry7 and materials science8 has attracted considerable interest in development of various synthetic methodologies for synthesis of these trifluoromethylated species.9 In this context, special attention has naturally been brought to trifluoromethyl-allene,10 alkyne11 and 1,3-diene12 building blocks and how to access them.
During the last few years, our research group and others have demonstrated that 2-trifluoromethyl-1,3-conjugated enynes are readily available fluorinated building blocks for the synthesis of fluorinated heterocycles and carboncycles. They could act as four- or two- carbon components in palladium catalyzed intermolecular formal [4 + 2] or [3 + 2] cycloaddition reactions for trifluoromethyl benzenes or exomethylene cyclopentane derivatives synthesis (Scheme 1a).13 They also could serve as novel electrophiles to react with bisnucleophiles such as hydroxylamine, primary amine, aminomalonate and 1,3-dicarbonyl compounds for various fluorinated heterocycles synthesis such as pyrrole, N-hydroxypyrrole, isoxazole, cyclic nitrone, pyrroline, pyrrolidine and fluorinated carboncycles synthesis such as cyclopentene derivatives, etc. in transition metal catalysed or simple base mediated cascade reactions (Scheme 1b).14
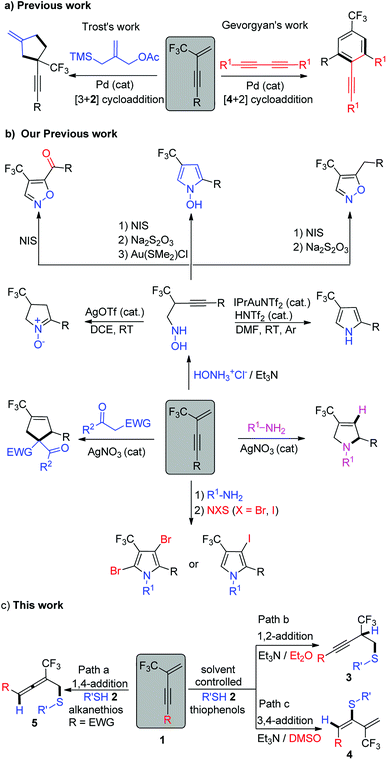 |
| Scheme 1 Divergent synthesis from 2-trifluoromethyl-1,3-conjugated enynes. | |
Sulfur-containing compounds (SCCs) have important applications in pharmaceuticals,15 materials,16 and foods.17 Within this context, we became interested in the regioselective nucleophilic addition of sulfur nucleophiles to 2-trifluoromethyl-1,3-conjugated enynes to construct useful building blocks containing both sulfur and fluorine elements, which are both leading constituents of the pharmaceuticals that comprise our medicinal history.15a We herein report our investigation toward this aim and found that 1,4-addition leading to thioether-functionalized trifluoromethyl-allenes was realized when enynes possessing electron-withdrawing aryl groups on the alkyne moiety were used as reaction partner and alkanethiols were used as nucleophiles, whereas solvent-controlled construction of functionalized 1,3-dienes and alkynes were realized, respectively, via 3,4-addition pattern or 1,2-addition pattern if thiophenols were applied as nucleophiles (Scheme 1c).
Results and discussion
We initiated this project using enyne 1a and 4-methyl-thiophenol 2a as model substrates for screening reaction conditions. Under base free conditions, no reaction occurred, the result prompted us to test base as additives. Considering that the replacement of the alkaline metal ion with ammonium cation formed by deprotonation of hydrosulfuryl of thiophenol will weaken the metalfluorine interaction, thus suppress the defluorination process,18,19 we choose organic base such as tertiary amine for reaction screening (for detailed reaction conditions screening, please see ESI Table 1†). During our initial screens, we found that two main regioselective nucleophilic adducts, that is, functionalized alkyne 3aa via 1,2-addition pattern and functionalized 1,3-diene 4aa via 3,4-addition pattern were formed, which relied heavily on the solvent and base used. In order to control regioselectivity and to have the optimized reaction conditions for the formation of 3aa and 4aa, respectively, extensive optimizations were performed. After many attempts, we were pleased to find that the combination of Et3N as base and ether as solvent was found to be the optimal reaction conditions for 1,2-nucleophilic addition pattern, and functionalized alkyne 3aa could be obtained in 77% isolated yield. Interestingly, simply use DMSO instead of ether as solvent invert the regioselectivity to 3,4-nucleophilic addition pattern, and functionalized 1,3-diene 4aa could be obtained in 80% isolated yield. We hypothesize that thiophenol has a relatively high acidity and is easily ionized with increasing nucleophilicity in a polar solvent with relatively high dielectric constant such as DMSO compared with Et2O under basic reaction conditions,20 which facilitate both allene intermediate formation and subsequent stereospecific nucleophilic addition to allene and β-heteroatom elimination tandem process, leading to functionalized 1,3-diene formation.21
With the optimal reaction conditions established, the enyne substrate scope as well as thiophenol substrate scope were then examined and demonstrated with sixteen examples for these two regioselective nucleophilic addition patterns, respectively, as shown in Scheme 2. There are several points that are noteworthy: (1) in most cases, the reactions are solvent-controlled regioselectivity, functionalized alkynes 3 and 1,3-dienes 4 are two predominate adducts while the amount of the allene 5 is negligible, which accounts for the mass balance; (2) in one case for synthesis of functionalized alkynes 3ha, enyne 1h bearing strong electron-withdrawing group such as nitro group in para-position of the benzene ring on the alkyne moiety exhibits substrate-controlled regioselectivity and allene 5ha is formed predominately (61% isolated yield); (3) enynes 1e, 1f bearing relatively weakly electron-withdrawing group such as acyl, nitrile group afford functionalized alkynes 3ea, 3fa in 22%, 33% isolated yield along with the formation of 1,3-butadiene 4ea and 4fa in 60%, 52% isolated yield, respectively; (4) in most cases, both enyne and thiophenol show good functional groups tolerance for 3,4-nucleophilic addition pattern, and good to excellent yields of the 1,3-butadiene products could be obtained with high stereoselectivity, most of addition products were observed as single double bond E-isomers (4aa–4da, 4ia–4ja, 4ab–4ag), and (5) the structure of compound 4ag was further confirmed by means of single-crystal X-ray crystallography (Fig. 1);22 (6) owing to its insolubility in ether, 4-nitrobenzenethiol 2g failed to afford corresponding alkyne 3ag in ether, however, it reacts well with enyne 1a in DMSO to afford corresponding 1,3-diene 4ag in 84% isolated yield.
|
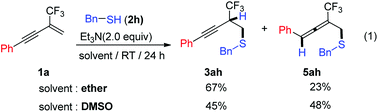 | (1) |
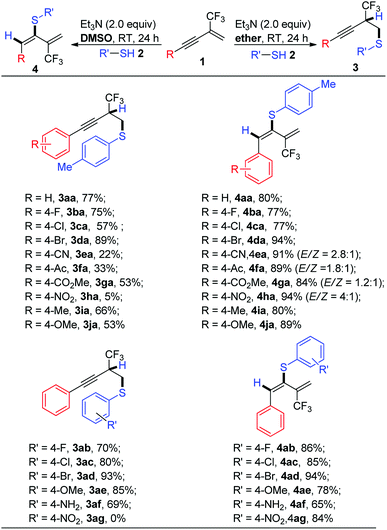 |
| Scheme 2 Scope of 2-trifluoromethyl-1,3-enynes 1 and thiophenols 2 for synthesis of thioether-functionalized trifluoromethyl-alkynes 3 and 1,3-dienes 4. | |
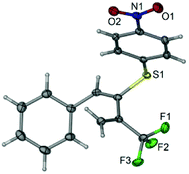 |
| Fig. 1 ORTEP depiction of compound 4ag. | |
Next, we evaluated the use of alkanethiol instead of thiophenol as nucleophile. Initially, benzyl mercaptan 2h was selected as a representative alkanethiol reagent to react with enyne 1a under above two optimal reaction conditions. It is interesting to note that regioselective nucleophilic addition pattern varied from 1,2-/3,4-addition pattern to 1,2-/1,4-addition pattern and functionalized alkynes 3ah and allene 5ah were two predominately formed adducts according to crude 1H NMR analysis, while the amount of the 1,3-diene 4ah was negligible [eqn (1)]. The interesting results prompted us to further explore optimal reaction conditions for allene synthesis. Based on the formation of allene 5ha and our previously published paper for cyclopentene synthesis,14f concerning the significant role of electronic effects in allene formation, enyne 1g with an ester substituent was selected as model substrate to react with benzyl mercaptan 2h for optimal reaction conditions screening. After numerous attempts, we were pleased to find that allene 5gh could be obtained in 75% isolated yield when reaction was performed in the co-solvent of DMSO and CHCl3 (v/v = 1
:
1) at room temperature by using 10% TMEDA as catalytic base (TMEDA = N,N,N′,N′-tetramethyl-ethane-1,2-diamine) (see ESI Table 2†).
With these results in hand, the enyne substrate scope as well as alkanethiol scope were then carried out (Scheme 3). Points to note: (1) in general, enynes possessing electron-withdrawing aryl group on the alkyne moiety are general good candidates for allene formation and the desired thioether-functionalized-allenes could be obtained in moderate to good yield (5gh–5ht); (2) although 1,4-addition pattern proceeded well with alkanethiols and enyne bearing electron-withdrawing groups, product mixture were generally observed and functionalized alkynes were predominated by-products; (3) a variety of substituted benzyl thiols, including hindered substrate underwent 1,4-addition smoothly and corresponding functionalized allenes could be obtained in moderate to good yield (5hi–5hp); (4) both primary and secondary alkanethiol (5ht) as well as tertiary alkanethiol (5hs) are suitable nucleophiles. Using alkanethiols as nucleophiles, 3,4-nucleophilic addition pattern leading to functionalized 1,3-diene was suppressed. We hypothesize that this may be due to its relatively low acidity and hardly ionization in DMSO compared with thiophenols,20 which reduces its ability to nucleophilic attack to allene intermediate to form functionalized 1,3-diene.21
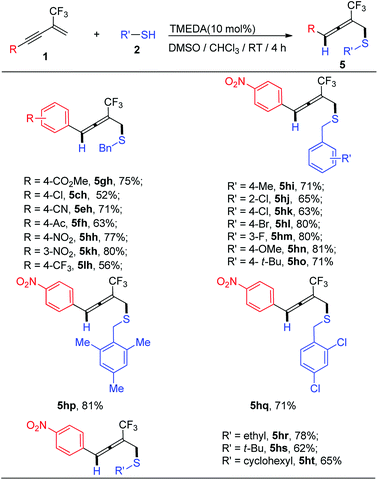 |
| Scheme 3 Scope of 2-trifluoromethyl-1,3-enynes 1 and aliphatic thios 2 for synthesis of thioether-functionalized trifluoromethyl-allenes 5. | |
To highlight the synthetic utilities of present transformation, several selective transformations of the representative functionalized alkyne 3aa, 1,3-diene 4aa as well as functionalized allenes 5eh and 5hh are shown in Scheme 4. The results show that they are versatile organic building blocks in organic synthesis. For example, upon treatment of 3aa with molecule iodine, a I+ induced electrophilic cyclization and subsequent I− induced ring-opening reaction occurred and diiodic compound 6 featured with alkenyl and alkyl iodide was obtained in 50% isolated yield, which was further confirmed by an X-ray crystallography analysis.22 Interestingly, synthetically valuable β-halo alkenyl sulfides 7 or 8 could be delivered in quantitative yield but with poor stereoselectivity by the treatment of 3aa with N-halosuccinimides (NXS). As we know, vinyl sulfides have wide applications in organic synthesis, material science and pharmaceutics, thus, novel and efficient methods for constructing them are still of contemporary importance to the synthetic community and have received continuous attention.23 Notably, functionalized 1,3-diene can also serve as novel electrophiles for the nucleophilic addition. 1,3-Diene 4aa can react readily with benzylamine to afford multifunctional tetrasubstituted alkenes 10 in 86% isolated yield via a consecutive regioselective nucleophilic addition followed by double bond isomerization. Furthermore, 1,3-diene 4aa could undergo Diels–Alder reaction with N-phenyl-maleimidein in chlorobenzene at 150 °C, yielding the corresponding cycloaddition product 9 in 62% isolated yield with moderate diastereoselectivity. It is also important to highlight that halogenated trifluoromethylated thiophenes 11–13 could be delivered in good isolated yield upon treatment of allene 5eh, 5hh with three equivalents of N-halosuccinimide (NXS) in toluene at room temperature via sequential electrophilic cyclization/oxidation/debenzylation cascade process.24
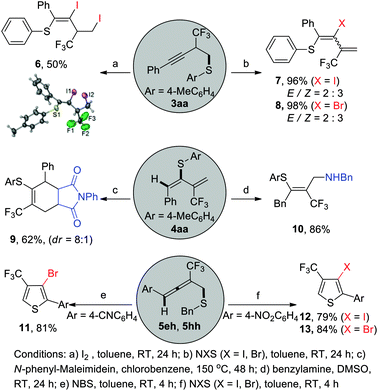 |
| Scheme 4 Synthetic transformation of alkyne 3aa, 1,3-diene 4aa and allenes 5eh, 5hh. | |
Conclusions
In summary, we have discovered the divergent synthesis of thioether-functionalized trifluoromethyl-alkynes, 1,3-dienes and allenes from the regioselective nucleophilic addition reactions of 2-trifluoromethyl-1,3-conjugated enynes with sulfur nucleophiles. The addition patterns depend on the type of enynes, sulphur nucleophiles and reaction conditions used. The three types of compounds containing both sulfur and fluorine elements are valuable building blocks for synthesis of multifunctional fluorinated vinyl sulfides and thiophenes derivatives.
Experimental
General procedure for the synthesis of thioether-functionalized trifluoromethyl-alkynes 3
To the solution of 2-trifluoromethyl 1,3-conjugated enynes 1 (1.0 mmol), thiophenol 2 (1.5 mmol) in toluene (5.0 mL) under nitrogen at room temperature was added Et3N (2.0 mmol), the reaction was stirred at room temperature for 24 h. After 1 was completely consumed, which was determined by TLC analysis, the solvent was removed under reduced pressure and the crude reaction mixture was purified by flash column chromatography on silica gel (petroleum ether
:
ethyl acetate = 1
:
0–5
:
1) to give the desired 3.
General procedure for the synthesis of thioether-functionalized trifluoromethyl-1,3-dienes 4
To the solution of 2-trifluoromethyl 1,3-conjugated enynes 1 (1.0 mmol), thiophenol 2 (1.1 mmol) in DMSO (5.0 mL) under nitrogen at room temperature was added Et3N (2.0 mmol), the reaction was stirred at room temperature for 24 h. After 1 was completely consumed, which was determined by TLC analysis, water (15 mL) was added to reaction mixture and extracted with ethyl acetate (3 × 5 mL). Organic layers were combined, washed with brine (4.0 mL) and dried over MgSO4, after filtration and evaporation, the residue was purified through flash column chromatography on silica gel (petroleum ether
:
ethyl acetate = 1
:
0–5
:
1) to give the desired 4.
General procedure for the synthesis of thioether-functionalized trifluoromethyl-allenes 5
To the solution of benzyl mercaptan 2 (1.0 mmol) in DMSO/CHCl3 (v/v = 1
:
1, 5.0 mL) under nitrogen at room temperature was added TMEDA (0.1 mmol), then added 2-trifluoromethyl 1,3-conjugated enynes 1 (1.6 mmol), the reaction was stirred at room temperature for 4 h. After 2 was completely consumed, which was determined by TLC analysis, water (2.0 mL) was added and the reaction mixture was extracted with ethyl acetate (3 × 4.0 mL). The combined organic extracts were washed with brine (4.0 mL) and dried over MgSO4. After filtration and evaporation, the residue was purified by flash column chromatography on silica gel (petroleum ether
:
ethyl acetate = 100
:
0–50
:
1) to give the desired 5.
Conflicts of interest
There are no conflicts to declare.
Acknowledgements
We are grateful to 973 Programs (2015CB856600), the National Natural Science Foundation of China (21372084, 21425205, 21871093), STCSM (17ZR1408800) and Changjiang Scholars and Innovative Research Team in University (PCSIRT) for financial supports.
Notes and references
-
(a) N. Krause and A. S. K. Hashmi, Modern Allene Chemistry, Wiley-VCH, Weinheim, 2004 CrossRef;
(b) J. Ye and S. Ma, Acc. Chem. Res., 2014, 47, 989 CrossRef CAS PubMed;
(c) S. Yu and S. Ma, Angew. Chem., Int. Ed., 2012, 51, 3074 CrossRef CAS PubMed;
(d) For a series of reviews on the reactivity of allenes, see: Progress in allene chemistry, Chem. Soc. Rev. 2014, 43, 2879 Search PubMed.
-
(a) B. M. Trost and Ch.-J. Li, Modern Alkyne Chemistry, Wiley-VCH, Weinheim, 2015 Search PubMedFor selected reviews on the reactivity of fuctionalized alkynes, see:
(b) L. Zhang, G. Fang, R. K. Kumar and X. Bi, Synthesis, 2015, 47, 2317 CrossRef CAS;
(c) A. Duefert and D. B. Werz, Chem.–Eur. J., 2016, 22, 16718 CrossRef CAS PubMed;
(d) B. Wagner, K. Belger, S. Minkler, V. Belting and N. Krause, Pure Appl. Chem., 2016, 88, 391 CAS;
(e) H. Yoshida, ACS Catal., 2016, 6, 1799 CrossRef CAS;
(f) M. Patel, R. K. Saunthwal and A. K. Verma, Acc. Chem. Res., 2017, 50, 240 CrossRef CAS PubMed.
-
(a) H. Li, S. Liu and L. S. Liebeskind in Copper-Mediated Cross-Coupling Reactions, ed. G. Evano and N. Blanchad, John Wiley & Sons, Inc, Hoboken, New. Jersey, 2014, p. 485 Search PubMedFor selected reviews on the reactivity of fuctionalized 1,3-dienes, see:
(b) E. McNeill and T. Ritter, Acc. Chem. Res., 2015, 48, 2330 CrossRef CAS PubMed;
(c) P. Margaretha, Helv. Chim. Acta, 2014, 97, 1027 CrossRef CAS;
(d) S. V. Kostjuk, RSC Adv., 2015, 5, 13125 RSC.
- For reviews on synthesis of functionalized allene, see:
(a) K. M. Brummond and J. E. Deforrest, Synthesis, 2007, 795 CrossRef CAS;
(b) A. Hoffmann-Röder and N. Krause, Angew. Chem., Int. Ed., 2002, 41, 2933 CrossRef;
(c) D. Tejedor, G. Mendez-Abt, L. Cotos and F. Garcia-Tellado, Chem. Soc. Rev., 2013, 42, 458 RSC ; For selected recent examples, see;
(d) Y. Yang, Z. Liu, A. Porta, G. Zanoni and X. Bi, Chem.–Eur. J., 2017, 23, 9009 CrossRef CAS PubMed;
(e) M. Schaarschmidt and K. T. Wanner, J. Org. Chem., 2017, 82, 8371 CrossRef CAS PubMed;
(f) Y. Jiang, A. B. Diagne, R. J. Thomson and S. E. Schaus, J. Am. Chem. Soc., 2017, 139, 1998 CrossRef CAS PubMed.
- For selected reviews on synthesis of functionalized alkyne, see:
(a) E. Bernoud, R. Veillard, C. Alayrac and A. C. Gaumont, Molecules, 2012, 17, 14573 CrossRef CAS PubMed;
(b) K. Lauder, A. Toscani, N. Scalacci and D. Castagnolo, Chem. Rev., 2017, 117, 14091 CrossRef CAS PubMed.
- For selected reviews on synthesis of functionalized 1,3-diene, see:
(a) A. Deagostino, C. Prandi, C. Zavattaro and P. Venturello, Eur. J. Org. Chem., 2006, 2463 CrossRef CAS;
(b) J. M. Monbaliu, K. G. R. Masschelein and C. V. Stevens, Chem. Soc. Rev., 2011, 40, 4708 RSC . For selected recent examples;
(c) W. Li and J. Zhang, Org. Lett., 2014, 16, 162 CrossRef CAS PubMed;
(d) A. M. Olivares and D. J. Weix, J. Am. Chem. Soc., 2018, 140, 2446 CrossRef CAS PubMed;
(e) D. Fiorito, S. Folliet, Y. Liu and C. Mazet, ACS Catal., 2018, 8, 1392 CrossRef CAS.
-
(a) V. Gouverneur and K. Muller, Fluorine in Pharmaceutical and Medicinal Chemistry: From Biophysical Aspects to Clinical Applications, Imperial College Press, London, 2012 CrossRef;
(b) J. Wang, M. Sanchez-Rosello, J. N. Acena, C. del Pozo, A. E. Sorochinsky, S. Fustero, V. A. Soloshonok and H. Liu, Chem. Rev., 2014, 114, 2432 CrossRef CAS PubMed;
(c) F. Meyer, Chem. Commun., 2016, 52, 3077 RSC;
(d) Y. Zhou, J. Wang, Z. Gu, S. Wang, W. Zhu, J. L. Aceña, V. A. Soloshonok, K. Izawa and H. Liu, Chem. Rev., 2016, 116, 422 CrossRef CAS PubMed.
- For reviews:
(a) R. Berger, G. Resnati, P. Metrangolo, E. Weber and J. Hulliger, Chem. Soc. Rev., 2011, 40, 3496 RSC;
(b) N. V. S. Dinesh, K. Bhupathiraju, W. Rizvi, J. D. Batteas and C. M. Drain, Org. Biomol. Chem., 2016, 14, 389 RSC.
- For selected recent reviews, see:
(a) S. Barata-Vallejo, B. Lantano and A. Postigo, Chem.–Eur. J., 2014, 20, 16806 CrossRef CAS PubMed;
(b) L. Chu and F.-L. Qing, Acc. Chem. Res., 2014, 47, 1513 CrossRef CAS PubMed;
(c) C. Alonso, E. Martinez de Marigorta, G. Rubiales and F. Palacios, Chem. Rev., 2015, 115, 1847 CrossRef CAS PubMed.
- For selected recent examples for trifluoromethyl-allenes synthesis, please see:
(a) T. S. N. Zhao and K. J. Szabó, Org. Lett., 2012, 14, 3966 CrossRef CAS PubMed;
(b) B. R. Ambler, S. Peddi and R. A. Altman, Org. Lett., 2015, 17, 2506 CrossRef CAS PubMed;
(c) A. Boreux, G. H. Lonca, O. Riant and F. Gagosz, Org. Lett., 2016, 18, 5162 CrossRef CAS PubMed;
(d) J.-L. Li, X.-J. Yang, M. Jiang and J.-T. Liu, Tetrahedron Lett., 2017, 58, 3377 CrossRef CAS.
- For a recent review concerning trifluoromethylated alkynes synthesis, see:
(a) T. Konno, Synlett, 2014, 25, 1350 CrossRef ; for selected examples;
(b) X. Jiang, L. Chu and F.-L. Qing, J. Org. Chem., 2012, 77, 1251 CrossRef CAS PubMed;
(c) A. Sanz-Marco, G. Blay, C. Vila and J. R. Pedro, Org. Lett., 2016, 18, 3538 CrossRef CAS PubMed.
-
(a) S. A. Pujai, K. P. Kaliapan, A. Valleix, D. Gree and R. Gree, Synlett, 2008, 2503 Search PubMed;
(b) F. A. Akkerman, R. Kickbusch and D. Lentz, Chem.–Asian J., 2008, 3, 719 CrossRef CAS PubMed;
(c) T. Hayashi, Y. Usuki, Y. Wakamatsu and H. Iio, Synlett, 2010, 2843 CAS;
(d) Q. Zhao, V. Tognetti, L. Joubert, T. Besset, X. Pannecoucke, J.-P. Bouillon and T. Poisson, Org. Lett., 2017, 19, 2106 CrossRef CAS PubMed.
-
(a) B. M. Trost and L. Debien, J. Am. Chem. Soc., 2015, 137, 11606 CrossRef CAS PubMed;
(b) O. V. Zatolochnaya and V. Gevorgyan, Org. Lett., 2013, 15, 2562 CrossRef CAS PubMed.
-
(a) Q. Zeng, L. Zhang, J. Yang, B. Xu, Y. Xiao and J. Zhang, Chem. Commun., 2014, 50, 4203 RSC;
(b) L. Zhang, Q. Zeng, A. Mao, Z. Wu, T. Luo, Y. Xiao and J. Zhang, Org. Biomol. Chem., 2014, 12, 8942 RSC;
(c) J. Yang, X. Zhou, Y. Zeng, C. Huang, Y. Xiao and J. Zhang, Org. Biomol. Chem., 2017, 15, 2253 RSC;
(d) X. Zhou, C. Huang, Y. Zeng, J. Xiong, Y. Xiao and J. Zhang, Chem. Commun., 2017, 53, 1084 RSC;
(e) Ch.-Q. Huang, Y. Zeng, H. Cheng, A. Hu, L. Liu, Y. Xiao and J. Zhang, Org. Lett., 2017, 19, 4968 CrossRef CAS PubMed;
(f) Y. Zeng, Ch.-Q. Huang, P. Ni, L. Liu, Y. Xiao and J. Zhang, Adv. Synth. Catal., 2017, 359, 3555 CrossRef CAS;
(g) Q. Zeng, L. Zhang, Y. Xiao and J. Zhang, Org. Biomol. Chem., 2018, 16, 1375 RSC.
- For recent reviews:
(a) E. A. Ilardi, E. Vitaku and J. T. Njardarson, J. Med. Chem., 2014, 57, 2832 CrossRef CAS PubMed;
(b) M. Feng, B. Tang, H. L. Steven and X. Jiang, Curr. Top. Med. Chem., 2016, 16, 1200 CrossRef CAS PubMed.
- For recent reviews:
(a) D. P. Nair, M. Podg_rski, S. Chatani, T. Gong, W. Xi, C. R. Fenoli and C. N. Bowman, Chem. Mater., 2014, 26, 724 CrossRef CAS;
(b) M. E. Cinar and T. Ozturk, Chem. Rev., 2015, 115, 3036 CrossRef CAS PubMed.
-
(a) E. Block, Angew. Chem., Int. Ed. Engl., 1992, 31, 1135 CrossRef;
(b) D. Y. Lin, S.-Z. Zhang, E. Block and L. C. Katz, Nature, 2005, 434, 470 CrossRef CAS PubMed.
- K. Uneyama, T. Katagiri and H. Amii, Acc. Chem. Res., 2008, 41, 817 CrossRef CAS PubMed.
- For reviews on addition reactions towards fluorine-containing alkenes, please see:
(a) J. Ichikawa, J. Synth. Org. Chem., Jpn., 2010, 68, 1175 CrossRef CAS;
(b) J. Ichikawa, Chim. Oggi, 2007, 25, 54 CAS.
- F. G. Bordwell, Acc. Chem. Res., 1988, 21, 456 CrossRef CAS.
- X. Yu, H. Ren, Y. Xiao and J. Zhang, Chem.–Eur. J., 2008, 14, 8481 CrossRef CAS PubMed.
- X-Ray data and ORTEP depiction for compounds 4ag (CCDC 1867549) and 6 (CCDC 1860794) please see ESI.†.
- For reviews, please see:
(a) C. Shen, P. Zhang, Q. Sun, S. Bai, T. S. A. Hor and X. Liu, Chem. Soc. Rev., 2015, 44, 291 RSC;
(b) R. Chinchilla and C. Nájera, Chem. Rev., 2014, 114, 1783 CrossRef CAS PubMed ; for recent examples;
(c) T. Inami, T. Kurahashi and S. Matsubara, Chem. Commun., 2015, 51, 1285 RSC;
(d) Y. Lin, G. Lu, C. Cai and W. Yi, Org. Lett., 2015, 17, 3310 CrossRef CAS PubMed;
(e) J. Chen, S. Chen, X. Xu, Z. Tang, C.-T. Au and R. Qiu, J. Org. Chem., 2016, 81, 3246 CrossRef CAS PubMed.
- A. S. Santana, D. B. Carvalho, N. S. Cassemiro, L. H. Viana, G. R. Hurtado, M. S. Amaral, N. M. Kassab, P. G. Guerrero Jr, S. L. Barbosa, M. J. Dabdoub and A. C. M. Baroni, Tetrahedron Lett., 2014, 55, 52 CrossRef CAS.
Footnote |
† Electronic supplementary information (ESI) available. CCDC 1860794 and 1867549. For ESI and crystallographic data in CIF or other electronic format see DOI: 10.1039/c8ra07834c |
|
This journal is © The Royal Society of Chemistry 2018 |
Click here to see how this site uses Cookies. View our privacy policy here.