DOI:
10.1039/C8RA07619G
(Paper)
RSC Adv., 2018,
8, 39593-39601
Design, synthesis and fungicidal activity of isothiazole–thiazole derivatives†
Received
13th September 2018
, Accepted 8th November 2018
First published on 26th November 2018
Abstract
3,4-Dichloroisothiazoles can induce systemic acquired resistance (SAR) to enhance plant resistance against a subsequent pathogen attack, and oxathiapiprolin exhibits excellent anti-fungal activity against oomycetes targeting at the oxysterol-binding protein. To discover novel chemicals with systemic acquired resistance and fungicidal activity, 21 novel isothiazole–thiazole derivatives were designed, synthesized and characterized according to the active compound derivatization method. Compound 6u, with EC50 values of 0.046 mg L−1 and 0.20 mg L−1 against Pseudoperonospora cubensis (Berk. et Curt.) Rostov and Phytophthora infestans in vivo, might act at the same target as oxysterol binding protein (PcORP1) of oxathiapiprolin; this result was validated by cross-resistance and molecular docking studies. The expression of the systemic acquired resistance gene pr1 was significantly up-regulated after treating with compound 6u for 24 h (43-fold) and 48 h (122-fold). These results can help the development of isothiazole–thiazole-based novel fungicides.
1 Introduction
Crops are often infected by various pathogens, which results in yield reduction. Oomycetes are filamentous eukaryotic microorganisms1 that attack a large number of plants2 and animals3 and therefore, they can sometimes threaten agricultural ecosystems. Some pathogens cause humanitarian disasters; for example, the Great Famine was caused by oomycetes attacking potato fields.4 The oomycetes classified as Phytophthora are one of the most serious pathogenic threats all over the world.5 Only Phytophthora infestans (Mont.) de Bary caused the failure of the potato crop protection in 1840s and decreased the population of Ireland nearly by 25%.6
Heterocyclic compounds are widely used in novel agrochemical researches and developments.7–10 Thiazoles have attracted the interest of pharmaceutical and agrochemical anti-fungal research since the development of thiabendazole as a fungicide in 1962 by Merck.11 Oxathiapiprolin was developed by DuPont in 2007 as a piperidinyl thiazole isoxazoline fungicide targeting at oxysterol binding protein (PcORP1) against Peronospora belbahrii, Phytophthora parasitica var. Nicotianae (Breda de Hean) Tucker, Phytophthora capsici Leonian and downy mildew.12–16 However, fungicides acting at a single site of action have a high level of resistance risk.12
As a derivative of 1,3-thiazole, isotianil has a wide range of biological activities17–19 including fungicidal, insecticidal, herbicidal and antiviral activities via activating the plant induced systemic resistance and affecting multiple links in the life cycle of pathogens. Particularly, 3,4-dichloroisothiazoles not only have antifungal activity, but also show good systemic acquired resistance.17 Novel fungicide development is one of the important directions and measures for fungicide resistance management.20
On the basis of our former findings,4,5,21–23 to continue our aim of finding novel highly anti-fungal active compounds with novel modes of action and without resistance risks, 4 types of novel target molecules were designed and synthesized for fungicidal activity and systemic acquired resistance evaluation based on PcORP1 as the target and N and S containing five-membered heterocycles as bioactive substructures by the combination of bioactive substructures of plant elicitor isotianil and fungicide oxathiapiprolin according to the active compound derivatization method24 (Fig. 1 and Table 1). The mode of action of the active compound was validated by cross resistance, molecular biology and molecular docking studies.
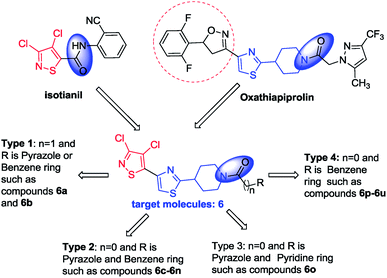 |
| Fig. 1 Design of target isothiazole–thiazoles. | |
Table 1 The structures of the target compounds 6a–6u
Compound |
n |
R |
R1 |
Compound |
n |
R |
R1 |
6a |
1 |
 |
— |
6k |
0 |
 |
 |
6b |
1 |
 |
— |
6l |
 |
6c |
0 |
 |
 |
6m |
 |
6d |
 |
6n |
 |
6e |
 |
6o |
0 |
 |
— |
6f |
 |
6p |
0 |
 |
— |
6g |
0 |
 |
 |
6q |
0 |
 |
— |
6h |
 |
6r |
0 |
 |
— |
6i |
 |
6s |
0 |
 |
— |
6j |
 |
6t |
0 |
 |
— |
|
|
|
|
6u |
0 |
 |
— |
2 Results and discussion
2.1 Chemistry
The synthesis route of isothiazole–thiazole derivatives is designed and shown in Scheme 1. Compound 1 was constructed according to reported procedures.17 3,4-Dichloro-N-methoxy-N-methylisothiazole-5-carboxamide was obtained by the reaction between 3,4-dichloroisothiazole-5-carboxylic acid and N,O-dimethylhydroxylamine hydrochloride to produce the Weinreb amide, which was then reacted with methyl magnesium bromide to obtain 1-(3,4-dichloroisothiazol-5-yl)ethan-1-one 1 with a good yield. Compound 2 could be prepared in one step from compound 1 by a substitution reaction with pyridinium tribromide. Compound 4 was synthesized by condensation between intermediate 2 and tert-butyl 4-carbamothioylpiperidine-1-carboxylate 3. After removal of protection group at N-Boc of compound 4under trifluoroacetic acid, intermediate 5 was obtained. By the activation of the corresponding acid using N-(3-dimethylaminopropyl)-N′-ethylcarbodiimide hydrochloride and 1-hydroxybenzotriazole and the succeeding condensation with compound 5, isothiazole–thiazole derivatives 6 were effectively afforded. A crystal of the representative compound 6j was obtained from the mixture of dichloromethane and ethyl acetate for X-ray diffraction (Fig. 2).
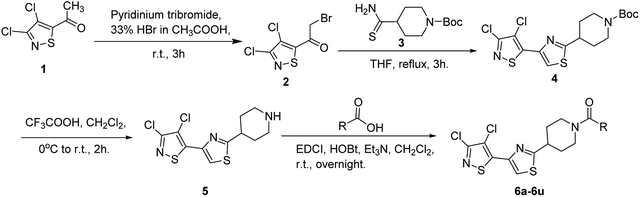 |
| Scheme 1 Synthesis route of isothiazole–thiazole derivatives. | |
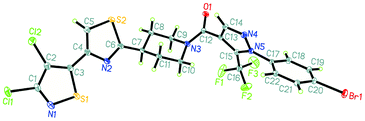 |
| Fig. 2 X-ray single crystal structure of compound 6j. | |
2.2 Compound 6u has the same potent target as oxathiapiprolin
2.2.1 Fungicidal spectrum in vitro. The median effective concentration (EC50) values of compounds 6a–6u with inhibition rates greater than 70% at 50 mg L−1 are listed in Table 2.
Table 2 Toxicities (EC50 values) of the target compounds in vitro
Fungi |
Compd |
Regression equation |
R2 |
EC50/mg L−1 |
Azoxystrobin, the data cited from the ref. 21. Azoxystrobin, the data cited from the ref. 22. |
A. solani |
6o |
y = 4.2714 + 0.7903x |
0.9642 |
8.92 |
6s |
y = 4.3901 + 0.6928x |
0.9839 |
7.84 |
Oxathiapiprolin |
y = 2.3920 + 1.0678x |
0.9857 |
296.60 |
Azoxystrobina |
y = 3.7648 + 0.5446x |
0.9251 |
185.42 |
G. zeae |
6a |
y = 3.5238 + 1.2423x |
0.9917 |
15.03 |
6f |
y = 4.2432 + 0.8867x |
0.9828 |
7.67 |
6o |
y = 3.8997 + 0.9485x |
0.9858 |
15.60 |
Oxathiapiprolin |
y = 4.8606 + 0.8936x |
0.9944 |
1.47 |
Azoxystrobinb |
y = 4.3881 + 0.7278x |
0.9777 |
6.92 |
S. sclerotiorum |
6b |
y = 6.8128 + 2.7830x |
0.9298 |
0.22 |
6c |
y = 5.1267 + 0.4377x |
0.9284 |
0.53 |
6l |
y = 4.5982 + 0.7369x |
0.9611 |
3.83 |
6r |
y = 3.1831 + 1.4723x |
0.9582 |
17.36 |
Oxathiapiprolin |
y = 3.9644 + 1.3662x |
0.9855 |
5.98 |
Azoxystrobina |
y = 4.6795 + 0.5283x |
0.8375 |
4.04 |
Both compounds 6o and 6s showed higher activities, with EC50 values of 8.92 mg L−1 and 7.84 mg L−1, respectively, than oxathiapiprolin (EC50 = 296.60 mg L−1) and azoxystrobin (EC50 = 185.42 mg L−1) against Alternaria solani. The EC50 values of compounds 6b (EC50 = 0.22 mg L−1) and 6c (EC50 = 0.53 mg L−1) were about 1/20 and 1/10, respectively, as compared to that of commercial fungicides oxathiapiprolin (EC50 = 5.98 mg L−1) and azoxystrobin (EC50 = 4.04 mg L−1) against Sclerotinia sclerotiorum.
2.2.2 Result of cross-resistance. The cross-resistance between oxathiapiprolin and 6u against P. capsici was detected. As shown in Table 3, the mutants LP3-m and LP3-h exhibited significant resistance to 6u (the inhibition rates were 12% and 7%, respectively, whereas the inhibition rate of LP3 was 32% at 10 mg L−1). These results indicated that the oxysterol binding protein PcORP1, i.e., the target site of oxathiapiprolin was the potent target of 6u. These tentative results were further validated by docking studies.
Table 3 Oomycete sensitivity in wild-type and oxathiapiprolin-resistant strains of P. capsici
Strains |
Inhibition ratio (mean ± SD) |
6u (10 mg L−1) |
Oxathiapiprolin (10 mg L−1) |
Oxathiapiprolin (1 mg L−1) |
LP3 (wild-type) |
32 ± 3% |
100% |
100% |
LP3-m (oxathiapiprolin-resistant) |
12 ± 2% |
78 ± 2% |
26 ± 3% |
LP3-h (oxathiapiprolin-resistant) |
7 ± 2% |
71 ± 5% |
17 ± 5% |
2.2.3 Docking analysis. Molecular docking is the commonly used method to validate the potential target of pesticides. To elucidate the possible binding modes and affinities between the newly designed inhibitors and the potent target PcORP1, detailed interactions of oxathiapiprolin and compound 6u were docked into the active center of PsORP1 (Fig. 3). The conformation of PsORP1 in the complex with oxathiapiprolin was used as the control for molecular docking, and the docked complexes were shown by Pymol and LigPlot, which displayed potential bonding interactions for a hypothetical binding mode of oxathiapiprolin and PcORP1. The F atoms in trifluoromethyl of oxathiapiprolin could form a hydrogen bond with a NH of adjacent Asn765 in very excellent interaction. The thiazole ring of 6u could interact with Trp762 through π–π interaction. In the docked complexes between 6u and PsORP1, no hydrogen bond appeared. This might be the cause of poor activity of 6u towards PsORP1. However, π–π interaction between the piperidine ring of 6u and Trp762 appeared to be possible.
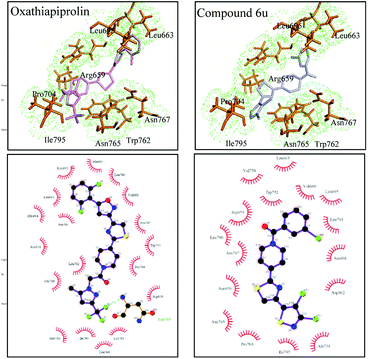 |
| Fig. 3 Molecular docking comparison results of oxathiapiprolin and compound 6u with PsORP1. | |
2.3 Results of compounds 6a–6u against oomycetes in vivo
The fungicidal activities of 6a–6u against oomycetes of P. cubensis and P. infestans at 100 mg L−1 in vivo are listed in Table 4. Most of the designed isothiazole–thiazole derivatives displayed excellent in vivo anti-oomycete activity (100%) against P. cubensis and P. infestans at 100 mg L−1, and the EC50 values of the compounds with inhibition over 90% at 100 mg L−1 against P. cubensis (Table 5) and P. infestans (Table 6) in vivo were determined.
Table 4 Fungicidal activity of piperidinyl-thiazoles at 100 mg L−1 in vivo
Compd |
Inhibition rate (%) |
P. cubensis |
P. infestans |
6a |
0 |
0 |
6b |
100 |
100 |
6c |
100 |
100 |
6d |
100 |
100 |
6e |
100 |
100 |
6f |
30 ± 2 |
0 |
6g |
0 |
0 |
6h |
100 |
0 |
6i |
40 ± 1 |
100 |
6j |
0 |
0 |
6k |
100 |
100 |
6l |
100 |
100 |
6m |
100 |
100 |
6n |
100 |
100 |
6o |
100 |
60 ± 4 |
6p |
100 |
100 |
6q |
100 |
0 |
6r |
100 |
100 |
6s |
100 |
100 |
6t |
80 ± 4 |
100 |
6u |
100 |
100 |
Isotianil |
100 |
100 |
Oxathiapiprolin |
100 |
100 |
Table 5 The EC50 values of 6a–6u against P. cubensis in vivo
Compd |
Regression equation |
R2 |
EC50 (mg L−1) |
95% CIa of EC50 |
95% confidence interval. Not determined. |
6a |
—b |
— |
>100 |
— |
6b |
y = 5.7565 + 0.7424x |
0.9808 |
0.10 |
0.01–0.70 |
6c |
y = 2.9999 + 3.1494x |
0.9330 |
4.61 |
0.97–22.46 |
6d |
y = 2.9744 + 3.1505x |
0.9273 |
4.71 |
0.91–23.79 |
6e |
y = 0.4131 + 4.9556x |
0.9901 |
12.49 |
7.59–22.36 |
6f |
— |
— |
>100 |
— |
6g |
— |
— |
>100 |
— |
6h |
y = 0.4131 + 4.9556x |
0.9901 |
12.49 |
7.59–22.36 |
6i |
— |
— |
>100 |
— |
6j |
— |
— |
>100 |
— |
6k |
y = 4.7655 + 1.5431x |
0.9665 |
1.42 |
0.35–6.39 |
6l |
y = 4.7479 + 0.8326x |
1.0000 |
2.02 |
1.99–2.18 |
6m |
y = 5.1520 + 2.2378x |
0.9609 |
0.91 |
0.16–4.94 |
6n |
y = 4.9881 + 1.0722x |
0.9998 |
1.05 |
0.93–1.24 |
6o |
y = 0.4131 + 4.9556x |
0.9901 |
12.49 |
7.59–22.36 |
6p |
y = 7.2490 + 2.3175x |
0.9788 |
0.11 |
0.05–0.24 |
6q |
y = 0.4710 + 4.9817x |
0.9858 |
13.58 |
7.23–24.05 |
6r |
y = 3.4658 + 2.9562x |
0.9253 |
3.49 |
0.62–19.46 |
6s |
y = 4.1499 + 2.9208x |
1.0000 |
2.13 |
2.06–2.11 |
6t |
— |
— |
— |
— |
6u |
y = 9.1621 + 3.0757x |
0.9621 |
0.046 |
0.016–0.13 |
Oxathiapiprolin |
y = 8.7940 + 1.6011x |
0.9997 |
0.0046 |
0.0042–0.0051 |
Isotianil |
y = 5.1578 + 5.0000x |
0.9985 |
1.01 |
0.78–1.22 |
Table 6 The EC50 values of 6a–6u against P. infestans in vivo
Compd |
Regression equation |
R2 |
EC50 (mg L−1) |
95% CIa of EC50 |
95% confidence interval. Not determined. |
6a |
—b |
— |
>100 |
— |
6b |
y = 5.3634 + 1.9964x |
0.9634 |
0.68 |
0.21–2.10 |
6c |
y = 3.0649 + 3.1028x |
0.9704 |
4.44 |
1.73–11.03 |
6d |
y = 0.2101 + 4.8366x |
0.9889 |
13.00 |
7.16–22.02 |
6e |
y = 0.2101 + 4.8366x |
0.9889 |
13.00 |
7.16–22.02 |
6f |
— |
— |
>100 |
— |
6g |
— |
— |
>100 |
— |
6h |
— |
— |
>100 |
— |
6i |
y = 3.0513 + 3.1078x |
0.9662 |
4.39 |
1.67–11.19 |
6j |
— |
— |
>100 |
— |
6k |
y = 4.7053 + 1.0010x |
0.9969 |
2.14 |
1.61–2.86 |
6l |
y = 3.2938 + 3.0991x |
0.9770 |
3.66 |
1.76–8.40 |
6m |
y = 5.1537 + 1.0510x |
0.9921 |
0.74 |
0.48–1.25 |
6n |
y = 5.2728 + 1.0510x |
0.9352 |
0.75 |
0.17–3.47 |
6o |
— |
— |
>100 |
— |
6p |
y = 6.3442 + 2.9208x |
0.9167 |
0.38 |
0.06–2.31 |
6q |
— |
— |
>100 |
— |
6r |
y = 3.0513 + 3.1078x |
0.9662 |
4.39 |
1.67–11.19 |
6s |
y = 5.1960 + 1.7904x |
0.9555 |
0.81 |
0.26–2.67 |
6t |
y = 4.7813 + 2.0405x |
0.9050 |
1.32 |
0.22–7.80 |
6u |
y = 6.8798 + 2.6878x |
0.9651 |
0.20 |
0.06–0.75 |
Oxathiapiprolin |
— |
— |
<0.10 |
— |
Isotianil |
y = 5.4639 + 2.0486x |
0.9749 |
0.61 |
0.23–1.71 |
All compounds except 6a, 6f, 6g, 6i, 6j, 6o and 6q exhibited good to excellent inhibitory activity against P. cubensis and P. infestans with EC50 values of 0.046–12.49 mg L−1 and 0.20–13.00 mg L−1, respectively. For P. cubensis, compounds 6k, 6l, 6n and 6s displayed the same level of fungicidal activity as that of isotianil (EC50 = 1.01 mg L−1) with EC50 from 1.05 mg L−1 to 2.13 mg L−1, whereas compounds 6b, 6m, 6p and 6u with EC50 of less than 1.00 mg L−1 exhibited higher activity than the positive control of isotianil against P. cubensis. The anti-oomycete activity of compound 6u (EC50 = 0.046 mg L−1) was 10 times less effective than that of the positive control oxathiapiprolin (EC50 = 0.0046 mg L−1) against P. cubensis; however, it was 20 times better than that of the positive control isotianil (EC50 = 1.01 mg L−1). It is worth noting that compounds 6b, 6m, 6p and 6u also displayed excellent inhibitory activities against P. infestans with EC50 values less than 1.00 mg L−1. Moreover, EC50 of compound 6n was 0.75 mg L−1. Ultimately, compound 6u exhibited the best fungicidal activity against P. infestans with EC50 of 0.20 mg L−1 among all the isothiazole–thiazoles designed and synthesized in this study.
2.4 Compound 6u can activate SAR in plants
2.4.1 Defense gene expression was induced in the SA pathway. Systemic acquired resistance (SAR) is a predominant form of inducing disease resistance in plant defence systems. SAR promotes plant protection against pathogens through induced salicylic acid (SA) biosynthesis, activated SA signalling pathway and enhanced expression of pathogenesis-related proteins (PRs).25 In particular, the expressed pr1 is a marker gene for SAR activation26 and NPR1 is an SA receptor that leads to pr1 activation.27“*” and “**” indicate significant difference between treated and mock at P < 0.05 and P < 0.01, respectively.
The expressions of npr1 and pr1 were analyzed in A. thaliana. The pr1 expression was significantly up-regulated by 64-fold and 143-fold when treated with 6u for 24 hours and 48 hours, respectively (Fig. 4). The npr1 expression was also significantly up-regulated by 4.7-fold after 48 hours of treatment, but the change was not very significant when treated for 24 hours. Isotianil, discovered by Bayer CropScience AG in 1997 and developed jointly with Sumitomo Chemical Co., Ltd, is used as a crop protectant against rice blast and rice leaf blight. The difference in gene expressions identified after isotianil treatment for 24 hours and 48 hours in Oryza sativa by microarray analysis showed that 17 PR genes including pr1a, pbz, npr1 and PR involved in antifungal activities such as chitinase and β-glucanase were upregulated.28 Based on these results, it is possible that the activation of pr1 can induce SAR and increase the fungicidal activity of compound 6u in vivo.
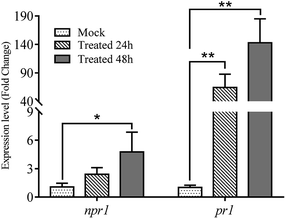 |
| Fig. 4 The expressions of pr1 and npr1 after treating with 6u. | |
3 Experimental
3.1 General information
All solvents were of analytical grade unless otherwise noted. 1-Substituted phenyl-5-trifluoromethyl-4-pyrazole carboxylic acid and substituted phenyl-5-difluoromethyl-4-pyrazole carboxylic acid were provided by Jia-xing Huang of China Agricultural University (Beijing, China). Melting points (temperature uncorrected) were recorded on an X-4 binocular microscope. 1H and 13C Nuclear Magnetic Resonance (NMR) spectra in CDCl3 or dimethyl sulfoxide-d6 were recorded on a Bruker AV400 spectrometer with tetramethylsilane as the internal standard. Mass spectrometry was conducted using Agilent 6520 Q-TOF LC/MS. The single crystal diffraction was carried out on a Rigaku 007HF XtaLAB P200 diffractometer. Fungicidal activities in vitro and in vivo were tested at Nankai University (Tianjin, China) and Shenyang Research Institute of Chemical Industry (Shenyang, China), respectively.
3.2 Synthesis
3.2.1 Synthesis and characterization of compound 2. To a solution of compound 1 (15.3 mmol) in 5 mL of 33% HBr and CH3COOH, pyridinium tribromide (18.8 mmol) was added. The mixture was stirred at room temperature for 3 h. After stopping the reaction, 50 mL of water was added into the reaction solution and the pH was adjusted to 4–5 with sodium bicarbonate. The aqueous layer was extracted using ethyl acetate (2 × 100 mL). The combined organic layers were washed with saturated brine (100 mL) and then dried over anhydrous Na2SO4. After removing the solvent via vacuum, the residue was purified by column chromatography on a silica gel using ethyl acetate and petroleum ether (60–90 °C) with υ/υ of 1
:
50 to obtain 2 as a white solid (15.3 mmol, 100%). Mp 52–53 °C; 1H NMR (400 MHz, CDCl3) δ 4.47 (d, J = 2.9 Hz, 2H, CH2). HRMS (ESI) [M − H]− calcd For C5H2BrCl2NOS (M − H)−: 271.8418, found: 271.8344
3.2.2 Synthesis and characterization of compound 4. A solution of tert-butyl 4-carbamothioylpiperidine-1-carboxylate 3 (8.25 mmol), which can be prepared from piperidine-4-carboxamide,29 in tetrahydrofuran (50 mL) was added to the intermediate 2 (9.07 mmol) while stirring and refluxing for 3 h. The solvent was evaporated, and the residue was dissolved using ethyl acetate (50 mL). The organic layer was washed with sodium hydroxide solution (1 mol L−1, 50 mL) and saturated brine (50 mL) successively and then dried over anhydrous sodium sulfate. After the solvent was removed under reduced pressure, the residue was purified by column chromatography on a silica gel using ethyl acetate and petroleum ether (60–90 °C) with υ/υ of 1
:
16 to obtain 4 as a white solid (3.48 mmol, 42%). Mp 153–155 °C; 1H NMR (400 MHz, CDCl3) δ 8.04 (s, 1H, thiazole-CH), 4.21 (t, J = 10.7 Hz, 2H, piperidine-CH2), 3.19 (m, 1H, piperidine-CH), 2.93 (t, J = 12.1 Hz, 2H, piperidine-CH2), 2.13 (m, 2H, piperidine-CH2), 1.77 (m, 2H, piperidine-CH2), 1.46 (s, 9H, CH3). HRMS (ESI) (M + H)+ calcd for C16H19Cl2N3O2S2: 420.0296, found: 420.0345; HRMS (ESI) (M + Na)+ calcd for C16H19Cl2N3O2S2: 442.0296, found: 442.0183.
3.2.3 Synthesis and characterization of compound 5. To an ice-cooled solution of 4 (3.48 mmol) in dry dichloromethane (30 mL), trifluoroacetic acid (87 mmol) was added, and the reaction mixture was stirred at room temperature for 2 h. After completion of the conversion, dichloromethane (50 mL) was added again to the mixture, and the pH was adjusted to 8 with sodium hydroxide solution (1 mol L−1). Then, the organic layer was extracted and washed using saturated brine (50 mL). After drying the organic layer over anhydrous sodium sulfate, the solvent dichloromethane (50 mL) was added again and the pH was adjusted to 8 with sodium hydroxide solution (1 mol L−1). Then, the organic layer was extracted and washed using saturated brine (50 mL). After the organic layer was dried over anhydrous sodium sulfate, the solvent was removed under reduced pressure and the residue was purified by column chromatography on a silica gel using dichloromethane, methanol and triethylamine with υ/υ/υ of 6
:
1
:
0.001 to obtain 5 as a white solid (3.19 mmol, 92%). Mp 97–99 °C; 1H NMR (400 MHz, CDCl3) δ 8.04 (s, 1H, thiazole-CH), 5.31 (s, 1H, NH), 3.22 (t, J = 11.6 Hz, 2H, piperidine-CH2), 3.17 (s, 1H, piperidine-CH), 2.78 (t, J = 11.7 Hz, 2H, piperidine-CH2), 2.14 (m, 2H, piperidine-CH2), 1.76 (m, 2H, piperidine-CH2). HRMS (ESI) [M + H]+ calcd for C11H11Cl2N3S2: 319.9771, found: 319.9850.
3.2.4 General procedure for the preparation of title compounds. The solution of 2-(5-methyl-3-(trifluoromethyl)-1H-pyrazol-1-yl)acetic acid (0.395 mmol), N-(3-dimethylaminopropyl)-N′-ethylcarbodiimide hydrochloride (0.451 mmol) and 1-hydroxybenzotriazole (0.395 mmol) in dry CH2Cl2 (10 mL) was cooled to 0 °C, and the mixture was stirred for 1 h under nitrogen atmosphere. Then, the mixture of compound 5 (0.376 mmol) in dry CH2Cl2 (10 mL) and Et3N (0.451 mmol) were added. The reaction mixture was stirred overnight at room temperature. After stopping the reaction, the mixture was washed with saturated NaHCO3 (20 mL) and brine (20 mL). The organic layer was dried (sodium sulfate anhydrous) and concentrated, and the residue was purified by column chromatography on a silica gel using ethyl acetate and petroleum ether (60–90 °C) with υ/υ of 1
:
9–1
:
3 to obtain target compound 6a.Compounds 6b to 6u were synthesized according to a similar procedure as that used for 6a by changing the raw materials, as shown in Scheme 1.
Data for 6a. White solid, mp > 200 °C, yield 60%, 1H NMR (400 MHz, DMSO) δ 8.08 (s, 1H, thiazole-CH), 6.35 (s, 1H, pyrazole-CH), 5.01 (s, 2H, CH2), 4.58 (d, J = 13.3 Hz, 1H, piperidine-CH2), 4.08 (d, J = 12.5 Hz, 1H, piperidine-CH2), 3.33 (s, 2H, piperidine-CH2), 2.93 (m, 1H, piperidine-CH), 2.34 (s, 3H, CH3), 2.22 (m, 2H, piperidine-CH2), 1.91–1.68 (m, 2H, piperidine-CH2). HRMS (ESI) [M + H]+ calcd for C18H16Cl2F3N5OS2: 510.0125, found: 510.0203. Anal. calcd for C18H16Cl2F3N5OS2: C, 42.36; H, 3.16; N, 13.72. Found: C, 42.15; H, 3.69; N, 13.74.
Data for 6c. White solid, mp 125–127 °C, yield 95%, 1H NMR (400 MHz, CDCl3) δ 8.10 (s, 1H, thiazole-CH), 7.78 (s, 1H, pyrazole-CH), 7.54 (s, 5H, Ph-H), 6.93 (t, J = 53.0 Hz, 1H, CHF2), 4.76 (s, 1H, piperidine-CH2), 4.24 (d, J = 64.7 Hz, 1H, piperidine-CH2), 3.38 (s, 2H, piperidine-CH2), 3.14 (s, 1H, piperidine-CH), 2.27 (m, 2H, piperidine-CH2), 1.91 (m, 2H, piperidine-CH2). HRMS (ESI) [M + H]+ calcd for C22H17Cl2F2N5OS2: 540.0220, found: 540.0299. Anal. calcd for C22H17Cl2F2N5OS2: C, 48.89; H, 3.17; N, 12.96. Found: C, 48.23; H, 3.30; N, 13.08.
Data for 6g. White solid, mp 181–183 °C, yield 92%, 1H NMR (400 MHz, CDCl3) δ 8.09 (s, 1H, thiazole-CH), 7.76 (s, 1H, pyrazole-CH), 7.50 (d, J = 8.2 Hz, 5H, Ph-H), 4.79 (d, J = 12.8 Hz, 1H, piperidine-CH2), 3.91 (d, J = 12.7 Hz, 1H, piperidine-CH2), 3.34 (t, J = 14.6 Hz, 2H, piperidine-CH2), 3.10 (m, 1H, piperidine-CH), 2.26 (m, 2H, piperidine-CH2), 1.87 (m, 2H, piperidine-CH2). HRMS (ESI) [M + H]+ calcd for C22H16Cl2F3N5OS2: 558.0125, found: 558.0190. Anal. calcd for C22H16Cl2F3N5OS2: C, 47.32; H, 2.89; N, 12.54. Found: C, 47.14; H, 3.41; N, 12.88.
Data for 6k. White solid, mp 167–169 °C, yield 90%, 1H NMR (400 MHz, CDCl3) δ 7.86 (d, J = 74.7 Hz, 1H, thiazole-CH, pyrazole-CH), 7.50–7.04 (m, 5H, Ph-H), 4.60 (s, 1H, piperidine-CH2), 3.59 (d, J = 64.5 Hz, 1H, piperidine-CH2), 3.12 (t, J = 64.9 Hz, 2H, piperidine-CH2), 2.99–2.75 (m, 1H, piperidine-CH), 2.12 (m, 2H, piperidine-CH2), 1.71 (m, 2H, piperidine-CH2). HRMS (ESI) [M + H]+ calcd for C22H16Cl2F3N5OS2: 591.9736, found: 591.9812. Anal. calcd for C22H16Cl2F3N5OS2: C, 44.57; H, 2.55; N, 11.81. Found: C, 44.42; H, 2.68; N, 12.28.
Data for 6p. White crystals, mp 163–164 °C, yield 100%, 1H NMR (400 MHz, CDCl3) δ 7.95 (s, 1H, thiazole-CH), 7.33 (s, 5H, Ph-H), 4.68 (s, 1H, piperidine-CH2), 3.81 (s, 1H, piperidine-CH2), 3.23 (m, 1H, piperidine-CH), 3.04 (t, J = 55.1 Hz, 2H, piperidine-CH2), 2.10 (m, 2H, piperidine-CH2), 1.74 (m, 2H, piperidine-CH2). HRMS (ESI) [M + H]+ calcd for C18H15Cl2N3OS2: 424.0034, found: 424.0109. Anal. calcd for C18H15Cl2N3OS2: C, 50.95; H, 3.56; N, 9.90. Found: C, 50.33; H, 3.36; N, 9.87.
3.3 In vitro antifungal activity test
The fungicidal activities of title compounds against Cercospora arachidicola (C. A), Alternaria solani (A. S), Botrytis cinereal (B. C), Gibberella zeae (G. Z), Physalospora piricola (P. P), Sclerotinia sclerotiorum (S. S), Rhizoctonia cerealis (R. C) and Pellicularia sasakii (P. S) were tested at 50 mg L−1 in vitro by the mycelium growth rate method according to our previous publications.30,31 Commercial fungicides oxathiapiprolin, isotianil and azoxystrobin were chosen as positive controls. The EC50 values of the compounds with fungi growth inhibition greater than 70% at 50 mg L−1 were measured.
3.4 Oomycete sensitivity in wild-type and oxathiapiprolin-resistant strains of P. capsici
The P. capsici strains of the oxathiapiprolin-resistant mutants LP3-m, LP3-h and wild-type LP3 (ref. 32) were used for the effectiveness tests of compound 6u and oxathiapiprolin by measuring their colony diameters after dark-incubation at 25 °C for 5 days. Each strain and concentration were measured in three plates and the experiments were performed for three times.
3.5 Molecular modelling studies and docking analysis of PsORP1
For molecular modelling, the structure of PsORP1 (ref. 32) (Protein ID: 558498) in Phytophthora capsici was generated using the homology model application within the YASARA program33 with default parameters. The binding center of PsORP1 was identified by aligning to the template structure in Autodock Tools.34,35 The coordinate of the binding center in PsORP1 was identified by the model structures of 4B2Z and 5H2D. The structures of the ligands were optimized with the ligand minimization protocol. The molecular docking analysis was performed by Autodock Tools and ten random conformations were generated for each ligand. The rest of the parameters were set to default values. The optimal structure of the complex was selected based on visual inspection and the docking score. The structures of the complexes were shown by Pymol36 and LigPlot.37
3.6 In vivo antifungal activity test
The protective activities in potted plants of the title compounds were determined by the procedures described below:31 compounds 6a–6u (10.0 mg) and positive controls oxathiapiprolin and isotianil were dissolved in 0.5 mL DMF solutions. Then, they were diluted using 95.5 mL of distilled water (containing 0.1% Tween 80) to obtain the working solutions of 100 mg L−1. The working solutions were sprayed on to the host plant using a sprayer when the plants were grown to 1 to 3 leaf stages. After 24 h, the leaves treated by working solution were inoculated by the fungi of P. cubensis and P. infestans; 7 days later, the inhibitory activities in vivo of all the compounds were assessed. Two concentrations of 10 mg L−1 and 1 mg L−1 were further tested for the compounds with activity over 90% against P. cubensis at 100 mg L−1. Then, the compounds with more than 90% inhibition against P. cubensis at 1 mg L−1 were investigated at 0.1 mg L−1, 0.01 mg L−1 and 0.001 mg L−1. The compounds with 100% inhibition rate against P. infestans were tested for 3 concentrations of 10 mg L−1, 1 mg L−1 and 0.1 mg L−1. An inhibition rate of 100% represents complete control of the fungi growth and an inhibition rate of 0% represents no control of the fungi growth.
3.7 RNA extraction and Q-PCR analysis of the expression of defence genes in the salicylic acid pathway
Based on the difference between anti-oomycete activities in vivo and in vitro, we proposed that compound 6u might be able to induce systemic acquired resistance of plants. The RNAs of Arabidopsis thaliana, which was treated with 6u (10 mg L−1) and DMF, were isolated by using E.Z.N.A.® Plant RNA Kit (Omega, USA) and the mRNA was transcribed into cDNA reversely. Q-PCR was performed using the 2−ΔΔCT method38 with TransStart Top Green Q-PCR Super Mix (K31102, TransStart, China). The expressions of defense genes pr1 and npr1 in the salicylic acid pathway were analyzed and the primers used for Q-PCR are shown in Table 7.
Table 7 Primers used for Q-PCR
Gene ID |
Gene name |
Primer-F |
Primer-R |
AT3G41768 |
18s |
tgtttgatggtaactactactc |
gaatgatgcctcgccagcacaga |
AT1G64280 |
npr1 |
acgaagagaacatcaccggg |
cgggaagaatcgtttcccga |
AT2G14610 |
pr1 |
attttactggctattctcgatt |
agttgcctcttagttgttctgcg |
4 Conclusions
A series of novel isothiazole–thiazole derivatives were designed, synthesized and rationally characterized. The novel compounds were screened for antifungal activity in vitro and anti-oomycete activity in vivo. Compound 6u exhibited excellent anti-oomycete activity in vivo with EC50 values of 0.046 mg L−1 and 0.27 mg L−1 against P. cubensis and P. infestans, respectively. Although 6u was not as effective as oxathiapiprolin, it was much better than isotianil, a compound which can induce plant systemic acquired resistance. Therefore, isothiazole–thiazole derivatives are worthy of further research. Since the docking experiments indicated that oxathiapiprolin can form a hydrogen bond with Asn 765 of PsORP1, analogues with appropriately placed N–H, O–H and F–H units in a target molecule will be designed for further improvement of anti-fungal activity of compound 6u.
Conflicts of interest
There are no conflicts to declare.
Acknowledgements
This work was supported by the National Natural Science Foundation of China (No. 31571991 and No. 31872007), the Tianjin Natural Science Foundation (No. 18JCZDJC33500), the China Postdoctoral Science Foundation (No. 2017M611156) and the International Science & Technology Cooperation Program of China (No. 2014DFR41030). The authors also thank Dr Jiaxing Huang for providing pyrazole compounds (synthesized in the Key Technologies R&D program of China, 2015BAK45B01, CAU) for model reaction. The authors are grateful to the research team of Professor Xi-Li Liu at CAU for their help of determination of the active compounds against oxathiapiprolin-resistant fungi. Kalinina T. A. thanks the Russian Foundation for Basic Research (Grant No. 18-316-20018).
Notes and references
- M. Larousse and E. Galiana, PLoS Pathog., 2017, 13, e1006028 CrossRef PubMed.
- S. Kamoun, O. Furzer, J. D. G. Jones, H. S. Judelson, G. S. Ali, R. J. D. Dalio, S. G. Roy, L. Schena, A. Zambounis and F. Panabières, Mol. Plant Pathol., 2015, 16, 413–434 CrossRef PubMed.
- P. V. West and G. W. Beakes, Fungal Biol., 2014, 118, 525–526 CrossRef PubMed.
- Y. J. Zhu, J. Q. Huo, Z. J. Fan, Q. F. Wu, X. Li, S. Zhou, L. X. Xiong, T. Kalinina and T. Glukhareva, Org. Biomol. Chem., 2018, 16, 1163–1166 RSC.
- Z. J. Fan, Z. K. Yang, H. K. Zhang, M. Na, H. Wang, C. Fei, X. Zuo, Q. X. Zheng and H. B. Song, J. Agric. Food Chem., 2010, 58, 2630–2636 CrossRef CAS PubMed.
- W. Fry, Mol. Plant Pathol., 2008, 9, 385–402 CrossRef PubMed.
- M. A. Castro, A. M. Gamito, V. Tangarife-Castano, V. Roa-Linares, M. D. C. J. Ma, A. C. Mesa-Arango, L. Betancur-Galvis, A. M. Francesch and A. S. Feliciano, RSC Adv., 2015, 46, 1244–1261 RSC.
- B. L. Wang, H. W. Zhu, Z. M. Li, L. Z. Wang, X. Zhang, L. X. Xiong and H. B. Song, Pest Manage. Sci., 2018, 74, 726–736 CrossRef CAS PubMed.
- S. K. Li, K. U. Huang, B. A. Cao, J. W. Zhang, W. J. Wu and X. M. Zhang, Angew. Chem., Int. Ed., 2012, 51, 8573–8576 CrossRef CAS PubMed.
- L. Zhang, W. Li, T. F. Xiao, Z. H. Song, R. Csuk and S. K. Li, J. Agric. Food Chem., 2018, 66, 8957–8965 CrossRef CAS PubMed.
- H. D. Brown, A. R. Matzuk, I. R. Ilves, L. H. Peterson, S. A. Harris, L. H. Sarett, J. R. Egerton, J. J. Yakstis, W. C. Campbell and A. C. Cuckler, J. Am. Chem. Soc., 1961, 83, 174–175 Search PubMed.
- R. J. Pasteris and M. A. Hanagan, US Pat., WO/2013/0261154, 2014.
- J. Q. Miao, X. Dong, D. Lin, Q. S. Wang, P. F. Liu, F. R. Chen, Y. X. Du and X. L. Liu, Pest Manage. Sci., 2016, 72, 1572–1577 CrossRef CAS PubMed.
- P. Ji, A. S. Csinos, L. L. Hickman and U. Hargett, Plant Dis., 2014, 98, 1551–1554 CrossRef CAS.
- P. Ji and A. S. Csinos, Ann. Appl. Biol., 2015, 166, 229–235 CrossRef CAS.
- Y. Cohen, PLoS One, 2015, 10, e0140015 CrossRef PubMed.
- L. Chen, X. F. Guo, Z. J. Fan, N. L. Zhang, Y. J. Zhu, Z. M. Zhang, I. Khazhieva, M. Y. Yurievich, N. P. Belskaya and V. A. Bakulev, RSC Adv., 2017, 7, 3145–3151 RSC.
- J. G. Samaritoni, J. M. Babcock, M. L. Schlenz and G. W. Johnson, J. Agric. Food Chem., 1999, 47, 3381–3388 CrossRef CAS PubMed.
- X. Y. Chen, L. Y. Dai, Y. D. Li, W. T. Mao, Z. Fang, J. J. Li, D. Wang, K. Tatiana and Z. J. Fan, Chin. J. Pestic. Sci., 2013, 15, 140–144 CAS.
- D. Worrall, G. H. Holroyd, J. P. Moore, M. Glowacz, P. Croft, J. E. Taylor, N. D. Paul and M. R. Roberts, New Phytol., 2012, 193, 770–778 CrossRef CAS PubMed.
- L. Chen, B. Zhao, Z. J. Fan, X. M. Liu, Q. F. Wu, H. P. Li and H. X. Wang, J. Agric. Food Chem., 2018, 66, 7319–7327 CrossRef CAS PubMed.
- L. Chen, Y. J. Zhu, Z. J. Fan, X. F. Guo, Z. M. Zhang, J. H. Xu, Y. Q. Song, Y. Y. Morzherin, N. P. Belskaya and V. A. Bakulev, J. Agric. Food Chem., 2017, 65, 745–751 CrossRef CAS PubMed.
- B. Zhao, S. J. Fan, Z. J. Fan, H. X. Wang, N. L. Zhang, X. F. Guo, D. Y. Yang, Q. F. Wu, B. Yu and S. Zhou, J. Agric. Food Chem., 2018, 66, 12439–12452 CrossRef CAS PubMed.
- A. Y. Guan, C. L. Liu, X. P. Yang and M. Dekeyser, Chem. Rev., 2014, 114, 7079–7107 CrossRef CAS PubMed.
- F. Sun, P. Y. Zhang, M. R. Guo, W. Q. Yu and K. S. Chen, Food Chem., 2013, 138, 539–546 CrossRef CAS PubMed.
- L. C. Van Loon, M. Rep and C. M. Pieterse, Annu. Rev. Phytopathol., 2006, 44, 135–162 CrossRef CAS PubMed.
- Y. Wu, D. Zhang, J. Y. Chu, P. Boyle, Y. Wang, I. D. Brindle, L. V. De and C. Després, Cell Rep., 2012, 1, 639–647 CrossRef CAS PubMed.
- V. Toquin, C. Sirven, L. Assmann and H. Sawada, in Modern Crop Protection Compounds, 2012, ed. W. Krämer, U. Schirmer, P. Jeschke and M. Witschel, Wiley-VCH, Weinheim, Germany, pp. 739–748 Search PubMed.
- H. Kim, S. J. Cho, M. Yoo, S. K. Kang, K. R. Kim, H. H. Lee, J. S. Song, S. D. Rhee, W. H. Jung and J. H. Ahn, Bioorg. Med. Chem. Lett., 2017, 27, 5213–5220 CrossRef CAS PubMed.
- Z. J. Fan, Z. G. Shi, H. K. Zhang, X. F. Liu, L. L. Bao, L. Ma, X. Zuo, Q. X. Zheng and N. Mi, J. Agric. Food Chem., 2009, 57, 4279–4286 CrossRef CAS PubMed.
- F. Y. Li, X. F. Guo, Z. J. Fan, Y. Q. Zhang, G. N. Zong, X. L. Qian, L. Y. Ma, L. Chen, Y. J. Zhu and K. Tatiana, Chin. Chem. Lett., 2015, 26, 1315–1318 CrossRef CAS.
- J. Q. Miao, C. Meng, D. Xue, L. Li, L. Dong, C. Zhang, Z. L. Pang and X. L. Liu, Front. Microbiol., 2016, 7, 615 Search PubMed.
- H. Land and M. S. Humble, Methods Mol. Biol., 2018, 1685, 43–67 CrossRef PubMed.
- E. D. Muzio, D. Toti and F. Polticelli, J. Comput.-Aided Mol. Des., 2017, 31, 1–6 CrossRef PubMed.
- G. M. Morris, D. S. Goodsell, R. Huey and A. J. Olson, J. Mol. Recognit., 1996, 10, 293–304 CAS.
- D. Seeliger and B. L. D. Groot, J. Comput.-Aided
Mol. Des., 2010, 24, 417–422 CrossRef CAS PubMed.
- A. C. Wallace, R. A. Laskowski and J. M. Thornton, Protein Eng., 1995, 8, 127–134 CrossRef CAS PubMed.
- K. J. Livak and T. D. Schmittgen, Methods, 2001, 25, 402–408 CrossRef CAS PubMed.
Footnotes |
† Electronic supplementary information (ESI) available: Crystal data of 6j (CCDC 1867013), antifungal activities of target compounds in vivo and vitro and the physico-chemical data of the title compound. CCDC 1867013. For ESI and crystallographic data in CIF or other electronic format see DOI: 10.1039/c8ra07619g |
‡ Qifan Wu and Bin Zhao contributed equally to this work. |
|
This journal is © The Royal Society of Chemistry 2018 |
Click here to see how this site uses Cookies. View our privacy policy here.