DOI:
10.1039/C8RA07361A
(Paper)
RSC Adv., 2018,
8, 40748-40759
Simultaneous optimization of ultrasonic-assisted extraction of antioxidant and anticoagulation activities of compounds from Leonurus japonicus Houtt. by response surface methodology†
Received
4th September 2018
, Accepted 16th November 2018
First published on 5th December 2018
Abstract
Leonurus japonicus Houtt. is a herbaceous annual of the Lamiaceae family with pantropical distribution; it is called motherwort in China. It plays important roles in anticoagulation and antioxidation. This study aimed to explore the optimization of ultrasound-assisted extraction of multiple compounds from motherwort as well as their antioxidant and anticoagulation activities using response surface methodology. Box–Behnken design was employed to optimize three significant influences, namely extraction time, extraction temperature and ethanol concentration. The optimum extraction parameters acquired based on a combination of the yield of the target compounds and their antioxidant and anticoagulation activities were an extraction time of 38.2 min, an extraction temperature of 30.0 °C, an ethanol concentration of 48.9% (v/v), a solid–liquid ratio of 30.0 mL g−1 and an ultrasonic power of 500.0 W. Under the optimal conditions, the maximal yield of the anticoagulation and antioxidant compounds of motherwort was 0.994%; the thrombin time was 19.872 s; prothrombin time was 8.270 s; the activated partial thromboplastin time was 15.535 s; the fibrinogen was 1.420 g L−1; and the 1,1-diphenyl-2-picrylhydrazyl free radical scavenging activity was 1.503 mg mL−1. The optimized conditions model showed a good correlation between the predicted and experimental values. According to the results of our study, the optimization extraction significantly enabled study of the anticoagulation and antioxidant activities of compounds in motherwort; this may contribute to future research on the pharmacological activities of motherwort.
1. Introduction
Leonurus japonicus Houtt. (LJ), a traditional herb with a long history of medicinal use, is found in several regions in Asia, including China, Korea and Japan. China is the main productive area of LJ.1 In China, LJ is an abundant resource, and the production of LJ is nearly hundreds of thousands of tons.2
In 1990, LJ was added to the Pharmacopoeia of the People's Republic of China. According to preliminary statistics, more than 300 prescriptions containing LJ have been adopted to treat many diseases, especially those interfering with blood coagulation, in China. Some well-known prescriptions are currently widely used, such as “Xin-Sheng-Hua Granule” and “Ba Zhen Yi Mu Wan”.1,3–5 Currently, due to its pharmacological anti-cancer, anti-inflammatory, antibacterial, antifungal and antioxidant effects, LJ is widely used in health care products and/or functional foods to improve the symptoms of blood coagulation and oxidative damage in some common chronic diseases, such as cancer, diabetes and cardiovascular diseases.
Modern pharmacological research has indicated that excessive oxidative stress and blood coagulation are associated with cancer, diabetes and cardiovascular diseases.6–8 Furthermore, smoking, radiation and environmental pollutants may cause excessive oxidative stress and blood coagulation in the human body. Therefore, anticoagulants and antioxidants can be regarded as effectual methods to prevent and decrease the negative effects caused by blood coagulation and oxidative stress, respectively.9–11 Previous studies have reported that common herbs, such as Persicae Semen, Carthami Flos and Crataegi Fructus, are excellent sources of natural anticoagulants and antioxidants due to their high anticoagulation and antioxidant activities.12–17 According to social research, the use of natural anticoagulants and antioxidants in medicine, health products, cosmetics and food processing is steadily increasing; therefore, it is necessary to identify and research natural anticoagulants and antioxidants from plant sources.
As a traditional Chinese herbal medicine, previous reports and our preliminary experiments simultaneously proved that LJ possesses both high antioxidant and remarkable anticoagulation activities.1,2 However, to date, there have been few studies on the connection between the effects of antioxidant and anticoagulation compounds in LJ. Additionally, LJ is inexpensive and readily available, and it is generally considered as waste in the areas in which it is produced. In the local region, LJ is commonly cremated and/or abundantly discarded in soil and water; no local enterprises collect LJ practically. Moreover, due to its vast planting area and detrimental effects on the environment, LJ is a pressing problem for local farmers, enterprises and governments. The main reason for the waste of LJ is that there are few studies on its applications and adequate value-added processes. Additionally, only limited research has focused on how to transform waste LJ into other valuable products, which undoubtedly leads to further waste of LJ. Therefore, we believe it is imperative to find a new and environmentally friendly method to solve the above problem. An effective method would enable the preparation of the abundant antioxidants and anticoagulants in LJ as valuable products. This could provide a wide area for its development and utilization; this would not only reduce the pressure of waste LJ on abundant planting areas, but would also create more assets for local farmers and decrease environmental pollution.
To isolate natural bioactive compounds from plants, extraction is the first key step. According to previous reports, conventional solvent and Soxhlet methods are commonly used for the extraction of antioxidant and anticoagulant compounds from plants; these methods possess many shortcomings, such as high usage of extraction solvents, long extraction times, inconvenient operation and poor extraction efficiency.18 Currently, increasing numbers of extraction methods, including ultrasound-assisted extraction (UAE),19–21 microwave-assisted extraction (MAE),22 pressurized liquid extraction,23 and supercritical fluid extraction, are being developed for the recovery of antioxidant and anticoagulation compounds from plants.24 Comparatively speaking, MAE and UAE are better than other traditional extraction methods; they can decrease the extraction time and the usage of solvents, optimize the extraction temperature and increase the yields of target compounds.25–28 Particularly, UAE can disrupt cellular walls, allowing better penetration of solvents in the matrix material; this improves mass transfer and increases the release of cell contents.29 Moreover, UAE is the simplest and most economical technique, and it can be readily scaled up for industrial production. Many factors, including ultrasound power, extraction time and temperature, and solvent-to-material ratio, can influence the UAE process individually and collectively; thus, it is difficult to identify main independent variables to optimise.30,31 From previous reports, conventional multivariable optimisation is usually based on single-factor experiments and orthogonal experimental design; this method possesses many disadvantages, such as high cost, more experiments and inaccurate results.32 Response surface methodology (RSM) is a useful tool for evaluating multiple parameters; it can effectively overcome the drawbacks of single-factor experiments and orthogonal experimental design. RSM has been successfully used to optimize the extraction of active compounds from plants, such as polyphenols, polysaccharides and proteins.32–35
Additionally, it is recognized that the simultaneous analysis of acidic and alkaline components is difficult in plant matrices because they can undergo acid-base neutralization reactions.36 LJ contains a variety of alkaloids, flavones, diterpenes and phenolic acids;1 thus, both acidic and alkaline components are present in extracts of LJ. Previous studies showed that high performance liquid chromatography (HPLC) and ultra-performance liquid chromatography tandem mass-spectrometry (UPLC-MS/MS) can be used to analyze the different components of LJ; however, these studies only focused on the determination of alkaline (alkaloids) or acidic (flavones and phenolic acids) components, and there are few studies on the simultaneous determination of alkaline and acidic components in LJ.37–39 To solve the problem of the coexistence of acid and alkaline compounds, in this paper, we developed a method involving ultra-performance liquid chromatography coupled with triple-quadrupole tandem mass spectrometry (UPLC-TQ-MS/MS) for simultaneous determination of alkaline and acidic components in LJ.
Thus, the present study was designed to determine the optimum UAE conditions for maximizing the yields and antioxidant and anticoagulation activities of compounds from LJ. As far as we know, there are no relevant reports on the application of RSM to the optimization of UAE conditions for obtaining remarkable antioxidant and anticoagulation activities of compounds in LJ. Additionally, this is the first time that a rapid and accurate quality control UPLC-TQ-MS/MS method was employed to simultaneously analyze acid and alkaline compounds in LJ.
2. Materials and methods
2.1. Herbal samples
The samples of Leonurus japonicus Houtt. (LJ) were collected in August 2017 from fields in Xuyi County, Jiangsu Province, China. The plant was authenticated by Dr Hui Yan (Department of Medicinal Plants, Nanjing University of Chinese Medicine, Nanjing, China). A voucher specimen (NJUCM-20170830) was deposited in the Herbarium of Nanjing University of Chinese Medicine.
2.2. Chemicals and solvents
All chemicals and reagents were of HPLC grade. The standard of 1,1-diphenyl-2-picrylhydrazyl (DPPH) was purchased from Nanjing Jiancheng Bioengineering Institute (Nanjing, China). Activated partial thromboplastin time (APTT), prothrombin time (PT), thrombin time (TT) and fibrinogen (FIB) assay kits were purchased from Nanjing Maibo Biotech Co. Ltd (Nanjing, China). Other standards of trigonelline (1), stachydrine hydrochloride (2), chlorogenic acid (3), leonurine hydrochloride (4), rutin (5), p-hydroxybenzoic acid (6), syringic acid (7), caffeic acid (8), vanillic acid (9), kaempferol-3-O-rutinoside (10), hyperoside (11), isoquercitrin (12), ferulic acid (13), benzoic acid (14), quercetin (15), apigenin (16), kaempferol (17), isorhamnetin (18), wogonin (19) and genkwanin (20) were purchased from Nanjing Liangwei Biotech Co. Ltd (Nanjing, China). The purity of each compound was >98%. Deionized water was purified by a Milli-Q water purification system from Millipore (MA, USA). Other reagents such as formic acid, acetonitrile and anhydrous ethanol were supplied by Merck (Darmstadt, Germany).
2.3. Determination of extraction yield
The extraction yield of LJ was represented by the total contents of the main components, including alkaloids (1, 2 and 4), flavonoids (5, 11, 12, 15, 16, 17, 18, 19 and 20), phenolic acids (3, 6, 7, 8, 9, 13 and 14) and glycosides (10). These components, the main active compounds in LJ, were identified and determined by UPLC-TQ-MS. UPLC was performed on a Waters Acquity UHPLC system (Waters, Milford, MA, USA) equipped with a quaternary pump solvent management system, an online degasser, a triple quadrupole mass detector and an autosampler. An Acquity UPLC BEH C18 column (2.1 mm × 100 mm, 1.7 μm) was used with an injection volume of 2 μL. The column temperature was maintained at 35 °C. The mobile phase was composed of A (0.1% formic acid solution) and B (acetonitrile solution). The flow rate was set at 0.4 mL min−1. The linear gradient conditions were: 90% A (0 to 1.0 min), 90% to 50% A (1.0 to 7.0 min), 50% to 10% A (7.0 to 13.0 min), 10% to 0% A (13.0 to 14.0 min) and 0% A (14.0 to 14.5 min). A Waters Synapt mass spectrometer (Waters) was used for the mass spectrometry analysis; it was equipped with an electrospray ionization source (ESI) in both positive and negative modes with a scan range of m/z 100 to 1000 Da. The MS parameters are listed in Table 1. Representative MRM chromatograms for the 50 ng mL−1 mixture of the 20 standard analytes and sample are shown in Fig. 1a and b, respectively. Also, the chemical structures of the 20 standards are shown in Fig. 2.
Table 1 MS parameters of the 20 investigated target compounds
Compound |
MW |
MRM transition |
Cone voltage (V) |
Collision energy (eV) |
Rt (min) |
Ion mode |
1 |
137.14 |
138.10 > 91.66 |
30 |
22 |
2.92 |
ES+ |
2 |
179.50 |
144.07 > 84.10 |
36 |
22 |
2.99 |
ES+ |
3 |
354.31 |
353.22 > 191.07 |
18 |
18 |
6.19 |
ES− |
4 |
347.79 |
312.14 > 181.04 |
26 |
24 |
6.94 |
ES+ |
5 |
610.51 |
609.16 > 300.16 |
40 |
32 |
7.00 |
ES− |
6 |
138.13 |
139.00 > 95.01 |
28 |
12 |
7.00 |
ES+ |
7 |
198.18 |
199.04 > 140.01 |
12 |
14 |
7.04 |
ES+ |
8 |
180.15 |
181.10 > 163.06 |
12 |
10 |
7.06 |
ES+ |
9 |
168.15 |
169.00 > 64.99 |
24 |
12 |
7.14 |
ES+ |
10 |
594.52 |
593.22 > 284.82 |
40 |
28 |
7.33 |
ES− |
11 |
464.38 |
465.22 > 303.18 |
12 |
22 |
7.37 |
ES+ |
12 |
464.38 |
463.15 > 301.09 |
14 |
12 |
7.41 |
ES+ |
13 |
194.19 |
195.03 > 144.87 |
14 |
18 |
8.14 |
ES+ |
14 |
122.12 |
123.00 > 78.99 |
24 |
8 |
9.30 |
ES+ |
15 |
302.00 |
301.03 > 151.00 |
30 |
20 |
9.54 |
ES− |
16 |
270.24 |
271.10 > 152.96 |
62 |
30 |
10.18 |
ES+ |
17 |
286.23 |
287.10 > 153.01 |
44 |
28 |
10.39 |
ES+ |
18 |
316.26 |
315.16 > 299.99 |
38 |
22 |
10.44 |
ES− |
19 |
284.27 |
285.10 > 270.07 |
32 |
24 |
12.24 |
ES+ |
20 |
284.27 |
285.08 > 242.02 |
56 |
30 |
12.47 |
ES+ |
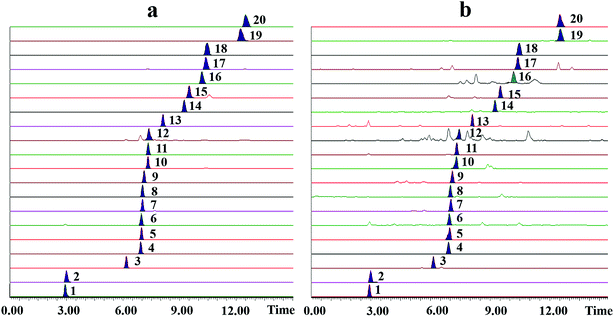 |
| Fig. 1 Representative MRM chromatogram of 20 compounds in 50.0 ng mL−1 mixture standards (a) and a sample (b). Peaks: 1 (trigonelline), 2 (stachydrine hydrochloride), 3 (chlorogenic acid), 4 (leonurine hydrochloride), 5 (rutin), 6 (p-hydroxybenzoic acid), 7 (syringic acid), 8 (caffeic acid), 9 (vanillic acid), 10 (kaempferol 3-O-rutinoside), 11 (hyperoside), 12 (isoquercitrin), 13 (ferulic acid), 14 (benzoic acid), 15 (quercetin), 16 (apigenin), 17 (kaempferol), 18 (isorhamnetin), 19 (wogonin) and 20 (genkwanin). | |
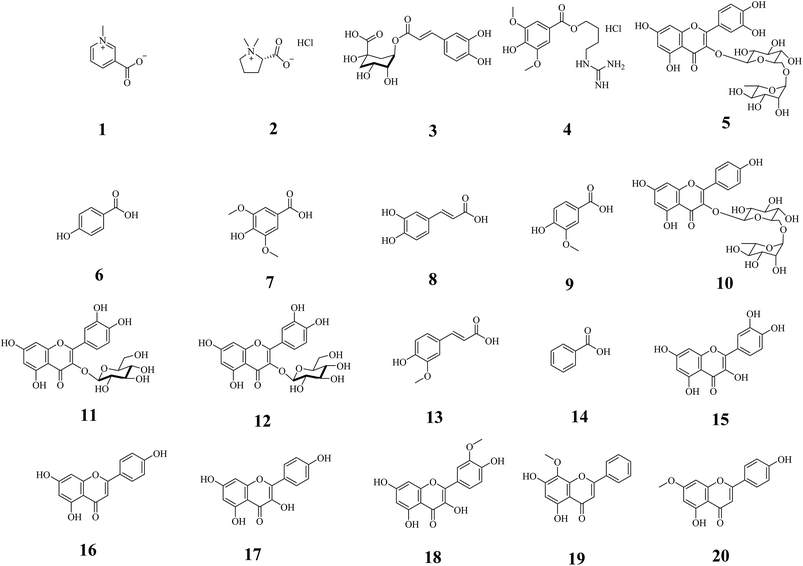 |
| Fig. 2 Chemical structures of the 20 standards in Leonurus japonicus Houtt. | |
In this part, 20 standards were used to identify multiple compounds from LJ, and the contents of the 20 components are displayed as the dry weight of LJ. The extraction yield of LJ was calculated by the following formula:
|
Extraction yield (%) = alkaloids (%) + flavonoids (%) + phenolic acids (%) + glycoside (%)
| (1) |
2.4. Ultrasound-assisted extraction procedure
In this study, UAE was applied for the extraction of multiple components from LJ. An ultrasonic device (KH-500 DB; Kunshan Hechuang Ultrasonic Instrument Factory, Kunshan, China) was used for UAE; this device can be used to adjust and control the sonication power, time and temperature simultaneously. The samples of LJ were cut into segments and crushed into powder, which was filtered through a no. 3 (355 μm ± 13 μm) sieve. Then, the powdered sample (0.5 g) was mixed with ethanol solution in a conical flask. All the samples were extracted in triplicate simultaneously. After UAE, the extracting solution was centrifuged and the supernatant was collected for the subsequent experiments, including UPLC-TQ-MS/MS analysis and the determination of the antioxidant and anticoagulation activities.
2.5. Experimental design
The experiments were executed in three phases. (1) The effects of three solvents, namely water, methanol and ethanol, on the yield of compounds with antioxidant and anticoagulation activities in LJ were compared. (2) Ethanol was chosen as the extraction solvent in the first phase, and the following parameters were investigated: ethanol concentration (10% to 90%), solid–liquid ratio (10
:
1 to 80
:
1 mL g−1), temperature (30 °C to 70 °C), ultrasonic power (200 to 500 W) and ultrasonication time (10 to 90 min). (3) The variables of significance determined from the single-factor experiment were investigated by RSM with Box–Behnken design (BBD). The independent variables (Table 2) were selected based on previous reports.40,46 Also, the 17 BBD design experiments are displayed in Table 3.
Table 2 Independent variables and levels used for the Box–Behnken design
Name, unit |
X1: time (min) |
X2: temperature (°C) |
X3: ethanol concentration (%(v/v)) |
Low |
20 |
30 |
20 |
High |
50 |
70 |
70 |
Table 3 Response surface design and experimental results
Run |
X1 (min) |
X2 (°C) |
X3 (%) |
Y1 (%) |
Y2 (s) |
Y3 (s) |
Y4 (s) |
Y5 (g L−1) |
Y6 (mg mL−1) |
1 |
35 |
50 |
45 |
0.99 |
20.58 |
8.23 |
14.25 |
1.43 |
2.98 |
2 |
50 |
30 |
45 |
0.96 |
19.67 |
8.30 |
18.50 |
1.44 |
3.19 |
3 |
20 |
50 |
70 |
0.93 |
19.80 |
8.80 |
19.67 |
1.49 |
6.92 |
4 |
20 |
70 |
45 |
0.84 |
20.60 |
13.68 |
17.60 |
1.45 |
2.15 |
5 |
35 |
50 |
45 |
0.83 |
20.00 |
8.20 |
14.53 |
1.43 |
2.12 |
6 |
50 |
70 |
45 |
0.84 |
19.40 |
6.73 |
14.33 |
1.44 |
4.89 |
7 |
35 |
30 |
20 |
0.71 |
19.68 |
7.20 |
14.00 |
1.39 |
7.83 |
8 |
35 |
50 |
45 |
0.81 |
19.80 |
8.27 |
12.00 |
1.43 |
2.69 |
9 |
20 |
50 |
20 |
0.80 |
20.25 |
5.00 |
10.73 |
1.43 |
13.50 |
10 |
50 |
50 |
70 |
0.80 |
19.33 |
5.57 |
17.95 |
1.43 |
7.13 |
11 |
20 |
30 |
45 |
0.69 |
19.70 |
5.58 |
14.08 |
1.44 |
3.76 |
12 |
35 |
50 |
45 |
0.84 |
20.27 |
6.08 |
12.63 |
1.43 |
4.46 |
13 |
35 |
70 |
70 |
0.70 |
19.70 |
5.63 |
14.67 |
1.42 |
6.46 |
14 |
35 |
30 |
70 |
1.26 |
19.48 |
8.15 |
14.65 |
1.42 |
6.19 |
15 |
50 |
50 |
20 |
0.63 |
19.37 |
7.60 |
8.45 |
1.40 |
13.46 |
16 |
35 |
70 |
20 |
1.05 |
20.10 |
8.70 |
7.27 |
1.39 |
5.91 |
17 |
35 |
50 |
45 |
0.73 |
20.57 |
8.30 |
9.50 |
1.46 |
4.17 |
2.6. Determination of anticoagulation activity
The anticoagulation activity of LJ was evaluated by activating the TT, PT, APTT and FIB assays in vitro. In this study, blood samples were collected from rabbit auricular vein (no: 2017-021) and deposited into a 10 mL centrifuge tube, where they were soaked in sodium citrate (38 mg mL−1, 800 μL). Then, the above blood samples were centrifuged at 3000 rpm (4 °C) for 15 min and the plasma was acquired for the next experiments. All the animal procedures and experiments were approved by the Animal Ethical Committee of Nanjing University of Chinese Medicine, (Nanjing, China). All experiments were performed according to the guidelines of the National Institute of Health and the institutional rules for the use and care of laboratory animals at Nanjing University of Chinese Medicine (Nanjing, China).
2.6.1. TT assay. TT is mainly reflected by the time of conversion from fibrinogen to fibrin. An increase in TT indicates that the sample has invigorating effects on blood circulation. The specific operation was as follows: 50 μL of LJ extract and 200 μL of plasma were added to the test cup, respectively; then, the mixture was incubated at 37 °C for 3 min. Finally, 200 μL TT reagent were added, and the clotting time (TT value) was recorded.41
2.6.2. PT assay. PT mainly indicates the status of the extrinsic blood coagulation system. When a sample has an invigorating effect on blood circulation, PT will increase. In contrast, PT decreases if the sample causes blood coagulation. In this assay, 25 μL of LJ extract was added to the test cup, followed by 100 μL of plasma. Similarly, the mixture was maintained at 37 °C for 3 min; then, 200 μL PT reagent preheated to 37 °C was added to the test cup, and the coagulation time (PT value) was then recorded.41
2.6.3. APTT assay. APTT mainly indicates the status of the intrinsic blood coagulation system. Like PT, APTT increases when a sample invigorates blood circulation. The specific operation of APTT was as follows: 100 μL of plasma, 25 μL of LJ extract and 100 μL of APTT reagent preheated to 37 °C were added in sequence to the test cup. Then, the above mixture was incubated at 37 °C for 3 min. In the last step, 100 μL of CaCl2 solution preheated to 37 °C was added, and the coagulation time (APTT value) was recorded.41
2.6.4. FIB assay. The FIB assay demonstrates the content of fibrinogen. FIB has a normal range of 2 to 4 g L−1, and its content decreases when the sample plays an invigorating role in the blood circulation. Firstly, we drew a standard curve according to the specified requirements; then, sample determination was performed. 100 μL of LJ extract, 200 μL of plasma and 700 μL of buffer were blended in a tube; then, 200 μL of the mixture was removed and incubated at 37 °C for 3 min. Finally, 100 μL thrombin solution was added to the above mixture and the fibrinogen content was recorded; this content is the FIB value.41For all the tests mentioned above, plasma and breviscapine were used as the blank control and positive control, respectively. The TT, PT, APTT and FIB assays were conducted using a semi-automatic coagulation analyzer.
2.7. Determination of DPPH free radical scavenging activity
To measure the antioxidant activities of the LJ extracts, we adopted the DPPH radical scavenging assay from a previous study with minor alterations.42–45 In brief, DPPH solution was formulated by dissolving DPPH in absolute ethanol at a concentration of 0.1 mM. Then, 100 μL DPPH working solution were added to 100 μL of LJ extracts with various concentrations. Then, each mixture was shaken and incubated for 30 min at room temperature in the dark. The absorbance of each solution was measured on a microplate reader at 517 nm. Necessarily, ascorbic acid (Vc) was used as a positive control. The radical scavenging activity was indicated by the percentage of DPPH according to the following formula: |
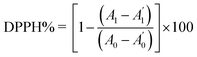 | (2) |
where A1 is the absorbance of the sample (containing DPPH solution), A0 is the absorbance of the control (DPPH solution with no sample),
is the absorbance of the solvent (ethanol solution with sample), and
is the absorbance of the solvent control (ethanol solution with no sample). All the experiments were performed in triplicate and the average values were calculated. The antiradical DPPH activity was shown as IC50 (mg mL−1), which is the concentration of sample required to scavenge 50% of DPPH free radicals.
2.8 Data analysis
All the experiments were performed in triplicate, and Design Expert software version 8.0 (Stat-Ease Inc., Minneapolis, USA) was used to analyse the experimental designs and data. All the results were analyzed by ANOVA, and p-values of less than 0.05 indicated that the data were statistically significant.
3. Results and discussion
3.1. Solvent experiment analysis
Solvents such as water, methanol and ethanol are commonly used for extraction of LJ. In this section, different solvents (water, 20% methanol, 60% methanol, 100% methanol, 20% ethanol, 60% ethanol and 100% ethanol) were respectively selected to study their effects on the yield of the target compounds and the antioxidant and anticoagulation activities of different extractions from LJ. As shown in Fig. 3A–F, in accordance with all the indicators, such as the yield of the target compounds (Y1), ethanol performed better than methanol and water. Meanwhile, the values of TT (Y2), PT (Y3) and APTT (Y4) with ethanol were higher than those with methanol and water. Moreover, for the FIB (Y5) and DPPH free radical scavenging activity (Y6), the values with ethanol were lower than those with methanol and water. Therefore, ethanol was selected as the ideal solvent for the subsequent experiments.43
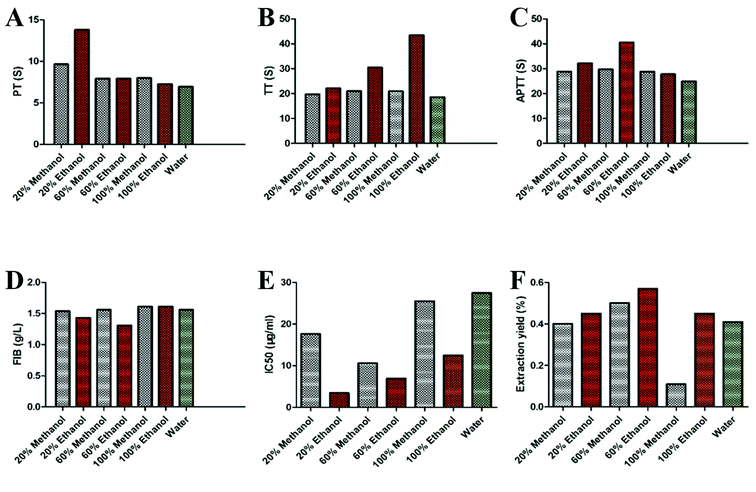 |
| Fig. 3 Effects of different solvents (water, 20% methanol, 60% methanol, 100% methanol, 20% ethanol, 60% ethanol and 100% ethanol) on the extraction yield of LJ (Y1), TT (Y2), PT (Y3), APTT (Y4), FIB (Y5) and DPPH free radical scavenging activity (Y6). | |
3.2. Single factor experiment analysis
3.2.1. Effects of ultrasonication time. The effects of ultrasonication time on the extraction yield of anticoagulant and antioxidant compounds from LJ were investigated at different times (10, 20, 30, 40, 50, 60, 70, 80 and 90 min) while the other extraction conditions were maintained as follows: ultrasonication temperature of 30.0 °C, ethanol concentration of 70.0% (v/v), ultrasonic power of 200.0 W and solid–liquid ratio of 20.0 mL g−1. As shown in Fig. 4, as the ultrasonication time increased from 10 to 50 min, Y1 was enhanced and then showed almost no changes. Y2 to Y4 showed continuous changes between 10 and 50 min. Meanwhile, Y5 decreased from 20 to 50 min and Y6 decreased from 30 to 50 min. Therefore, an ultrasonication time range of 20 to 50 min was chosen as optimal in the BBD experiments.45,46
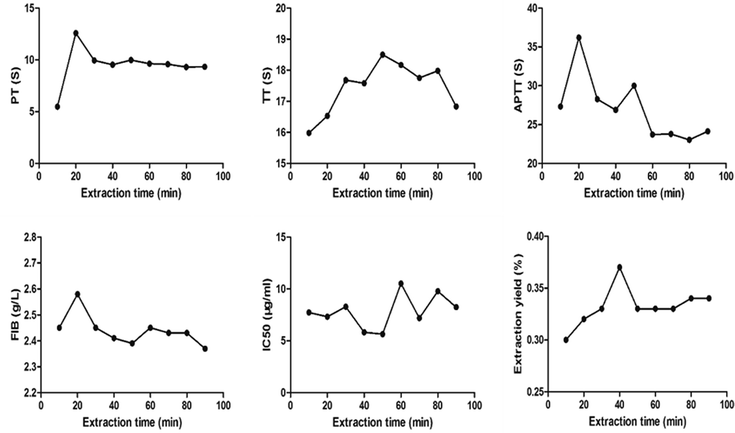 |
| Fig. 4 Effects of ultrasonication time on the extraction yield of LJ (Y1), TT (Y2), PT (Y3), APTT (Y4), FIB (Y5) and DPPH free radical scavenging activity (Y6). | |
3.2.2. Effects of ethanol concentration. In this part, to study the effects of ethanol concentration on the anticoagulant and antioxidant activities of LJ extracts, different ethanol concentrations of 10% to 90% (v/v) were investigated, while the other extraction conditions were maintained as follows: ultrasonication temperature, 30.0 °C; ultrasonication time, 30.0 min; solid–liquid ratio, 20.0 mL g−1; and ultrasonic power, 200.0 W. As shown in Fig. S1,† Y1 to Y4 showed increasing trends as the ethanol concentration increased from 20% to 70%, while Y5 and Y6 decreased to their valley values at ethanol concentrations of 20% and 40%, respectively. Therefore, the ethanol concentration range of 20% to 70% (v/v) was adopted as optimal in the subsequent BBD experiments.45,47
3.2.3. Effects of solid–liquid ratio. The effects of the solid–liquid ratio on the yield of the anticoagulant and antioxidant compounds of LJ were inspected at eight levels, from 10
:
1 to 80
:
1 mL g−1, while the other conditions were maintained as follows: ultrasonication temperature of 30.0 °C, ultrasonication time of 30.0 min, ethanol concentration of 70.0% (v/v) and ultrasonic power of 200.0 W. From Fig. S2,† when the solid–liquid ratios were 10
:
1 to 80
:
1 mL g−1, Y1 to Y6 remained similar and showed no obvious differences. However, when the solid–liquid ratio was 30
:
1 mL g−1, Y1, Y2, Y4 and Y6 were maximal. Thus, 30
:
1 mL g−1 was chosen for the following study to optimize the extraction conditions of LJ.
3.2.4. Effects of temperature. Temperature is an important factor that influences the extraction yield of the anticoagulant and antioxidant compounds of LJ. The effects of different temperatures (30 °C, 40 °C, 50 °C, 60 °C and 70 °C) on the extraction efficiency of LJ were investigated, and the other extraction parameters were maintained as follows: ultrasonication time, 30.0 min; ethanol concentration, 70.0% (v/v); ultrasonic power, 200.0 W; and solid–liquid ratio, 20.0 mL g−1. As shown in Fig. S3,† as the temperature increased from 30 °C to 50 °C, Y1 and Y3 increased. After that, Y1 and Y3 were almost unchanged from 50 °C to 70 °C. Y2 and Y4 showed fluctuations from 30 °C to 70 °C. Y5 and Y6 were similar. Therefore, the temperature range of 30 °C to 70 °C was adopted as optimal in the BBD experiments.
3.2.5. Effects of ultrasonic power. The effects of ultrasonic power on the yield of the anticoagulant and antioxidant compounds of LJ were investigated at seven levels (200, 250, 300, 350, 400, 450 and 500 W), while the other conditions were maintained as follows: ultrasonication temperature, 30.0 °C; ultrasonication time, 30.0 min; ethanol concentration, 70.0% (v/v); and solid–liquid ratio, 20.0 mL g−1. As can be seen in Fig. S4,† Y1 to Y6 were almost unchanged and had no significant differences. Thus, for convenience, the ultrasonic power of 500.0 W was chosen for further optimization experiments of the extraction conditions.
3.3. Analysis of response surface methodology
3.3.1. Fitting the model. Compared with traditional single parameter optimization, RSM is more efficient in that it decreases the number of experiments and saves a remarkable amount of time. A total of 17 experiments were run to optimize the three individual variables (X1: extraction temperature, X2: extraction time and X3: ethanol concentration) in the current BBD, as shown in Table 3. A second order polynomial formula was adopted to correlate the independent variables with the response values of Y1 to Y6. A matched model for Y1 to Y6 was built to predict the relationships between the independent variables, and these relationships can be displayed by the following formulae: |
Y1 = 0.850 − 0.230X2X3
| (3) |
|
Y2 = 20.240 − 0.320X1 − 0.330X32
| (4) |
|
Y3 = 8.020 − 1.170X1X2 − 1.460X1X3 − 1.010X2X3
| (5) |
|
Y4 = 13.180 + 3.310X3 + 2.25X12
| (6) |
|
Y5 = 1.430 + 0.019X3 + 0.022X12 − 0.017X32
| (7) |
|
Y6 = 3.280 − 1.750X3 + 5.030X32
| (8) |
The analysis of variance for the experimental results of the BBD is shown in Table 4. Taking Y2 as an example, the p-value of 0.0407 (p < 0.05) indicates that the model was significant. Meanwhile, the “Lack of Fit” p-value of 0.9986 implied that the lack-of-fit was not significant, which further verified the validity of the model. The determination coefficient (R) was 0.8370, which indicates that the matched model can explain 83.70% of the variations. The adjusted determination coefficient (R-Adj) was 0.6275, which is close to R, showing that the observed values were significantly correlated with the predicted values. Furthermore, the coefficient of variation of 1.3157% indicates that the variation in the average value was low and the high-accuracy experimental results are dependable. All these results display that this model can completely explain the real relationships between the independent variables and response values.34,46,48,49
Table 4 Analysis of variance (ANOVA) for response surface quadratic model, and fits statistics for the response values (n = 3)
Analysis of variance (ANOVA) |
Source |
Y1 |
Y2 |
Y3 |
Y4 |
Y5 |
Y6 |
F-value |
p-value |
F-value |
p-value |
F-value |
p-value |
F-value |
p-value |
F-value |
p-value |
F-value |
p-value |
Model |
3.7031 |
0.0491 |
3.9945 |
0.0407 |
4.1449 |
0.0371 |
4.4179 |
0.0315 |
4.1652 |
0.0366 |
5.7131 |
0.0158 |
X1 |
0.0095 |
0.9249 |
12.1380 |
0.0102 |
0.0048 |
0.9465 |
0.2680 |
0.6207 |
4.0889 |
0.0829 |
0.2163 |
0.6560 |
X2 |
0.5452 |
0.4843 |
2.9411 |
0.1301 |
0.0555 |
0.8205 |
1.7903 |
0.2227 |
0.3307 |
0.5833 |
0.0947 |
0.7672 |
X3 |
3.4688 |
0.1048 |
2.1665 |
0.1845 |
0.0276 |
0.8728 |
23.1388 |
0.0019 |
14.0733 |
0.0072 |
7.6876 |
0.0276 |
X1X2 |
2.1072 |
0.1899 |
4.9924 |
0.0606 |
9.3589 |
0.0183 |
3.9120 |
0.0885 |
0.1925 |
0.6741 |
0.8583 |
0.3850 |
X1X3 |
0.0160 |
0.9029 |
0.6131 |
0.4593 |
14.6232 |
0.0065 |
0.0212 |
0.8884 |
1.1730 |
0.3147 |
0.0046 |
0.9479 |
X2X3 |
22.6297 |
0.0021 |
0.1459 |
0.7138 |
6.9621 |
0.0335 |
3.0063 |
0.1265 |
0.0763 |
0.7903 |
0.3754 |
0.5594 |
X12 |
3.0855 |
0.1224 |
3.1651 |
0.1185 |
3.4024 |
0.1076 |
5.6154 |
0.0496 |
9.1223 |
0.0194 |
4.9454 |
0.0615 |
X22 |
1.5672 |
0.2508 |
1.8704 |
0.2137 |
0.0005 |
0.9823 |
0.5349 |
0.4883 |
3.3366 |
0.1105 |
3.9048 |
0.0887 |
X32 |
0.1372 |
0.7220 |
6.6687 |
0.0363 |
2.4999 |
0.1579 |
1.6846 |
0.2354 |
5.9267 |
0.0451 |
33.4135 |
0.0007 |
Lack of fit |
5.4001 |
0.0685 |
0.0093 |
0.9986 |
3.5579 |
0.1259 |
5.5602 |
0.0654 |
2.2482 |
0.2249 |
6.1624 |
0.0557 |
Credibility analysis of the regression equations |
Index mark |
Y1 |
Y2 |
Y3 |
Y4 |
Y5 |
Y6 |
C.V.% |
11.1499 |
1.3157 |
10.2907 |
13.9154 |
1.0265 |
31.0486 |
R-squared |
0.8264 |
0.8370 |
0.8420 |
0.8503 |
0.8427 |
0.8802 |
Adj R-squared |
0.6033 |
0.6275 |
0.6389 |
0.6578 |
0.6403 |
0.7261 |
3.3.2. Effects of independent variables on the anticoagulant and antioxidant activities of LJ in the RSM model.
3.3.2.1. Extraction yield of LJ (Y1). To study the interactive effects of the independent variables on the extraction yield of LJ, three-dimensional response surfaces are shown in Fig. 5A–C and contour plots are shown in Fig. 5a–c. Fig. 5A and a show the interactive effects between ethanol concentration and temperature while the ultrasonication time remains at 0-level. When the temperature was fixed, Y1 rapidly increased with increasing ethanol concentration until it reached a maximum; then, it slowly decreased. These results indicate that most of the target components (medium-polarity compounds) from LJ can be easily dissolved in highly concentrated ethanol solution. Therefore, to improve the solubility of the medium-polarity target compounds, a higher ethanol concentration was used to enhance the value of Y1. Fig. 5B and b show the interactive effects between ethanol concentration and ultrasonication time while the temperature remains at 0-level. The value of Y1 remarkably improved with increasing ethanol concentration from 20% to 60% (v/v), while Y1 increased slightly with increasing ultrasonication time from 30 to 50 min. The main reason for this may be saturation of the solution; as the time increased, most components were extracted completely. Fig. 5C and c show the interactive effects between the temperature and ultrasonication time while the ethanol concentration was fixed. The value of Y1 increased remarkably with increasing temperature, while the changes in the ultrasonication time had almost no effect on Y1 after 50 min. The above results are in accordance with the ANOVA (Table 4). These results indicate that X2, X3 and X2X3 are significant (p < 0.05) for Y1.
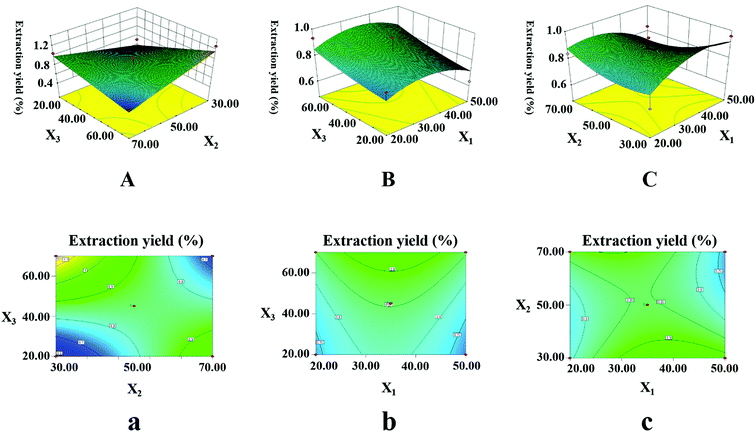 |
| Fig. 5 Response surface (3-D) and contour plots showing the effects of (A and a) extraction temperature (X2) and ethanol concentration (X3), (B and b) ultrasonication time (X1) and ethanol concentration (X3) and (C and c) ultrasonication time (X1) and extraction temperature (X2) on the extraction yield of LJ (Y1). | |
After optimization by the BBD software, the optimum conditions were an ultrasonication time of 41.2 min, a temperature of 30.0 °C, an ethanol concentration of 70.0% (v/v), a solid–liquid ratio of 30.0 mL g−1 and an ultrasonic power of 500.0 W.
3.3.2.2. TT assay (Y2). To explain the interactive effects on the anticoagulation activities of LJ extracts, three dimensional response surfaces are displayed in Fig. 6A–C; the contour plots are shown in Fig. 6a–c. Fig. 6A and a show the interactive effects between ethanol concentration and temperature with a fixed ultrasonication time. Y2 showed an enhancement and then decreased slightly when the ethanol concentration increased from 20% to 50% (v/v) and the temperature increased from 30 °C to 50 °C. Most of the components of LJ dissolved easily in highly concentrated ethanol solution. At the same time, with increasing temperature, the molecular movement became violent, resulting in complete extraction of the LJ. More extracted components may promote invigoration of the blood circulation. However, when the solution became saturated, the increases in ethanol concentration and temperature became unimportant. Fig. 6B and b show the interactive effects between ethanol concentration and ultrasonication time at a fixed temperature. As before, Y2 improved remarkably when the ethanol concentration increased from 20% to 60%. Fig. 6C and c show the interactive effects between the temperature and ultrasonication time with a stable ethanol concentration. Y2 increased as the temperature increased from 30 °C to 60 °C. However, extension of the ultrasonication time did not greatly affect Y2. The ANOVA results (Table 4) showed that X1 and X32 were significant (p < 0.05) for Y2 and that X1 and X3 are the major factors affecting Y2. Therefore, for Y2, the optimum conditions were found to be as follows: ultrasonication time, 20.0 min; temperature, 70.0 °C; ethanol concentration, 34.1% (v/v); solid–liquid ratio, 30.0 mL g−1; and ultrasonic power, 500.0 W.
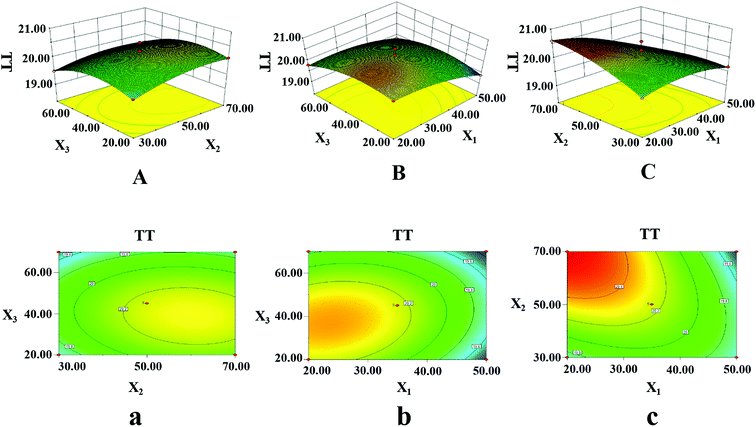 |
| Fig. 6 Response surface (3-D) and contour plots showing the effects of (A and a) extraction temperature (X2) and ethanol concentration (X3), (B and b) ultrasonication time (X1) and ethanol concentration (X3) and (C and c) ultrasonication time (X1) and extraction temperature (X2) on TT (Y2). | |
3.3.2.3. PT assay (Y3). The three-dimensional response surfaces and contour plots for Y3 are displayed in Fig. S5A–C and Fig. S5a–c,† respectively. Firstly, Fig. S5A and a† show the interactive effects between ethanol concentration and temperature with a fixed ultrasonication time. When the ethanol concentration increased, Y3 improved. Secondly, Fig. S5B and b† show the interactive effects between ethanol concentration and ultrasonication time at a stable temperature. Obviously, Y3 was affected by the ethanol concentration. Finally, Fig. S5C and c† showed the interactive effects between the temperature and ultrasonication time at a fixed ethanol concentration. Y3 decreased markedly with increasing temperature and ultrasonication time. The above results are in accordance with the ANOVA (Table 4), which showed that X1X2, X1X3 and X2X3 are significant (p < 0.05) for Y3. For Y3, the optimum conditions were found to be as follows: ultrasonication time, 20.0 min; temperature, 70.0 °C; ethanol concentration, 53.7% (v/v); solid–liquid ratio, 30.0 mL g−1; and ultrasonic power, 500.0 W.
3.3.2.4. APTT assay (Y4). Fig. S6A–C and Fig. S6a–c† show how the ethanol concentration, temperature and ultrasonication affect the blood circulation invigoration activities of LJ extract. From the results, ethanol concentration plays the most important role of the three independent variables. Y4 showed an obvious improvement with increasing ethanol concentration and showed no changes with increasing temperature and ultrasonication time. This analysis was in keeping with the ANOVA (Table 4), which showed that X3 is the major factor affecting Y4 and that X12 and X3 are significant (p < 0.05) for Y4. For Y4, the optimum conditions were found to be as follows: ultrasonication time, 20.0 min; temperature, 70.0 °C; ethanol concentration, 70.0% (v/v); solid–liquid ratio, 30.0 mL g−1; and ultrasonic power, 500.0 W.
3.3.2.5. FIB assay (Y5). Y5 is closely connected with the blood circulation invigorating activities of LJ extract. Next, Fig. S7A–C and Fig. S7a–c† show the relationships between the three independent variables and Y5. Fig. S7A and a† show the interactive effects of ethanol concentration and temperature with a fixed ultrasonication time. Y5 decreased as the ethanol concentration increased. Fig. S7B and b† show the interactive effects of ethanol concentration and ultrasonication time at a stable temperature. The ethanol concentration also affected Y5 remarkably, while the ultrasonication time showed no effects. Fig. S7C and c† show the interactive effects of temperature and ultrasonication time at a constant ethanol concentration. Y5 decreased with increasing temperature. The above results are in line with the ANOVA (Table 4), which indicates that X12, X32 and X3 are significant (p < 0.05) for Y5 and that X3 is the major factor affecting Y5. For Y5, the optimum extraction parameters were found to be as follows: ultrasonication time of 38.3 min, temperature of 30.1 °C, ethanol concentration of 20.4% (v/v), solid–liquid ratio of 30.0 mL g−1 and ultrasonic power of 500.0 W.
3.3.2.6. DPPH free radical scavenging activity (Y6). To investigate the interactive effects on the antioxidant activities of LJ extract, three dimensional response surface and contour plots were drawn and are displayed in Fig. S8A–C and Fig. S8a–c,† respectively. First, Fig. S8A and a† show that increasing the ethanol concentration led to a rapid decrease of Y6 when the ultrasonication time was fixed. Fig. S8B and b† show that Y6 decreased with increasing ethanol concentration and ultrasonication time at the same time. Fig. S8C and c† show that when the ethanol concentration was stable, Y6 changed slightly with increasing temperature and ultrasonication time. The above results are in keeping with the ANOVA (Table 4), which shows that X3 and X32 are significant (p < 0.05) for Y6 and that X3 is the major factor affecting Y6. Therefore, for Y6, the optimum parameters were found to be as follows: ultrasonication time, 30.6 min; temperature, 70.0 °C; ethanol concentration, 48.1% (v/v); solid–liquid ratio, 30.0 mL g−1; and ultrasonic power, 500.0 W.
3.3.3. Validation of the predicted value. In consideration of the multiple indicators of this experiment model, it was difficult to consider all of them. Therefore, the function of Design Expert software version 8.0 called ‘Numerical Optimization’ was used to coordinate all the conditions. Then, we acquired the optimal ultrasonic extraction conditions according to the model equation by predicting the response values: ultrasonication time, 38.2 min; temperature, 30.0 °C; ethanol concentration, 48.9% (v/v); solid–liquid ratio, 30.0 mL g−1; and ultrasonic power, 500.0 W. Under the optimal extraction conditions, the experimental values (extraction yield of LJ = 0.994%, TT = 19.872 s, PT = 8.270 s, APTT = 15.535 s, FIB = 1.420 g L−1 and DPPH = 1.503 mg mL−1) were acquired was and were well matched with the predicted values (extraction yield of LJ = 0.992%, TT = 19.876 s, PT = 8.249 s, APTT = 15.452 s, FIB = 1.420 g L−1 and DPPH = 1.498 mg mL−1). The excellent correlations between the experimental and predicted values indicated that the model obtained by BBD can accurately predict the anticoagulant and antioxidant extraction yield of LJ using UAE.
4. Conclusion
In this study, UAE was successfully optimized for the maximum extraction of natural antioxidants and anticoagulants from LJ using BBD. Five impact factors of extraction (ethanol concentration, solid–liquid ratio, ultrasonication time, ultrasonic power and temperature) were evaluated, and the results indicated that ethanol concentration, ultrasonication time and temperature have significant effects on the yield of antioxidants and anticoagulants extracted from LJ. Furthermore, the optimal technology was determined to be an ultrasonication time of 38.2 min; a temperature of 30.0 °C; an ethanol concentration of 48.9% (v/v); a solid–liquid ratio of 30.0 mL g−1; and an ultrasonic power of 500.0 W. These results demonstrate that UAE coupled with RSM is an effectual and feasible method for the extraction of natural antioxidants and anticoagulants from LJ; this will provide a scientific basis for the comprehensive utilization and development of LJ.
Conflicts of interest
There are no conflicts to declare.
Acknowledgements
This work was financially supported by the National Standardization Project for Traditional Chinese Medicines (ZYBZH-C-JS-34), National Natural Science Foundation of China (81573714, 81603257, 81773882, 81781260279 and 81803699) and 333 High-level Talents Training Project Funded by Jiangsu Province (BRA2016387). This research was also financially supported by Jiangsu Collaborative Innovation Center of Chinese Medicinal Resources Industrialization (No. 012092002006-4), A Project Funded by the Priority Academic Program Development of Jiangsu Higher Education Institutions (PAPD) and China Agriculture Research System (CARS-21). We are also pleased to thank Waters Ltd. for technical support.
References
- X. Shang, H. Pan, X. Wang, H. He and M. Li, J. Ethnopharmacol., 2014, 152, 14–32 CrossRef CAS.
- A. S. Oliveira, L. M. Cercato, M. T. de Santana Souz, A. J. de Oliveira Melo, B. d. S. Lima, M. C. Duarte, A. A. de Souza Araujo, A. M. de Oliveira e Silva and E. A. Camargo, J. Ethnopharmacol., 2017, 206, 144–151 CrossRef CAS.
- H. Nagasawa, H. Inatomi, M. Suzuki and T. Mori, Anticancer Res., 1992, 12, 141–143 CAS.
- H. Q. Pang, S. J. Yue, Y. P. Tang, Y. Y. Chen, Y. J. Tan, Y. J. Cao, X. Q. Shi, G. S. Zhou, A. Kang, S. L. Huang, Y. J. Shi, J. Sun, Z. S. Tang and J. A. Duan, Front. Pharmacol., 2018, 9, 1–18 CrossRef PubMed.
- S. W. Park, R. Vepachedu, N. Sharma and J. M. Vivanco, Planta, 2004, 219, 1093–1096 CrossRef CAS.
- C. Kaur and H. C. Kapoor, Int. J. Food Sci. Technol., 2001, 36, 703–725 CrossRef CAS.
- A. Kumar and A. Singh, Front. Pharmacol., 2015, 6, 1–13 CAS.
- I. S. Young and J. V. Woodside, J. Clin. Pathol., 2001, 54, 176–186 CrossRef CAS PubMed.
- A. W. Boots, G. R. M. M. Haenen and A. Bast, Eur. J. Pharmacol., 2008, 585, 325–337 CrossRef CAS PubMed.
- S. Li, H. Y. Tan, N. Wang, Z. J. Zhang, L. Lao, C. W. Wong and Y. Feng, Int. J. Mol. Sci., 2015, 16, 26087–26124 CrossRef CAS PubMed.
- F. M. F. Roleira, E. J. Tavares-da-Silva, C. L. Varela, S. C. Costa, T. Silva, J. Garrido and F. Borges, Food Chem., 2015, 183, 235–258 CrossRef CAS PubMed.
- G. F. Deng, C. Shen, X. R. Xu, R. D. Kuang, Y. J. Guo, L. S. Zeng, L. L. Gao, X. Lin, J. F. Xie, E. Q. Xia, S. Li, S. Wu, F. Chen, W. H. Ling and H. B. Li, Int. J. Mol. Sci., 2012, 13, 8308–8323 CrossRef CAS.
- M. E. Embuscado, J. Funct. Foods, 2015, 18, 811–819 CrossRef CAS.
- E. N. Frankel and J. W. Finley, J. Agric. Food Chem., 2008, 56, 4901–4908 CrossRef CAS.
- P. V. Hung, Crit. Rev. Food Sci. Nutr., 2016, 56, 25–35 CrossRef PubMed.
- S. Li, S. K. Li, R. Y. Gan, F. L. Song, L. Kuang and H. B. Li, Ind. Crops Prod., 2013, 51, 289–298 CrossRef CAS.
- F. Shahidi and P. Ambigaipalan, J. Funct. Foods, 2015, 18, 820–897 CrossRef CAS.
- B. Gullon, T. A. Lu-Chau, M. T. Moreira, J. M. Lema and G. Eibes, Trends Food Sci. Technol., 2017, 67, 220–235 CrossRef CAS.
- M. Arshadi, T. M. Attard, R. M. Lukasik, M. Brncic, A. M. d. C. Lopes, M. Finell, P. Geladi, L. Noemi Gerschenson, F. Gogus, M. Herrero, A. J. Hunt, E. Ibanez, B. Kamm, I. Mateos-Aparicio, A. Matias, N. E. Mavroudis, E. Montoneri, A. R. C. Morais, C. Nilsson, E. H. Papaioannou, A. Richel, P. Ruperez, B. Skrbic, M. B. Solarov, J. Svarc-Gajic, K. W. Waldron and F. J. Yuste-Cordoba, Green Chem., 2016, 18, 6160–6204 RSC.
- F. Dahmoune, H. Remini, S. Dairi, O. Aoun, K. Moussi, N. Bouaoudia-Madi, N. Adjeroud, N. Kadri, K. Lefsih, L. Boughani, L. Mouni, B. Nayak and K. Madani, Ind. Crops Prod., 2015, 77, 251–261 CrossRef CAS.
- L. Liu, B. J. Shen, D. H. Xie, B. C. Cai, K. M. Qin and H. Cai, Pharmacogn. Mag., 2015, 11, 682–689 CrossRef CAS PubMed.
- F. Dahmoune, B. Nayak, K. Moussi, H. Remini and K. Madani, Food Chem., 2015, 166, 585–595 CrossRef CAS PubMed.
- D. Povilaitis, V. Sulniute, P. R. Venskutonis and V. Kraujaliene, J. Cereal Sci., 2015, 62, 117–123 CrossRef CAS.
- B. Diaz-Reinoso, A. Moure, H. Dominguez and J. C. Parajo, J. Agric. Food Chem., 2006, 54, 2441–2469 CrossRef CAS PubMed.
- Z. Zeković, A. Cvetanović, J. Švarc-Gajić, S. Gorjanović, D. Sužnjević, P. Mašković, S. Savić, M. Radojković and S. Đurović, Ind. Crops Prod., 2017, 108, 423–430 CrossRef.
- P. Mašković, V. Veličković, M. Mitić, S. Đurović, Z. Zeković, M. Radojković, A. Cvetanović, J. Švarc-Gajić and J. Vujić, Ind. Crops Prod., 2017, 109, 875–881 CrossRef.
- P. Mašković, V. Veličković, S. Đurović, Z. Zeković, M. Radojković, A. Cvetanović, J. Švarc-Gajić, M. Mitić and J. Vujić, Phytomedicine, 2017, 38, 118–124 CrossRef PubMed.
- V. Veličković, S. Đurović, M. Radojković, A. Cvetanović, J. Švarc-Gajić, J. Vujić, S. Trifunović and P. Mašković, J. Supercrit. Fluids, 2017, 128, 331–337 CrossRef.
- E. Backes, C. Pereira, L. Barros, M. A. Prieto, A. K. Genena and M. F. Barreiro, et al., Food Res. Int., 2018, 113, 197–209 CrossRef CAS PubMed.
- M. D. Esclapez, J. V. Garcia-Perez, A. Mulet and J. A. Carcel, Food Eng. Rev., 2011, 3, 108–120 CrossRef.
- O. Laguna, A. Barakat, H. Alhamada, E. Durand, B. Barea, F. Fine, P. Villeneuve, M. Citeau, S. Dauguet and J. Lecomte, Ind. Crops Prod., 2018, 118, 160–172 CrossRef CAS.
- T. Zhang, A. Sharma, Y. Li, Y. Zhou and X. Ding, J. Photochem. Photobiol., A, 2018, 178, 568–576 CrossRef CAS PubMed.
- C. Li, H. Song, Y. Jiao and H. Gao, Sci. Technol. Food Ind., 2016, 37, 204–209 CAS.
- I. Rodriguez Amado, D. Franco, M. Sanchez, C. Zapata and J. Antonio Vazquez, Food Chem., 2014, 165, 290–299 CrossRef PubMed.
- S. Y. Yang, S. W. Kim, Y. Kim, S. H. Lee, H. Jeon and K. W. Lee, Food Chem., 2015, 176, 420–425 CrossRef CAS PubMed.
- M. Pei, X. Duan and X. Pei, China J. Chin. Mater. Med., 2009, 34, 2047–2050 Search PubMed.
- X. Cheng, X. Sang, Q. Yang, J. Meng, X. Tang and C. Zhang, Chin. J. Hosp. Pharm., 2013, 33, 1850–1852 CAS.
- B. Liu and Y. Chen, J. Shenyang Pharm. Univ., 2016, 33, 303–306 CAS.
- C. Zhou, Y. Zhang, Y. He, Y. Wang, J. Yu, Z. Dai and S. Ma, Chin. J. Pharm. Anal., 2016, 36, 830–834 CAS.
- S. Tahmouzi and M. Ghodsi, Carbohydr. Polym., 2014, 112, 396–403 CrossRef CAS PubMed.
- Z. Yin, W. Zhang, J. Zhang and W. Kang, Chem. Cent. J., 2017, 11, 1–10 CrossRef PubMed.
- A. S. Ahmed, E. E. Elgorashi, N. Moodley, L. J. McGaw, V. Naidoo and J. N. Eloff, J. Ethnopharmacol., 2012, 143, 826–839 CrossRef PubMed.
- A. Pandey, T. Belwal, K. C. Sekar, I. D. Bhatt and R. S. Rawal, Ind. Crops Prod., 2018, 119, 218–225 CrossRef CAS.
- K. H. Wong, G. Q. Li, K. M. Li, V. Razmovski-Naumovski and K. Chan, Food Chem., 2017, 231, 231–237 CrossRef CAS PubMed.
- D. P. Xu, J. Zheng, Y. Zhou, Y. Li, S. Li and H.-B. Li, Food Chem., 2017, 217, 552–559 CrossRef CAS PubMed.
- S. Chen, Z. Zeng, N. Hu, B. Bai, H. Wang and Y. Suo, Food Chem., 2018, 242, 1–8 CrossRef CAS PubMed.
- A. B. Das, V. V. Goud and C. Das, Ind. Crops Prod., 2017, 95, 332–341 CrossRef CAS.
- X. Guo, X. Shang, X. Zhou, B. Zhao and J. Zhang, Ultrason. Sonochem., 2017, 38, 246–255 CrossRef CAS PubMed.
- H. Zhang, J. Birch, C. Xie, H. Yang, G. Dias, L. Kong and A. E. D. Bekhit, Ind. Crops Prod., 2018, 119, 191–200 CrossRef CAS.
Footnote |
† Electronic supplementary information (ESI) available. See DOI: 10.1039/c8ra07361a |
|
This journal is © The Royal Society of Chemistry 2018 |