DOI:
10.1039/C8RA07647B
(Paper)
RSC Adv., 2018,
8, 37064-37068
An efficient nickel/silver co-catalyzed remote C–H amination of 8-aminoquinolines with azodicarboxylates at room temperature†
Received
14th September 2018
, Accepted 30th October 2018
First published on 5th November 2018
Abstract
A highly efficient nickel/silver co-catalyzed C–H amination at the C5 position of 8-aminoquinolines with azodicarboxylates at room temperature is reported. The reaction undergoes a self-redox process without the necessity of external oxidant. It proceeded under simple and mild conditions without any additional ligand, base or oxidant and provided the desired products in good to excellent yields. This method also possessed the merits of good functional group compatibility and air and moisture tolerance. It provides an efficient strategy for the synthesis of useful quinoline derivatives.
Introduction
Arylamines are of great importance as they widely exist in pharmaceuticals, agrochemicals, dyes, herbicides, and functional materials.1 Therefore, the development of efficient methods for C–N bond construction has captured much attention. During the past decade, transition metal-catalyzed C–H bond amination has become a reliable and powerful tool for C–N bond formation in organic synthesis.2 Quinoline is one of the key heteroaryl skeletons as it has been found in many natural products, pharmaceuticals and agrochemicals.3 As a facile and efficient method for the synthesis of substituted quinolines, direct substitution of quinolines through C–H functionalization has been widely investigated in recent years.4 C–H functionalization on the C5 position of quinoline scaffolds still remains a challenge and has attracted much attention.4,5 The C5 amino-substituted quinolines have shown important antimalarial and anticancer activities and been applied in biological and pharmacological studies.6 Efforts have also been devoted towards the C5 amination of quinolines through C–H functionalization.
Recently, copper-catalyzed C5 C–H amination of 8-aminoquinolines with N-fluorobenzenesulfonimide (NFSI) or azoles were reported by Liu, Zhang and Lei's groups (Scheme 1a).7 In 2016, metal-free hypervalent iodine-mediated C5 C–H amination of 8-aminoquinoline with NFSI or dibenzenesulfonimides were also developed by our and Xu's groups (Scheme 1a).8 Azodicarboxylates, which contain a central azo functional group, have been used in numerous chemical reactions and emerged as efficient nitrogen source for various C–N bond formation reactions.9 In 2016, Baidya and coworkers reported that copper-catalyzed C5 C–H amination of 8-aminoquinolines with azodicarboxylates (Scheme 1a).10 However, high catalyst loading (10–20 mmol%), stoichiometric amount of external oxidant and elevated reaction temperature (70–100 °C) were normally required to reach high yields in most preceding works.
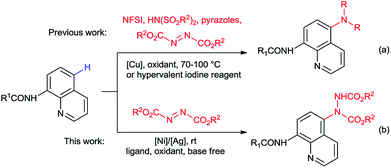 |
| Scheme 1 Direct C–H amination of quinolines on C5 position. | |
Recently, our group was also focused on efficient C5 C–H functionalization of quinolines.8b,11 Along with our studies, we have found that the C5 C–H amination of 8-aminoquinolines with azodicarboxylates could be achieved under a nickel/silver co-catalytic system (Scheme 1b). As a non-precious, earth-abundant and non-toxic metal, nickel has attracted extensive attention in organic synthesis and been used as catalyst in numerous C–H functionalizations.12 However, the examples of nickel-catalyzed C5 C–H functionalizations of quinolines are quite rare to date.13 Examples of silver catalysis in this kind of reactions are much less.14 But the combination of these two metals created amazing results.15 Within this paper, we report a highly efficient nickel/silver co-catalyzed C–H amination on C5 position of 8-aminoquinolines with azodicarboxylates at room temperature. The reactions proceed under simple and mild conditions without any additional ligand, base or oxidant and provide desired products in good to excellent yields.
Results and discussion
Initially study was operated by using N-(8-quinolinyl)acetamide (1a) (0.2 mmol) with diethyl azodicarboxylate (DEAD) (2a) (2 equiv.) as the model system for condition optimization and the results are displayed in Table 1. In the presence of Ni(OTf)2 (10 mol%), amination occurred on the C5 position of the quinoline ring at 80 °C in acetone, affording the desired product (3aa) in 11% isolated yield (Table 1, entry 1). The reaction was handled under air without anhydrous conditions. The structure of 3aa was unambiguously confirmed by X-ray crystallography.16 To our delight, when additional AgCO3 (10 mol%) was introduced the isolated yield of 3aa was dramatically increased to 89% (entry 2). Other silver salts were subsequently investigated. The product yield slightly deceased with AgOAc but improved with AgNO3 (entries 3 and 4). Next we examined different nickel catalyst with AgNO3. Among them, NiBr2 and Ni(PPh3)2Br2 showed poor results but Ni(acac)2 further improved the yield to 93% (entries 5–7). Then we attempted to reduce the catalyst loading. When the amount of Ni and Ag were both declined from 10 mol% to 5 and 3 mol%, the product yields remained high-level (93% and 96%) (entries 8 and 9). With 3 mol% of both Ni and Ag, other four solvents (EtOAc, CH3CN, THF and CH2Cl2) were checked (entries 10–13). Based on the results, this reaction showed excellent suitability of solvents as EtOAc, THF and CH2Cl2 all provide high yields although not better than acetone. From the perspective of green chemistry, acetone is also the best choice among them.17 Furthermore, the reaction temperature can also be decreased without significant influence of product yield (entries 14 and 15). For easy operation, room temperature was preferred which still provide the product in 95% yield (entry 15). Other modulation of reaction condition such as shortening reaction time (12 to 8 h) or reducing reagent amount (2 to 1.5 equiv.) still resulted in considerable high yields (entries 16 and 17). Even decreasing the catalyst loading to 1 mol%, 77% product yield was obtained (entry 18). From all above results, the condition showed in entry 16 was chose as the optimized one.
Table 1 Optimization of the reaction conditionsa
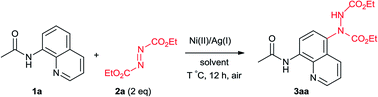
|
Entry |
Ni(II) (mol%) |
Ag(I) (mol%) |
Solvent |
T (°C) |
Yieldb (%) |
Reaction conditions: 1a (0.2 mmol), 2a (0.4 mmol), Ni(II) (10 mol%), Ag(I) (10 mol%) in solvent (2.0 mL) stirring under air for 12 h. Isolated yields. Reaction time 8 h. With 1.5 equiv. 2a. |
1 |
Ni(OTf)2 (10) |
— |
Acetone |
80 |
11 |
2 |
Ni(OTf)2 (10) |
Ag2CO3 (10) |
Acetone |
80 |
86 |
3 |
Ni(OTf)2 (10) |
AgOAc (10) |
Acetone |
80 |
83 |
4 |
Ni(OTf)2 (10) |
AgNO3 (10) |
Acetone |
80 |
89 |
5 |
NiBr2 (10) |
AgNO3 (10) |
Acetone |
80 |
27 |
6 |
Ni(acac)2 (10) |
AgNO3 (10) |
Acetone |
80 |
93 |
7 |
Ni(PPh3)2Br2 (10) |
AgNO3 (10) |
Acetone |
80 |
18 |
8 |
Ni(acac)2 (5) |
AgNO3 (5) |
Acetone |
80 |
93 |
9 |
Ni(acac)2 (3) |
AgNO3 (3) |
Acetone |
80 |
96 |
10 |
Ni(acac)2 (3) |
AgNO3 (3) |
EtOAc |
80 |
87 |
11 |
Ni(acac)2 (3) |
AgNO3 (3) |
CH3CN |
80 |
31 |
12 |
Ni(acac)2 (3) |
AgNO3 (3) |
THF |
80 |
93 |
13 |
Ni(acac)2 (3) |
AgNO3 (3) |
CH2Cl2 |
80 |
88 |
14 |
Ni(acac)2 (3) |
AgNO3 (3) |
Acetone |
40 |
96 |
15 |
Ni(acac)2 (3) |
AgNO3 (3) |
Acetone |
rt |
95 |
16c |
Ni(acac)2 (3) |
AgNO3 (3) |
Acetone |
rt |
93 |
17d |
Ni(acac)2 (3) |
AgNO3 (3) |
Acetone |
rt |
92 |
18 |
Ni(acac)2 (1) |
AgNO3 (1) |
Acetone |
rt |
77 |
Under the optimized conditions, the scope of 8-acylaminoquinolines (1) was examined for the C5 C–H amination with 2a (Table 2). Various alkyl amides were applicable in this reaction to give the desired products in excellent yields (3ba–3ha). The propionamide, butyramide and phenylpropanamide resulted in extremely high yields (3ba–3da, 98–99%). Sterically bulky groups such as isopropyl, tert-butyl, cyclopropyl and cyclohexyl did not hinder the reaction significantly (3ea–3ha). Acrylamide also showed high reactivity for this transformation (3ia). Aryl amides can also be applied and generated the corresponding products efficiently (3ja–3qa). Various functional groups such as MeO, F, Cl, Br and CF3 were tolerable on the benzene ring. Both electron-donating and electron-withdrawing substituted substrates provided excellent results without significant electronic effect. Notably, the o-C–H bonds existed in these benzamides did not influence this transformation at all, which might be activated and become reaction sites under nickel catalysis.12 Moreover, the substrates containing a substituent on the quinoline such as 2-Me, 4-Cl and 6-OMe (3ra–3ta) were also examined. Except the one with a C2 substituent which might hinder the coordination with catalysts, high yields were provided by other substrates.
Table 2 Substrate scope of 8-acylaminoquinolines (1)a,b
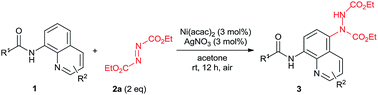
|
Reaction conditions: 1a (0.2 mmol), 2a (0.4 mmol), Ni(OTf)2 (3 mol%), AgNO3 (3 mol%) in acetone (2.0 mL) stirring at 25 °C under air for 12 h. Isolated yields. At 60 °C. |
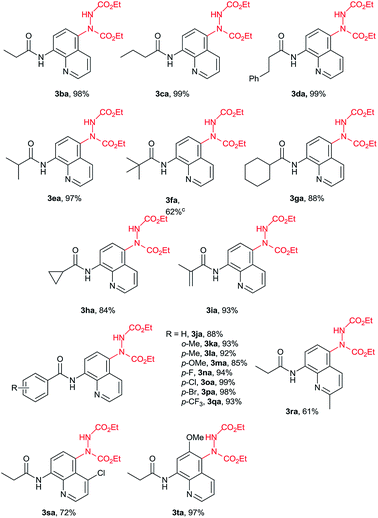 |
Next, the reaction of N-(8-quinolinyl)acetamide (1a) with different azodicarboxylates (2) was investigated as shown in Table 3. The isopropyl, tert-butyl and benzyl-substituted azodicarboxylates all reacted in this method. The tert-butyl azodicarboxylate, which was unreacted in previous method,10 provided a lower yield perhaps due to the steric effect (3ac). The benzyl-substituted azodicarboxylate gave the desired product 3ad in 97% yield while 3ae in 65% with p-chlorobenzyl azodicarboxylate. Unfortunately, azobisisobutyronitrile did not work in this reaction (3af).
Table 3 Substrate scope of azodicarboxylates (2)a,b
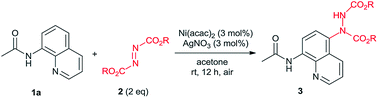
|
Reaction conditions: 1a (0.2 mmol), 2a (0.4 mmol), Ni(OTf)2 (3 mol%), AgNO3 (3 mol%) in acetone (2.0 mL) stirring at 25 °C under air for 12 h. Isolated yields. |
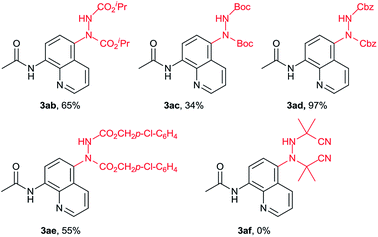 |
Several control experiments were carried out to clarify the reaction mechanism (Scheme 2). Stoichiometric experiments were conducted to illustrate the role of each transition-metal. Sole 1 equivalent of Ni(acac)2 generated 15% yield but 1 equivalent of AgNO3 only afforded trace amount of product. Combination of catalytic amount of Ni(acac)2 and stoichiometric AgNO3 resulted in high yield (Scheme 2a). These outcomes suggested the essential role of nickel as the main catalyst while silver as the co-catalyst which can facilitate the catalytic cycle. The reaction provided similar results either under nitrogen or oxygen atmosphere instead of air, which suggested that oxygen was not necessary (Scheme 2b). Thus this reaction might proceed via a self-redox process. The reaction was significantly inhibited in the presence of a radical scavenge TEMPO or BHT, which implied the involvement of radical intermediates in the reaction pathway (Scheme 2b). Analogous substrates N-(1-naphthalenyl) benzamide (4) and N-methyl-N-(8-quinolinyl)benzamide (5) were unreacted in this method which indicated the essential role of chelation assistance of the N–H bond and the quinoline group. When C5-blocked substrates such as 6 and 7 were used, no amination occurred at C7 or other positions. These results excluded an aromatic electrophilic substitution pathway.
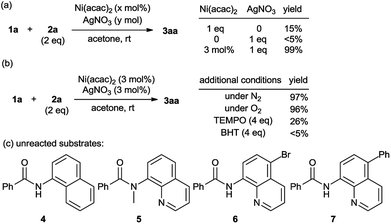 |
| Scheme 2 Control experiments. | |
Although detailed mechanism must await further investigation, a possible mechanism was proposed for this nickel/silver co-catalyzed C–H amination of 8-aminoquinoline based on the above results and previous reports (Scheme 3). Initially, Ni(II) catalyst coordinated with the N-(8-quinolyl)amide (1) through chelation-assistance to generate a Ni(II)-amide intermediate A. Then, the Ni(II) complex A was oxidized to a Ni(III) complex B by Ag(I). Subsequently, single electron transfer from the 8-aminoquinoline moiety to the Ni(III) center resulted in a Ni(II)-quinoline cationic radical species C. The azodicarboxylate (2) reacted with C at the C5 position to generate a Ni(III) intermediate D. Then intermediate D dissociated to provide the product 3 and Ni(III) which was reduced to Ni(II) with Ag(0) under reaction conditions. Thus the silver also finished its redox cycle.
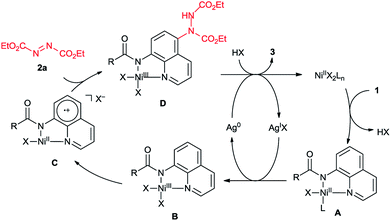 |
| Scheme 3 Plausible mechanism. | |
Conclusions
In summary, we have developed a highly efficient protocol for remote C–H amination of 8-aminoquinolines with azodicarboxylate. In the presence of a nickel/silver co-catalyst, the reaction smoothly proceeded at room temperature under air, affording the C5 aminated products in good to excellent yields. Mechanistic studies indicated that the reaction undergoes a self-redox process without necessary of external oxidant. This method also possessed the merit of good functional group compatibility and air and moisture tolerance. It provides an efficient strategy for the synthesis of useful quinoline derivatives and also provides new ideas of bimetallic catalyst development for the transformation of 8-aminoquinolines.
Conflicts of interest
There are no conflicts to declare.
Acknowledgements
We are grateful to the National Natural Science Foundation of China (Grant No. 21702038, 21702054), Shenzhen Fundamental Research Projects (JCYJ20160525164227350, JCYJ20170811161344569) and the open project program of Hubei Key Laboratory of Drug Synthesis and Optimization, Jingchu University of Technology (OPP2016ZD01) for financial support.
Notes and references
-
(a) J. Cheng, K. Kamiya and I. Kodama, Cardiovasc. Drug Rev., 2001, 19, 152 CrossRef CAS PubMed;
(b) C. Sánchez, C. Méndez and J. A. Salas, Nat. Prod. Rep., 2006, 23, 1007 RSC;
(c) Amino Group Chemistry, From Synthesis to the Life Sciences, ed. A. Ricci, Wiley-VCH, Weinheim, 2007 Search PubMed;
(d) R. Hili and A. K. Yudin, Nat. Chem. Biol., 2006, 2, 284 CrossRef CAS PubMed;
(e) S. A. Lawrence, Amines: Synthesis Properties and Applications, Cambridge University Press, Cambridge, 2004, pp. 265–305 Search PubMed;
(f) Amino Group Chemistry, From Synthesis to the Life Sciences, ed. A. Ricci, Wiley-VCH, Weinheim, 2008 Search PubMed.
- For recent reviews:
(a) E. M. Beccalli, G. Broggini, M. Martinelli and S. Sottocornola, Chem. Rev., 2007, 107, 5318 CrossRef CAS PubMed;
(b) F. Collet, R. H. Dodd and P. Dauban, Chem. Commun., 2009, 5061 RSC;
(c) S. H. Cho, J. Y. Kim, J. Kwak and S. Chang, Chem. Soc. Rev., 2011, 40, 5068 RSC;
(d) M.-L. Louillat and F. W. Patureau, Chem. Soc. Rev., 2014, 43, 901 RSC;
(e) J.-P. Wan and Y. Jing, Beilstein J. Org. Chem., 2015, 11, 2209 CrossRef CAS PubMed;
(f) P. Subramanian, G. C. Rudolf and K. P. Kaliappan, Chem.–Asian J., 2016, 11, 168 CrossRef CAS PubMed;
(g) J. Jiao, K. Murakami and K. Itami, ACS Catal., 2016, 6, 610 CrossRef CAS;
(h) H. Kim and S. Chang, ACS Catal., 2016, 6, 2341 CrossRef CAS;
(i) R. K. Rit, M. Shankara and A. K. Sahoo, Org. Biomol. Chem., 2017, 15, 1282 RSC;
(j) Y. Park, Y. Kim and S. Chang, Chem. Rev., 2017, 117, 9247 CrossRef CAS PubMed.
-
(a) J. Colomb, G. Becker, S. Fieux, L. Zimmer and T. Billard, J. Med. Chem., 2014, 57, 3884 CrossRef CAS PubMed;
(b) S. Vandekerckhove, H. G. Tran, T. Desmet and M. D'hooghe, Bioorg. Med. Chem. Lett., 2013, 23, 4641 CrossRef CAS PubMed;
(c) K. Kaur, M. Jain, R. P. Reddy and R. Jain, Eur. J. Med. Chem., 2010, 45, 3245 CrossRef CAS PubMed;
(d) V. R. Solomon and H. Lee, Curr. Med. Chem., 2011, 18, 1488 CrossRef CAS PubMed;
(e) J. P. Michael, Nat. Prod. Rep., 2008, 25, 166 RSC;
(f) T. Eicher, S. Hauptmann and A. Speicher, The Chemistry of Heterocycles: Structures, Reactions, Synthesis, and Applications, John Wiley & Sons, New York, 3rd edn, 2013 Search PubMed;
(g) A. R. Katritzky, C. A. Ramsden, E. F. V. Scriven and R. J. K. Taylor, Comprehensive Heterocyclic Chemistry III, Elsevier, Oxford, 2008 Search PubMed.
-
(a) J. Roger, A. L. Gottumukkala and H. Doucet, ChemCatChem, 2010, 2, 20 CrossRef CAS;
(b) T. Iwai and M. Sawamura, ACS Catal., 2015, 5, 5031 CrossRef CAS;
(c) D. E. Stephens and O. V. Larionov, Tetrahedron, 2015, 71, 8683 CrossRef CAS PubMed.
-
(a) L. Zhu, X. Cao, Y. Li, T. Liu, X. Wang, R. Qiu and S.-F. Yin, Chin. J. Inorg. Chem., 2017, 37, 1613 CrossRef CAS;
(b) B. Khan, H. S. Dutta and D. Koley, Asian J. Org. Chem., 2018, 7, 1270 CrossRef CAS.
-
(a) E. Pan, N. W. Oswald, A. G. Legako, J. M. Life, B. A. Posner and J. B. MacMillan, Chem. Sci., 2013, 4, 482 RSC;
(b) Y. Takayama, T. Yamada, S. Tatekabe and K. Nagasawa, Chem. Commun., 2013, 49, 6519 RSC;
(c) C. C. Hughes, J. B. MacMillan, S. P. Gaudêncio, P. R. Jensen and W. Fenical, Angew. Chem., Int. Ed., 2009, 48, 725 CrossRef CAS PubMed;
(d) J. Wienser, R. Ortmann, H. Jomaa and M. Schlitzer, Angew. Chem., Int. Ed., 2003, 42, 5274 CrossRef PubMed;
(e) V. K. Dua, S. N. Sinha, S. Biswas, N. Valecha, S. K. Puri and V. P. Sharma, Bioorg. Med. Chem. Lett., 2002, 12, 3587 CrossRef CAS PubMed.
-
(a) X. Zhu, P. Zhang, M. J. Ajitha, K.-W. Huang, Z. An and X. Liu, Chem.–Asian J., 2016, 11, 882 CrossRef PubMed;
(b) Y. Yin, J. Xie, F.-Q. Huang, L.-W. Qi and B. Zhang, Adv. Synth. Catal., 2017, 359, 1037 CrossRef CAS;
(c) H. Yi, H. Chen, C. Bian, Z. Tang, A. K. Singh, X. Qi, X. Yue, Y. Lan, J.-F. Lee and A. Lei, Chem. Commun., 2017, 53, 6736 RSC.
-
(a) D. Ji, X. He, Y. Xu, Z. Xu, Y. Bian, W. Liu, Q. Zhu and Y. Xu, Org. Lett., 2016, 18, 4478 CrossRef CAS PubMed;
(b) Y. Wang, Y. Wang, Z. Guo, Q. Zhang and D. Li, Asian J. Org. Chem., 2016, 5, 1438 CrossRef CAS.
-
(a) T. B. Poulsen, C. Alemparte and K. A. Jøgensen, J. Am. Chem. Soc., 2005, 127, 11614 CrossRef CAS PubMed;
(b) J. T. Suri, D. D. Steiner and C. F. Barbas, Org. Lett., 2005, 7, 3885 CrossRef CAS PubMed;
(c) X. Yang and F. D. Toste, J. Am. Chem. Soc., 2015, 137, 3205 CrossRef CAS PubMed;
(d) S.-G. Wang, Q. Yin, C.-X. Zhuo and S.-L. You, Angew. Chem., Int. Ed., 2015, 54, 647 CAS;
(e) H.-Y. Bai, Z.-G. Ma, M. Yi, J.-B. Lin and S.-Y. Zhang, ACS Catal., 2017, 7, 2042 CrossRef CAS;
(f) H. Zhu, S. Sun, H. Qiao, F. Yang, J. Kang, Y. Wu and Y. Wu, Org. Lett., 2018, 20, 620 CrossRef CAS PubMed.
- H. Sahoo, M. K. Reddy, I. Ramakrishna and M. Baidya, Chem.–Eur. J., 2016, 22, 1592 CrossRef CAS PubMed.
-
(a) Y. Wang, Y. Wang, K. Jiang, Q. Zhang and D. Li, Org. Biomol. Chem., 2016, 14, 10180 RSC;
(b) Y. Wang, Y. Wang, Q. Zhang and D. Li, Org. Chem. Front., 2017, 4, 514 RSC.
-
(a) G. Rouquet and N. Chatani, Angew. Chem., Int. Ed., 2013, 52, 11726 CrossRef CAS PubMed;
(b) Y. Aihara, J. Wuelbern and N. Chatani, Bull. Chem. Soc. Jpn., 2015, 88, 438 CrossRef CAS;
(c) L. C. M. Castro and N. Chatani, Chem. Lett., 2015, 44, 410 CrossRef CAS;
(d) N. Chatani, Top. Organomet. Chem., 2015, 56, 19 CrossRef;
(e) X. Cai and B. Xie, ARKIVOC, 2015, 184 Search PubMed;
(f) J. Yamaguchi, K. Muto and K. Itami, Top. Curr. Chem., 2016, 374, 55 CrossRef PubMed;
(g) G. Pototschnig, N. Maulide and M. Schnerch, Chem.–Eur. J., 2017, 23, 9206 CrossRef CAS PubMed.
- For only a few examples:
(a) B. Khan, R. Kant and D. Koley, Adv. Synth. Catal., 2016, 358, 2352 CrossRef CAS;
(b) H. Chen, P. Li, M. Wang and L. Wang, Org. Lett., 2016, 18, 4794 CrossRef CAS PubMed;
(c) J. Ding, Y. Zhang and J. Li, Org. Chem. Front., 2017, 4, 1528 RSC.
- Only one silver-mediated C5 C–H functionalization of 8-aminoquinolines existed: M. Sun, S. Sun, H. Qiao, F. Yang, Y. Zhu, J. Kang, Y. Wu and Y. Wu, Org. Chem. Front., 2016, 3, 1646 RSC.
- For reviews on bimetallic cooperative catalysis:
(a) A. E. Allen and D. W. C. MacMillan, Chem. Sci., 2012, 3, 633 RSC;
(b) M. M. Lorion, K. Maindan, A. R. Kapdi and L. Ackermann, Chem. Soc. Rev., 2017, 46, 7399 RSC;
(c) D. R. Pye and N. P. Mankad, Chem. Sci., 2017, 8, 1705 RSC.
- CCDC-1859349†.
-
(a) P. G. Jessop, Green Chem., 2011, 13, 1391 RSC;
(b) D. Prat, A. Wells, J. Hayler, H. Sneddon, C. R. McElroy, S. Abou-Shehada and P. J. Dunn, Green Chem., 2016, 18, 288 RSC.
Footnote |
† Electronic supplementary information (ESI) available: For detailed experimental procedures and analytical data. CCDC 1859349 for 3aa. For ESI and crystallographic data in CIF or other electronic format see DOI: 10.1039/c8ra07647b |
|
This journal is © The Royal Society of Chemistry 2018 |