DOI:
10.1039/C8RA07163B
(Paper)
RSC Adv., 2018,
8, 40894-40911
Retracted Article: Yiqi Fumai lyophilized injection attenuates doxorubicin-induced cardiotoxicity, hepatotoxicity and nephrotoxicity in rats by inhibition of oxidative stress, inflammation and apoptosis†
Received
28th August 2018
, Accepted 22nd November 2018
First published on 6th December 2018
Abstract
Doxorubicin (DOX) is one of the most effective antineoplastic drugs, however, its organ toxicity inhibits the clinical utility. This study was aimed at investigating the protective effects of Yiqi Fumai lyophilized injection (YQFM) against DOX-induced tissue injury and exploring the mechanisms which mediated reactive oxygen species (ROS), inflammation and apoptosis. The experiment was as follows: rats were subjected to an intraperitoneal injection (i.p.) of YQFM (0.481 g kg−1, i.p.) for 12 days; DOX (5 mg kg−1, i.p.) was administered on the 4th, 8th and 12th days to achieve a cumulative dose of 15 mg kg−1. Pretreatment of YQFM significantly ameliorated intracellular damage and dysfunction of the heart, liver and kidneys via decreasing activities of injury indexes. The levels of lipid peroxidation and glutathione depletion were clearly reduced following YQFM pretreatment, meanwhile the activities of glutathione peroxidase, superoxide dismutase, and catalase were elevated. Additionally administering YQFM could mitigate the cardiotoxicity, hepatotoxicity and nephrotoxicity via reducing levels of inflammatory factors and decreasing apoptosis. Accordingly, this study indicated that YQFM attenuated DOX-induced toxicity by ameliorating organ function, decreasing ROS production, and preventing excessive inflammation and apoptosis.
1. Introduction
Doxorubicin (DOX) is an anthracycline anticancer drug, which can treat a variety of human neoplasms such as leukemias, lymphomas, breast cancer, sarcomas and other malignancies.1,2 However, DOX has a main adverse effect, cardiotoxicity, which limits its clinical application. In addition, researchers find that DOX can also lead to severe hepato-renal toxicities. Although the pathogenesis of DOX-induced organ damage has not been found entirely, there exist several possible mechanisms of cardiotoxicity related to the increased oxidative stress, cardiomyocyte apoptosis, calcium dynamics interference, DNA damage, release of vasoactive amines, and altered adrenergic function.3,4 Apart from cardiotoxicity, it is also reported that DOX induces hepatocyte damage which is caused by proinflammatory cytokines, oxidative stress and apoptosis.5,6 Although the mechanisms responsible for DOX-induced toxicity are still not quite clear, most researchers find that nicotinamide adenine dinucleotide phosphate– (NADPH–) dependent cellular reductase can transform DOX into semiquinone free radicals which can increase reactive oxygen species (ROS) to damage lipids and proteins, eventually leading to organ injuries.7,8 Unfortunately, DOX leads to severe clinical toxicities in kidney too.9 There are many studies indicating that DOX-induced nephrotoxicity is caused by ROS which induces lipid peroxidation, damages renal cells, and increases glomerular capillary permeability and tubular atrophy.9,10 Yiqi Fumai lyophilized injection (YQFM) is a kind of modern injection based on a well-known traditional Chinese medicine (TCM) complex prescription Sheng-mai San. It was approved in 2012 by the China Food and Drug Administration for clinical. Clinically, many evidences indicate that YQFM has a good clinical efficiency on treating chronic heart failure, hypertrophic obstructive cardiomyopathy, ischemic heart disease and other microcirculatory disturbance-related diseases.11–13 YQFM is extracted from three traditional medicinal herbs, including Ginseng Radix et Rhizoma Rubra, Ophiopogonis Radix and Schisandrae chinensis Fructus.14 According to the previous reports, there are sixty-five compounds in YQFM. For the twenty-one compounds of them, there are fifteen ginsenosides, three ophiopogonins and three lignans quantified by UFLC-IT-TOF/MS.15 What's more, the main components of YQFM displayed antioxidant, inflammatory modulation and anti-apoptosis activity. Major compounds including ginsenoside Re, Rg3, Rg1, Rh1 and Rb1 can protect cardiomyocytes, liver and kidney.16–21
Briefly, YQFM is mainly used in cardiovascular diseases in clinical, and its main compounds have an effect on hepatorenal protection, while the main side effect of DOX is organ toxicity. Although it is reported that many medicines can separately treat DOX-induced cardiotoxicity, hepatotoxicity and nephrotoxicity, there are few of them have an effect on relieving all these organ toxicities at the same time. Moreover, as an injection, YQFM can be directly and effectively medical and avoid the first-pass effect caused by oral administration so as to play a better therapeutic role. That's to say, it is worthy to explore whether YQFM has a good influence on DOX-induced toxicities. Thus, it is really important to find if YQFM can prevent or treat DOX-induced organ toxicity and the potential pharmacological mechanisms underlying YQFM's actions. Therefore the present study is used to discover the possible treatment pathway and may offer a theoretical basis for the further research of YQFM and the clinical application of DOX.
2. Materials and methods
2.1 Chemicals and materials
DOX was obtained from Shanghai Yuanye Biological Technology Co., Ltd (Shanghai, China). YQFM was provided by Tasly Pride Pharmaceutical Company Limited (Tianjin, China). Superoxide (SOD), reduced glutathione (GSH), glutathione peroxidase (GPx), catalase (CAT), malondialdehyde (MDA), lactate dehydrogenase (LDH), aspartate aminotransferase (AST), alanine aminotransferase (ALT), alkaline phosphatase (ALP), blood urea nitrogen (BUN), creatinine (Cr), inducible nitric oxide synthase (iNOS) and nitric oxide (NO) assay kit were all purchased from Nanjing Jiancheng Bioengineer Institute (Nanjing, China). Antibodies against cleaved caspase-3, cyclooxygenase-2 (COX-2), BAX, Bcl-2, cytochrome c, nephrin, NPHS2 and transforming growth factor β1 (TGF-β1) secondary anti-rabbit antibodies were obtained from Abcam, (Cambridge, UK). Rat creatine kinase isoenzyme-MB (CK-MB), nuclear factor κB (NF-κB), and TNF-α ELISA kits were purchased from MDL (Beijing, China). Dihydroethidium (DHE) was purchased from Servicebio (Wuhan, China).
Reference standard compounds of ginsenosides Rb1, Rd, Re, Rf, Rg1, 20(R)-ginsenoside Rg3, 20(S)-ginsenoside F2, ophiopogonin D, schizandrol B, schisandrin B and schisandrin were purchased from Shanghai Standard Technology Co., Ltd (Shanghai, China).
2.2 Animals
Male Sprague-Dawley rats (250–350 g) were purchased from Beijing HFK Biotechnology Co., Ltd, SCXK-2014-0004. All rats were acclimatized for one week before the initiation of experimentation. The animals were kept in cages at 22 ± 2 °C with 12 h light and dark cycles and a humidity of 40 ± 5%, and they were provided with rat chow and water ad libitum. The experimental procedure used in this study met the guidelines of the Animal Care and Use. All animal procedures were performed in accordance with the Guidelines for Care and Use of Laboratory Animals of Tianjin Medical University, Tianjin, China in accordance with “Principles of Laboratory Animal Care and Use in Research” (State Council of China, 1988) and experiments were approved by the Animal Ethics Committee of Tianjin Experimental Animal Center SYXK-2014-0002.
2.3 Determination of main compounds in YQFM
2.3.1 Standard and sample solutions for qualitative analysis. Eleven standard compounds were accurately weighed (1.0 mg of each) and dissolved in 10 mL of methanol in a volumetric flask to prepare mixed standard solution. A 0.45 μm syringe filter was used to filter before analysis. Solutions were all kept at 4 °C.15,22Accurately weighed YQFM (1.30 g) was dissolved in 30% methanol, and then pretreated with C8 solid-phase extraction (SPE) to remove interferences.22 Firstly, the SPE was activated with methanol (10 mL) and followed by deionized water (10 mL). Secondly, the sample solution was added into SPE and the elution rate was 1 mL min−1. Sample was eluted sequentially with 30% methanol solution containing 0.5 mol L−1 sodium hydroxide (2 mL), 30% methanol (5 mL) and 100% methanol (5 mL). The 100% methanol was collected and added with methanol to 5 mL in a volumetric flask. Then a 0.45 μm membrane was used to filter the solution.22 Solution was also kept at 4 °C.
2.3.2 Qualitative analysis conditions. The high performance liquid chromatography (HPLC) system (Agilent Technologies 1200 Series, USA) was equipped with a quadrupole-time of flight tandem mass spectrometer (MicrOTOF-Q-II, Bruker Daltonics Inc. USA). The Diamonsil® C18 column (5 μm, 250 mm × 4.6 mm) was used to perform the chromatographic separation and the column temperature was kept at 30 °C. The flow rate of the mobile phase which consisting of 0.01% (v/v) formic acid in ultrapure water (A) and acetonitrile (B), was 1 mL min−1 and injection volume was 35 μL. The gradient elution program was shown as follows: 0–8 min, 20–30% B; 8–10 min, 30–32% B; 10–14 min, 32–34% B; 14–15 min, 34–35% B; 15–26 min, 35–35% B; 26–30 min, 35–37% B; 30–35 min, 37–40% B; 35–40 min, 40–45% B; 40–50 min, 45–55% B; 50–60 min, 55–70% B; 60–65 min, 70–95% B; 65–67 min, 95–95% B; 67–72 min, 95–98% B; 72–77 min, 98–20% B; 77–81 min, 20–20% B.15
2.4 Experimental design
Rats were randomly divided into four groups and treated as follows: control group (control, n = 10) received an intraperitoneal injection (i.p.) of normal saline (0.4 mL kg−1), the DOX control group (DOX, n = 10) received an intraperitoneal injection of 5 mg kg−1 DOX dissolved in normal saline on 4th, 8th and 12th day to obtain a cumulative dose of 15 mg kg−1 over a period of 12 days. In clinical, the adult dose of YQFM was 0.087 g kg−1, then according to interspecies equivalent dose standard, the equivalent in rats was 0.481 g kg−1 as YQFM (0.481 g kg−1, i.p. n = 10) group. And the next group was pretreated with an intraperitoneal injection of YQFM (0.481 g kg−1) for 12 consecutive days followed by three intraperitoneal injection of DOX (5 mg kg−1) on 4th, 8th and 12th day to get a cumulative dose of 15 mg kg−1 (DOX + YQFM, n = 10).23
Body weight was measured at the beginning of the study to acquire the basal level. Before the experiment and twenty-four hours later after the last dose of DOX injection, rats were anesthetized with chloral hydrate (10%, 0.3 mL/100 g; i.p.) and the cardiac function of the rats was evaluated by performing transthoracic echocardiography.24 Blood samples were collected from femoral artery and centrifuged at 3000 rpm for 15 min to obtain the serum used for biochemical analyses. Then, the next steps were to sacrifice rats and measure weights of hearts, livers and kidneys. The tissue index was calculated by equation. Tissue index = (tissue weight/body weight) × 100. Part of heart, liver and kidney tissues were homogenized in saline, and then the homogenates were used for estimating different biochemical parameters. Moreover, cardiac, hepatic and kidney specimens from different groups were fixed in 4% paraformaldehyde for histopathological and immunohistochemical examination. Some parts of tissues were kept at −80 °C until they were used for western blot analysis.25 And the rest parts of tissues were made into frozen section by freezing microtome (Thermo, Cryotome E) for the detection of reactive oxygen species (ROS).
2.5 Echocardiography
Echocardiography was recorded twice by an animal specific instrument (VisualSonics Vevo 2100 cardiac system) before the study and after 24 h of last dose of injections. Under inhaled isoflurane at 3.5%, rats were anaesthetized and kept in an induction chamber. Then data was measured from an M-mode image which was obtained after observing at least 6 cardiac cycles. Interventricular septal thickness (IVS), left ventricular diameter (LVD) and left ventricular posterior wall thickness (LVPW) were measured during systole (s) and diastole (d). Some formulae were used to calculate left ventricular mass, ejection fraction and fractional shortening. Left ventricular mass = 1.04 × ((LVDd + LVPWd + IVSd)3 − (LVDd)3) × 0.8 + 0.14, relative wall thickness = 2 × (LVPWd/LVDd). [% EF = (LVDd)3 − (LVDs)3/(LVDd)3 × 100] for ejection fraction and [%FS = LVDdLVDs/LVDd × 100] for fractional shortening.26
2.6 Cardiac, liver and kidney function indices analysis
LDH and CK-MB were specific cardiac injury biomarkers. The LDH (Nanjing Jiancheng Bioengineering Research Institute, Nanjing, China) and CK-MB (ELISA kits were purchased from MDL, Beijing, China) indices of serums were evaluated by available commercial kits. In addition, changes in the activities of the AST and ALT of heart tissue homogenates were also used to evaluate the function of heart.2
AST, ALT and ALP were three hepatic-specific enzymes. The levels of these markers in serum and tissue homogenates were been assessed by available commercial kits supplied by Nanjing Jiancheng Bioengineering Research Institute, Nanjing, China, according to the manufacturer's protocol.5
The BUN and Cr levels were examined according to standard methods by using available commercial kits (Nanjing Jiancheng Bioengineering Research Institute, Nanjing, China). Nephrin is a protein necessary for the proper functioning of the renal filtration barrier.27 And nephrin was adopted to show the interaction with NPHS2.28 Besides that, TGF-β1 was also used to determine the function of renal.29 Therefore, immunohistochemical examination was used to evaluate the levels of these three markers. All of the secondary anti-rabbit antibodies were purchased from Abcam (Cambridge, UK).
2.7 Measurement of ROS
The dihydroethidium (DHE) probe was used to detect the level of intracellular ROS. The frozen sections were incubated with DHE for 30 min at 37 °C in dark. Then sections were put into decolorization shaker and washed three times with PBS. Using fluorescence microscope (Nikon Eclipse C1, Japan) and Imaging system (Nikon DS-U3) to determine the fluorescence intensity at excitation wavelength of 518 nm.
2.8 Evaluation of oxidative stress markers and antioxidant enzyme activities
In this study, cardiac, liver and renal tissue homogenates were used for evaluating antioxidant enzymes activity SOD, GPx and CAT by rat reagent kits (Nanjing Jiancheng Bioengineering Research Institute, Nanjing, China) according to the manufacturer's instructions.30,31 Oxidative stress was assessed by MDA, GSH, NO and iNOS following the instruction of rat reagent kits (Nanjing Jiancheng Bioengineering Research Institute, Nanjing, China).23
2.9 Evaluation of inflammatory markers
The concentrations of TNF-α, NF-κB and COX-2 in serum were measured by respective commercial ELISA kits (MDL, China) according to the manufacturer's protocol. For the preparation of homogenate, one gram of tissue sample was washed by ice-cold saline and wiped by filter paper. The tissue samples were put into 10 mL of 0.1 M phosphate buffered saline (pH 7.4) at 4 °C and processed by using tissue crusher at 15
000 rpm. Homogenates were centrifuged at 3000 rpm for 15 min and the supernatants were collected and used for the assessment of tissue biochemical parameters.
2.10 Western blot analysis and immunohistochemical examination
Caspase-3 is an enzyme that is one of the most important enzymes of the process of apoptosis. It is considered that BAX is one of the most important apoptosis genes which belong to the Bcl-2 gene family. And the ratio of BAX/Bcl-2 is a key factor of determining the inhibition of apoptosis. Moreover, cytochrome c is also involved in initiation of apoptosis. Therefore, immunohistochemical examination and western blot analysis were used to detect the levels of caspase-3, BAX, Bcl-2 and cytochrome c. All of the secondary anti-rabbit antibodies were purchased from Abcam, UK. Frozen heart, liver and kidney tissues were homogenized by following the manufacturer's instruction. Whole protein extract was used to detect the expression of apoptotic protein. After immunoblotting, the blot was scanned by EPSON V300 Scanner. Alpha Innotech alphaEaseFC was performed to analyze optical density. And GAPDH was used as normalization of intensity of immunoblot bands.32
2.11 Histopathological examination
After rats were terminated, cardiac, liver and kidney tissue samples were excised and immediately placed in 4% paraformaldehyde for 48 h at 25 °C. Then graded concentrations of alcohol were used to dehydrated samples. After that tissue specimens were embedded in paraffin and cut into 5 μm sections and stained with hematoxylin and eosin (H&E). The slides were analyzed by optical microscopy and the graphics were captured by a digital camera (ECLIPSE TS 100, Nikon).33
2.12 Determination of protein content
The protein content of heart, liver and renal tissue homogenates was detected by the total protein assay kit (Nanjing Jiancheng Bioengineering Research Institute, Nanjing, China) according to the manufacturer's protocol.
2.13 Statistical analysis
The statistical analyses were carried out by using the SPSS 20.0 system (Chicago, IL, USA). All data were expressed as means ± standard error of the mean (S.E.M). And different group data were analyzed by one-way analysis of variance (ANOVA). Multiple comparisons were evaluated by LSD test. The 0.05 level of probability was regarded as statistical significance. The diagrams were plotted by GraphPad Prism software (version 5).
3. Results
3.1 Analysis of chemical composition of YQFM by HPLC-Q-TOF-MS
On the basis of the retention time and mass spectra, eleven standard compounds were identified in the mixed standard solution (Fig. 1A). After analyzing the total ion integrated chromatogram (TIC) of YQFM sample solution, there were 11 active compounds found compared with TIC of standard solution, ginsenoside Rh1 was identified by MS fragmentation behaviors and retention time with reference standards (Table 1 and Fig. 1B and 2).15
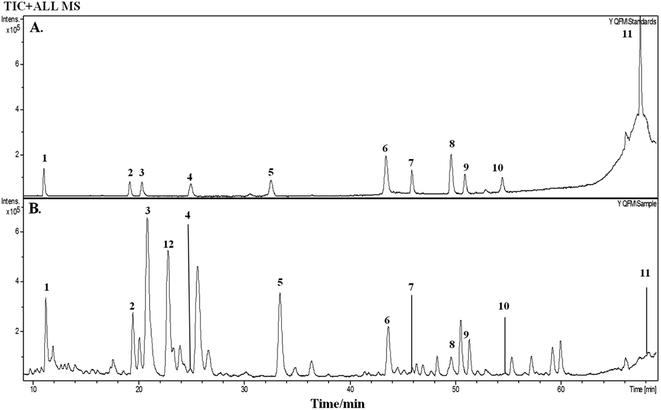 |
| Fig. 1 Total ion chromatogram (TIC) of standards and YQFM sample solution determined by HPLC-Q-TOF-MS. (A) Standard solution: peak 1: ginsenoside Rg1, peak 2: ginsenoside Rf, peak 3: ginsenoside Rb1, peak 4: ginsenoside Re, peak 5: ginsenoside Rd, peak 6: schisandrin, peak 7: 20(S)-ginsenoside F2, peak 8: schizandrol B, peak 9: 20(R)-ginsenoside Rg3, peak 10: ophiopogonin D, peak 11: schisandrin B. (B) Sample solution: peak 1: ginsenoside Rg1, peak 2: ginsenoside Rf, peak 3: ginsenoside Rb1, peak 4: ginsenoside Re, peak 5: ginsenoside Rd, peak 6: schisandrin, peak 7: 20(S)-ginsenoside F2, peak 8: schizandrol B, peak 9: 20(R)-ginsenoside Rg3, peak 10: ophiopogonin D, peak 11: schisandrin B, peak 12 ginsenoside Rh1. | |
Table 1 Chromatographic, mass spectral data and expected molecular weight of the 12 compounds of YQFM sample analyzed by HPLC-Q-TOF-MS
Peak |
tR (min) |
Identified compound |
Formula |
Theoretical m/z |
Experimental m/z |
Proposed ions |
Common fragment ions/(m/z) |
Plant source |
1 |
11.3 |
Ginsenoside Rg1 |
C42H72O14 |
823.4740 |
823.4828 |
[M + Na]+ |
823.4828, 643.4127 |
Ginseng Radix et Rhizoma Rubra |
2 |
19.5 |
Ginsenoside Rf |
C42H72O14 |
823.4730 |
823.4821 |
[M + Na]+ |
823.4821, 365.1038, 245.2083 |
3 |
20.8 |
Ginsenoside Rb1 |
C54H9O23 |
1131.5766 |
1131.5958 |
[M + Na]+ |
1131.5958, 788.5171, 365.1033 |
4 |
25.7 |
Ginsenoside Re |
C48H82O18 |
947.5212 |
947.5322 |
M+ |
947.5322, 789.9929 |
5 |
33.4 |
Ginsenoside Rd |
C48H82O18 |
969.5273 |
969.5404 |
[M + Na]+ |
969.5404, 789.4793 |
6 |
43.7 |
Schisandrin |
C24H32O7 |
455.1981 |
455.2030 |
[M + H]+ |
455.2030, 433.2210, |
Schisandrae chinensis Fructus |
7 |
46.3 |
20(S)-Ginsenoside F2 |
C42H72O13 |
785.4964 |
785.5209 |
M+ |
785.5209, 203.1752 |
Ginseng Radix et Rhizoma Rubra |
8 |
49.8 |
Schizandrol B |
C23H28O7 |
417.1854 |
417.1887 |
[M + H]+ |
417.1887, 401.1830 |
Schisandrae chinensis Fructus |
9 |
51.3 |
20(R)-Ginsenoside Rg3 |
C42H72O13 |
807.4765 |
807.4866 |
[M + Na]+ |
807.4866, 767.4841 |
Ginseng Radix et Rhizoma Rubra |
10 |
54.7 |
Ophiopogonin D |
C44H70O16 |
855.4628 |
855.3638 |
M+ |
855.3638, 287.1964 |
Ophiopogonis Radix |
11 |
69.5 |
Schisandrin B |
C23H28O6 |
401.1971 |
401.2973 |
[M + H]+ |
401.2973, 386.2923, 270.1907 |
Schisandrae chinensis Fructus |
12 |
22.8 |
Ginsenoside Rh1 |
C36H62O9 |
— |
638.2224 |
M+ |
638.2224, 531.3680, 203.1748 |
Ginseng Radix et Rhizoma Rubra |
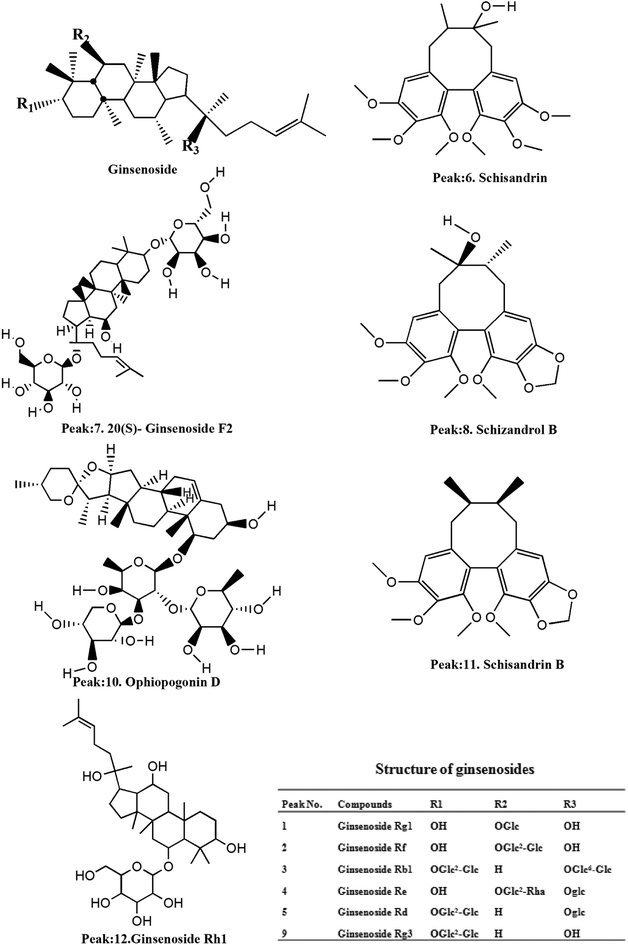 |
| Fig. 2 Structures of the compounds. | |
3.2 Effects of body weight and tissue index
That DOX caused the loss of body weight and the statistical significance was found out by comparing with the control group and YQFM treated group (0.481 g kg−1, n = 10) (Fig. 3). Although the rats of YQFM treated DOX group (0.481 g kg−1, n = 10) also lost body weight, their weight became gradually stable from 238.92 ± 13.42 g to 224.26 ± 13.52 g, during the last four days. As shown in Table 2, the heart weight and heart index in the DOX alone treated group were both significantly decreased (p < 0.05, and p < 0.001, respectively). Meanwhile the liver index and kidney index of DOX alone treated group were both significantly increased (p < 0.001), while pretreated with YQFM, the heart index, liver index and kidney index were shown a significant difference from DOX group. These results suggested that YQFM had an effect of preventing the body weight loss in DOX-induced injuries (Fig. 3).
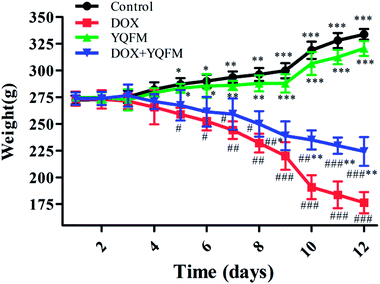 |
| Fig. 3 Effect of YQFM on DOX-induced changes in body weight of different groups. The results were presented as the mean ± SEM (n = 10). *p < 0.05, **p < 0.01, ***p < 0.001 vs. DOX group; #p < 0.05, ##p < 0.01, ###p < 0.001 vs. control group. | |
Table 2 Effects of treatment with YQFM on final heart weight, liver weight, kidney weight, and index of heart, liver and kidneya
|
Control |
DOX |
YQFM |
DOX + YQFM |
The results are presented as the mean ± standard error (n = 10); *p < 0.05, **p < 0.01, ***p < 0.001 vs. DOX treated group; #p < 0.05, ##p < 0.01, ###p < 0.001 vs. control group. |
Heart weight (g) |
1.402 ± 0.141*** |
0.688 ± 0.079### |
1.326 ± 0.107** |
0.893 ± 0.030#* |
Heart index |
0.420 ± 0.051*** |
0.390 ± 0.024### |
0.413 ± 0.016** |
0.398 ± 0.016#* |
Liver weight (g) |
10.427 ± 1.051** |
6.218 ± 0.060## |
10.339 ± 1.330** |
7.321 ± 0.280#* |
Liver index |
3.123 ± 0.213** |
3.525 ± 0.038## |
3.224 ± 0.130** |
3.283 ± 0.229* |
Kidney weight (g) |
2.566 ± 0.041*** |
1.801 ± 0.099### |
2.447 ± 0.137*** |
1.997 ± 0.228##* |
Kidney index |
0.768 ± 0.013*** |
1.021 ± 0.034### |
0.763 ± 0.026*** |
0.890 ± 0.017#*** |
3.3 Echocardiography
Echocardiography results (Table 3 and Fig. 4) demonstrated that at the end of experiment, the heart rate was unaffected by DOX and similar with normal control group, YQFM group (0.481 g kg−1, n = 10) and YQFM treated DOX group (0.481 g kg−1, n = 10). The IVS, relative wall thickness (RWT), LVD in systole (LVDs) and LVD in diastole (LVDd) of DOX group increased significantly (p < 0.05 and p < 0.001, respectively). However, the results of LVPW, ejection fraction (EF) and fractional shortening (FS) of DOX group were lower than the other groups (p < 0.05 and p < 0.001, respectively).
Table 3 Echocardiograpy measurements in normal saline control group, DOX group, YQFM group and YQFM treated DOX groupa
|
Control |
DOX |
YQFM |
DOX + YQFM |
Day |
1st |
12th |
1st |
12th |
1st |
12th |
1st |
12th |
The results are presented as the mean ± standard error (n = 10); *p < 0.05, **p < 0.01, ***p < 0.001 vs. DOX treated group; #p < 0.05, ##p < 0.01, ###p < 0.001 vs. control group. |
IVS (mm) |
1.536 ± 0.25 |
1.500 ± 0.26** |
1.646 ± 0.26 |
2.204 ± 0.32## |
1.536 ± 0.13 |
1.499 ± 0.07** |
1.646 ± 0.19 |
1.658 ± 0.15* |
PW (mm) |
2.853 ± 0.34 |
2.804 ± 0.34** |
2.999 ± 0.41 |
1.829 ± 0.07## |
2.853 ± 0.40 |
2.706 ± 0.29** |
3.182 ± 0.37 |
2.280 ± 0.25#* |
RWT |
0.524 ± 0.12 |
0.523 ± 0.07** |
0.565 ± 0.08 |
0.640 ± 0.04## |
0.460 ± 0.04 |
0.478 ± 0.04*** |
0.525 ± 0.09 |
0.547 ± 0.08** |
LVDs (mm) |
3.767 ± 0.62 |
3.694 ± 0.85** |
3.675 ± 0.46 |
5.267 ± 0.56## |
4.104 ± 0.35 |
4.425 ± 0.57** |
3.657 ± 0.60 |
4.998 ± 0.67## |
LVDd (mm) |
6.656 ± 0.38 |
6.327 ± 0.60** |
6.730 ± 0.59 |
7.627 ± 0.36## |
6.715 ± 0.31 |
6.803 ± 0.56* |
6.583 ± 0.38 |
6.644 ± 0.66* |
EF (%) |
73.382 ± 8.40 |
73.626 ± 8.79*** |
75.806 ± 9.31 |
46.090 ± 12.58### |
69.612 ± 3.67 |
62.905 ± 10.17*** |
74.888 ± 7.07 |
58.581 ± 6.39###*** |
FS |
43.407 ± 4.44 |
40.932 ± 6.57*** |
45.653 ± 5.52 |
23.626 ± 9.17### |
36.998 ± 3.59 |
34.965 ± 6.63** |
44.751 ± 8.21 |
31.844 ± 4.23#* |
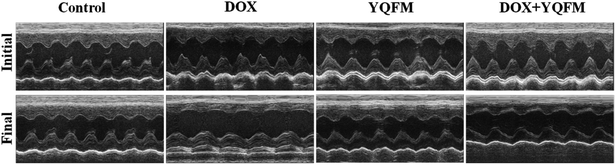 |
| Fig. 4 Echocardiographic M-mode images of different groups. | |
3.4 Effect of cardiac injury index
It was determined whether the attenuated DOX-induced toxicity by YQFM (0.481 g kg−1, n = 10) was correlated with the biochemical markers. In Fig. 5A and D the activities of AST and ALT in heart tissue homogenates were significantly increased in DOX group (p < 0.05 and p < 0.001, respectively) as compared with the YQFM treated DOX group (0.481 g kg−1, n = 10). As expected, the levels of the other two myocardial injury parameters, LDH (Fig. 5B) and CK-MB (Fig. 5C), were also significantly elevated in DOX group (p < 0.05 and p < 0.001, respectively) indicating severe damage to the myocardium. However, the pretreatment of DOX intoxicated rats with YQFM (0.481 g kg−1, n = 10) decreased the contents of LDH and CK-MB, indicating the protection of cardiac. As demonstrated by these data, pretreatment with YQFM could reduce DOX-induced cardiotoxicity.
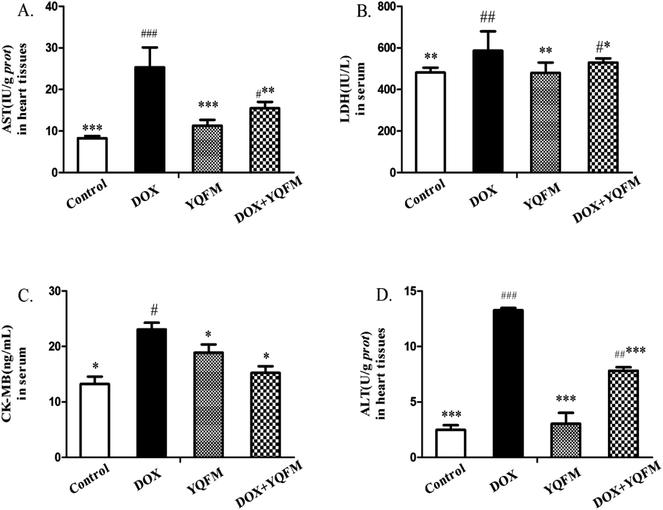 |
| Fig. 5 Effects of YQFM on DOX-induced changes in cardiac injury index. (A) AST, (B) LDH, (C) CK-MB and (D) ALT. The results were presented as the mean ± SEM (n = 10). *p < 0.05, **p < 0.01, ***p < 0.001 vs. DOX group; #p < 0.05, ##p < 0.01, ###p < 0.001 vs. control group. | |
3.5 Effects of liver function index
As shown in Fig. 6, the liver functional parameters of serum and tissue homogenates in YQFM control group showed similarly levels as normal control group. The rats exposed to DOX resulted in increasing levels of ALT (Fig. 6A and A1), AST (Fig. 6B and B1) and ALP (Fig. 6C and C1) in serum and tissue homogenates compared with the normal control group rats (p < 0.05 and p < 0.001, respectively). However, levels of these hepatic-specific enzymes in YQFM treated DOX group (0.481 g kg−1, n = 10) were shown a significant decrease comparing to the DOX group. The results indicated that YQFM could ameliorate liver injury induced by DOX.
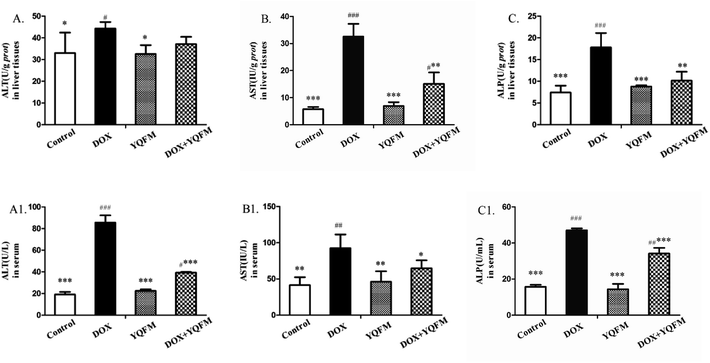 |
| Fig. 6 Effects of YQFM on DOX-induced changes in liver function index. (A) ALT, (B) AST and (C) ALP. The contents of these liver function markers in the liver tissues of each group. (A1) ALT, (B1) AST and (C1) ALP. The contents of these liver function markers in the serum of each group. The results were presented as the mean ± SEM (n = 10) *p < 0.05, **p < 0.01, ***p < 0.001 vs. DOX group; #p < 0.05, ##p < 0.01, ###p < 0.001 vs. control group. | |
3.6 Effects of kidney function index
Fig. 7A and B showed that DOX group revealed a significant increase in serum BUN and Cr amounting to 148% and 30.5%, respectively, as compared with the normal control group (p < 0.05 and p < 0.001, respectively). Moreover, to assess the function of kidney, this study measured nephrin, NPHS2 and TGF-β1 by immunohistochemistry analysis. Results were displayed in Fig. 7C, indicating that DOX led to obvious reduction in the expression of nephrin and NPHS2 in kidney. Positive staining of nephrin and NPHS2 in YQFM treated DOX group were markedly raised compared with that in the DOX group. Moreover, the results in Fig. 7C demonstrated that the DOX group intensified by the expression of TGF-β1 and the YQFM-treated DOX group showed a significant reduction.
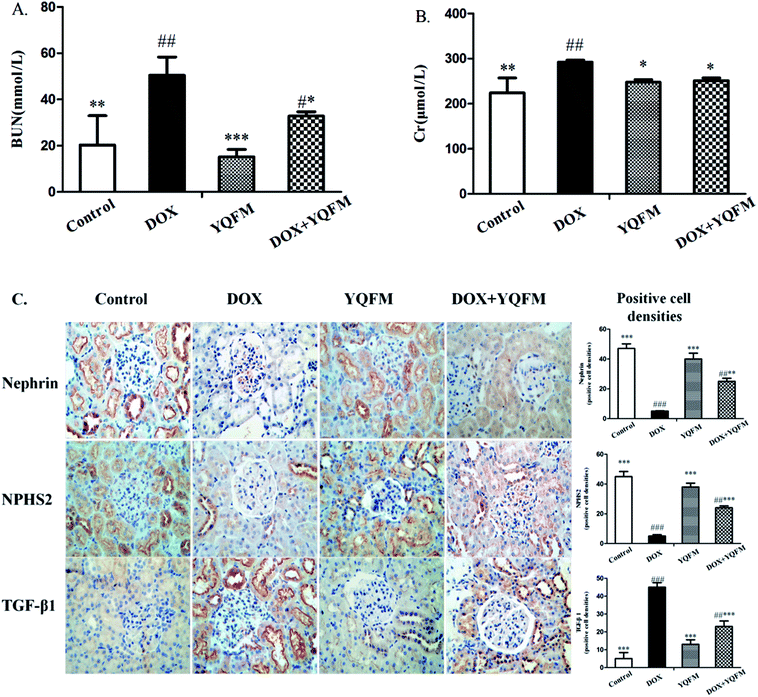 |
| Fig. 7 Effects of YQFM on DOX-induced was changed in kidney function markers. (A) BUN and (B) Cr. The content of the two kidney function markers were analyzed in the serum of each group. (C) Expression of nephrin, NPHS2 and TGF-β1 by immunohistochemical staining (400×). Quantitative image analysis for immunohistochemical staining expressed as positive cell densities across 10 different fields for each rat section. The results were presented as the mean ± SEM (n = 10) *p < 0.05, **p < 0.01, ***p < 0.001 vs. DOX group; #p < 0.05, ##p < 0.01, ###p < 0.001 vs. control group. | |
3.7 Effects of ROS and oxidative stress markers and antioxidant enzyme activities
To determine whether the YQFM reduced DOX-induced tissue injury through oxidative stress pathway, it was measured by levels of ROS, oxidative stress markers and antioxidant enzyme activities. The ROS was expressed as red fluorescence in Fig. 8, which was showed a significant increase in DOX group. On contrary, the level of ROS was markedly decreased with the treatment of YQFM as compared with DOX groups in all three kinds of tissues.
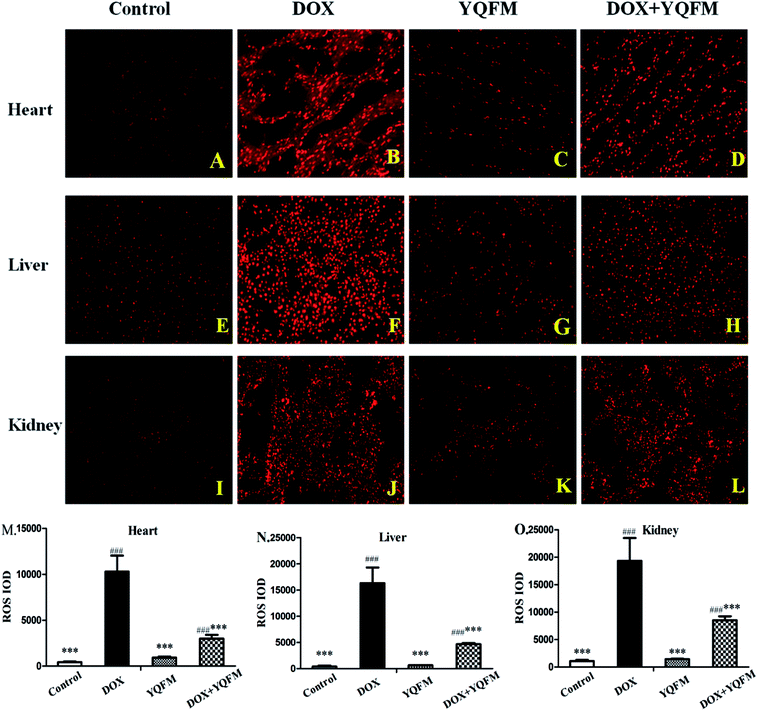 |
| Fig. 8 Effects of YQFM on DOX-induced was changed in ROS in different tissues (200×). (A) Normal saline control group of heart tissue, (B) DOX control group of heart tissue, (C) YQFM group of heart tissue, (D) YQFM treated DOX group of heart tissue. (E) Normal saline control group of liver tissue, (F) DOX control group of liver tissue, (G) YQFM group of liver tissue, (H) YQFM treated DOX group of liver tissue, (I) normal saline control group of kidney tissue, (J) DOX control group of kidney tissue, (K) YQFM group of kidney tissue, (L) YQFM treated DOX group of kidney tissue. Quantitative image analysis for immunofluorescence staining expressed as integrated optical density (IOD) 10 different fields for each rat section in different tissues. (M) IOD of ROS in heart tissue, (N) IOD of ROS in liver tissue, (O) IOD of ROS in kidney tissue. The results were showed as the mean ± SEM (n = 10) *p < 0.05, **p < 0.01, ***p < 0.001 vs. DOX group; #p < 0.05, ##p < 0.01, ###p < 0.001 vs. control group. | |
Furthermore, we examined several key antioxidants such as antioxidant enzyme GSH (Fig. 9A), GPx, (Fig. 9B), SOD (Fig. 9F) and CAT (Fig. 9G), which were specifically decreased in DOX group of the three tissues (p < 0.05 and p < 0.001, respectively). Compared to the DOX group, rats pretreated with YQFM showed a significant increase in the levels of GSH, GPx, SOD and CAT, whereas the content of MDA (Fig. 9E) was significantly decreased (p < 0.05 and p < 0.001, respectively). In addition, the level of MDA was markedly elevated in DOX group (p < 0.05), which indicated that the DOX-induced tissue damage might caused by oxidative stress. As shown in Fig. 9C and D, the rats in DOX group displayed significantly increased the contents of NO and iNOS (p < 0.05 and p < 0.001, respectively) as compared with normal control group. These findings indicated that administration of YQFM resulted in antioxidant effects in DOX-induced organ toxicity rats, as proved by activating antioxidant enzymes GPx, CAT and SOD and downregulating the levels of oxidative stress markers like MDA, NO and iNOS in heart, liver and kidney.
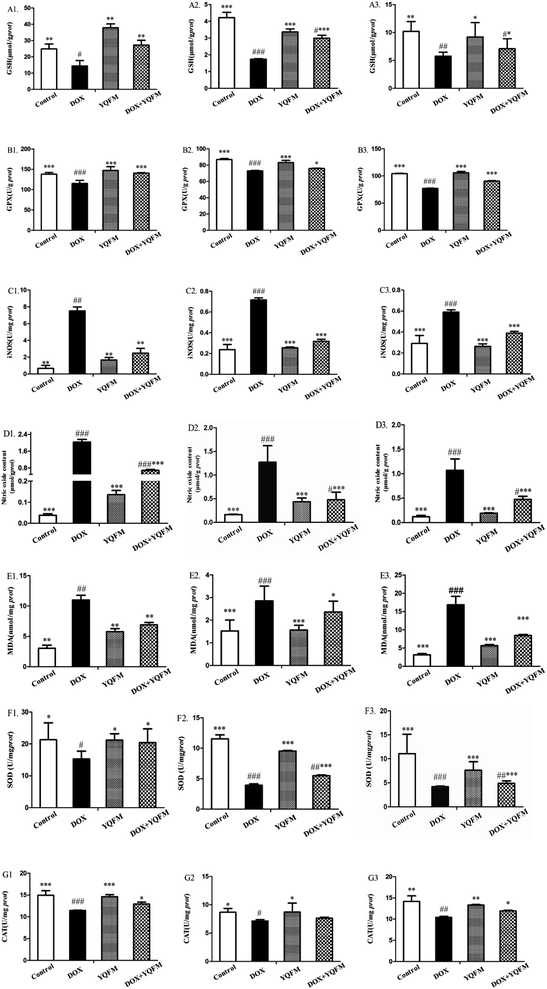 |
| Fig. 9 Effects of YQFM on DOX-induced changes were analyzed in oxidative stress markers and antioxidant enzyme activities in different tissues. (A1) GSH in heart, (A2) GSH in liver, (A3) GSH in kidney, (B1) GPx in heart, (B2) GPx in liver, (B3) GPx in kidney, (C1) iNOS in heart, (C2) iNOS in liver, (C3) iNOS in kidney, (D1) NO in heart, (D2) NO in liver, (D3) NO in kidney, (E1) MDA in heart, (E2) MDA in liver, (E3) MDA in kidney, (F1) SOD in heart, (F2) SOD in liver, (F3) SOD in kidney, (G1) CAT in heart, (G2) CAT in liver, (G3) CAT in kidney. The level of those markers in the heart tissue of control group, DOX group, YQFM group and YQFM treated DOX group. The results were showed as the mean ± SEM (n = 10) *p < 0.05, **p < 0.01, ***p < 0.001 vs. DOX group; #p < 0.05, ##p < 0.01, ###p < 0.001 vs. control group. | |
3.8 Expression of inflammatory markers
To examine whether YQFM could inhibit the inflammation that caused by DOX, we applied an immunohistochemical staining to measure the expression of proinflammatory enzyme COX-2. In rats receiving DOX alone treatment, we observed a specifically increase in the COX-2 (Fig. 10A) as shown by the intense brown staining in the myocardial, hepatic and renal tissue compared with normal control group and YQFM group. On the contrary, the pretreatment of DOX-intoxicated rats with YQFM showed anti-inflammatory effects via markedly preventing the elevation to the extent of expression of COX-2. In addition, YQFM treatment decreased the serum levels of NF-κB (Fig. 10B) and TNF-α (Fig. 10C), while DOX-induced proinflammatory response were evidenced by significant increase in NF-κB (p < 0.01) and TNF-α (p < 0.05) in the serum by 48.9% and 51.1% respectively as compared with normal control group. Treatment of rats with YQFM alone showed no significant effect on inflammatory parameters compared to the control group respectively.
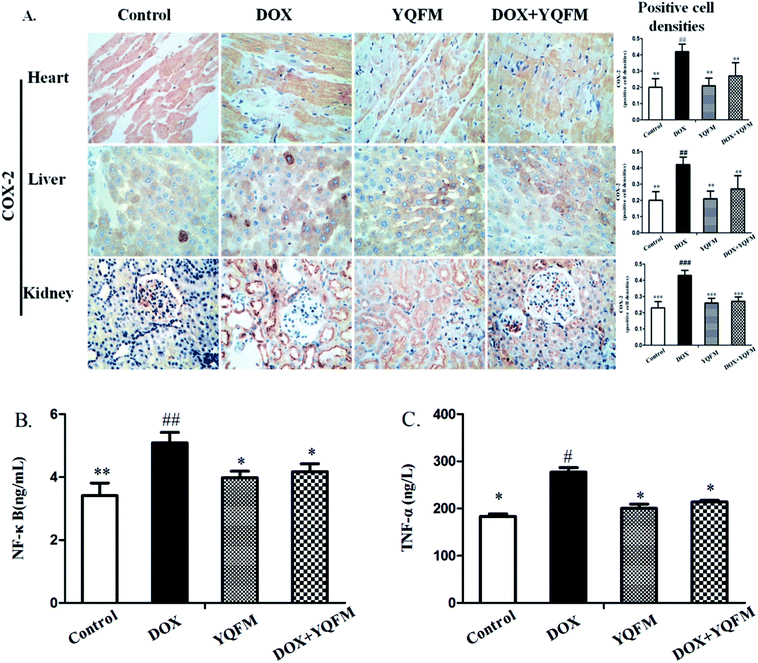 |
| Fig. 10 YQFM remarkably ameliorates the expression of inflammatory markers. (A) Expression of COX-2 was detected by immunohistochemical staining (400×). Quantitative image analysis for immunohistochemical staining expressed as positive cell densities across 10 different fields for each rat section. ELISA results of serum (B) NF-κB and (C) TNF-α. The results were shown as the mean ± SEM (n = 10) *p < 0.05, **p < 0.01 vs. DOX group; #p < 0.05, ##p < 0.01, ###p < 0.001 vs. control group. | |
3.9 Expression of apoptotic markers
The expression of apoptosis-related proteins was analyzed by immunohistochemical and immunoblot. As shown in Fig. 11, the expression levels of BAX and BAX/Bcl-2 ratio were significantly increased in the tissues of DOX alone administrated rats. Control rats showed minimal immunoblot for the proapoptotic protein BAX while intense levels of the antiapoptotic protein Bcl-2. However the immunoblot analysis also exhibited that expression of proapoptotic protein BAX and the antiapoptotic protein Bcl-2 in YQFM group were similar to the control group. Anti-apoptotic effect of YQFM pretreated group was evidenced by expressing faint immunostaining for BAX and intense immunostaining for Bcl-2.
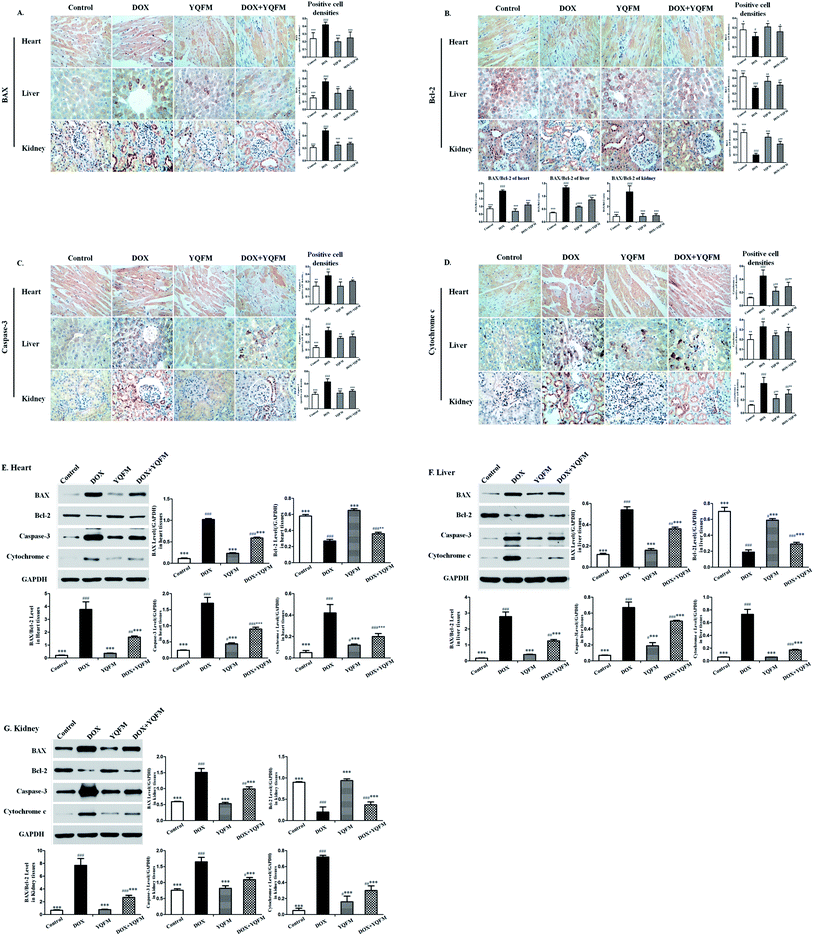 |
| Fig. 11 YQFM remarkably ameliorated the expression of apoptosis-related proteins. Expression of apoptosis-related proteins (A) BAX, (B) Bcl-2, (C) caspase-3 and (D) cytochrome c by immunohistochemical staining (400×). Quantitative image analysis for immunohistochemical staining expressed as positive cell densities ratio across 10 different fields for each rat section in different tissue. Immunoblot and bar charts showed quantitative results for the expression of BAX, Bcl-2, caspase-3 and cytochrome c in (E) heart, (F) liver and (G) kidney. The results were shown as the mean ± SEM (n = 10) *p < 0.05, **p < 0.01, ***p < 0.001 vs. DOX group; #p < 0.05, ##p < 0.01, ###p < 0.001 vs. control group. | |
Moreover the group treated with DOX alone showed significantly increased (p < 0.001) in the expression of caspase-3 in the heart, liver and kidney tissue. Meanwhile, it showed a little increased in caspase-3 of YQFM control group, due to its bidirectional regulation.34 Pretreated with YQFM could decrease the expression of caspase-3, as evidenced by the faint brown stain compared to DOX group.
In addition, apoptosis was further confirmed by assessing cytochrome c. It was found that there was significantly elevated cardiac, liver and renal cytochrome c levels in DOX group as compared with control group (p < 0.001). Contrariwise, pretreatment of YQFM modulated the expression of cytochrome c as compared with DOX alone treated group (p < 0.001). The results indicated that apoptotic damage was involved in the DOX-induced cardiotoxicity, hepatotoxicity and nephrotoxicity. YQFM pretreatment markedly prevented the DOX-induced apoptosis.
3.10 Histopathological examination
Histopathological examination of heart tissue was used to analyze the details of the cardiotoxicity induced by DOX. The rats in control group and YQFM group showed normal cardiomyocytes histoarchitecture and regular cell distribution (Fig. 12A and C). On the contrary, it was found that in DOX alone treated group, myocardial degeneration was shown as myofibrillar loss, increased intracellular gaps and vacuolization, inflammatory infiltrations, retrogressive lacerations in muscle fibres, interstitial edema and obvious capillary congestion (Fig. 12B). However, with pretreatment of the YQFM treated DOX group there showed almost regular cell distribution and normal myocardium architecture, except part of myofibrillar loss and capillary congestion (Fig. 12D).
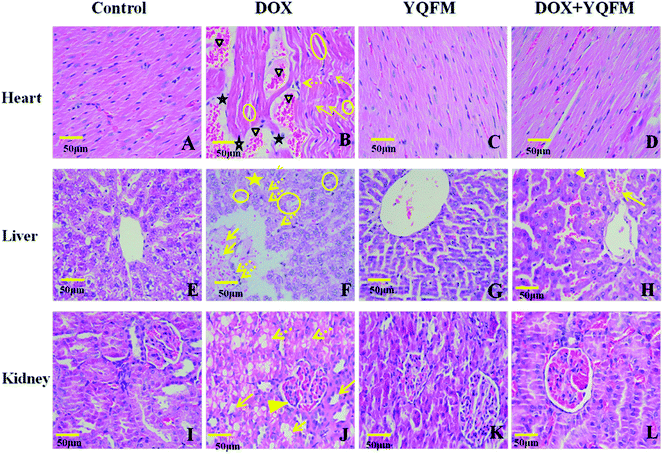 |
| Fig. 12 Micrograph of haematoxylin and eosin stained sections of heart, liver and kidney tissue depicting (400×). (A) Normal saline (0.4 mL kg−1, i.p.) control group (n = 10) of heart tissue, (B) DOX control group (DOX, n = 10) of heart tissue, showing DOX-induced myofibrillar loss (arrows), inflammatory cell infiltration (dotted arrows), cytoplasmic vacuolization (circles), edema (star), and congestion (solid triangle). (C) YQFM (0.481 g kg−1, i.p. n = 10) group of heart tissue, (D) YQFM (0.481 g kg−1, i.p.) treated DOX group (n = 10) of heart tissue. (E) Normal saline (0.4 mL kg−1, i.p) control group (n = 10) of liver tissue, showing normal polyhedral hepatic cells and clear hepatic cords, (F) DOX control group (DOX, n = 10) of liver tissue, indicating DOX-caused severe congestion of portal blood vessels (arrows), necrosis of hepatocytes (star), hepatic cell which showed obscure and fiberized boundary and inflammatory cell infiltration (dotted arrows), and also showing diffuse vacuolar degeneration and nuclear pyknosis (circles). (G) YQFM (0.481 g kg−1, i.p. n = 10) group of liver tissue, showing the same structure as normal saline control group. (H) YQFM (0.481 g kg−1, i.p.) treated DOX group (n = 10) of liver tissue, showing some diffuse vacuolar degeneration and nuclear pyknosis (solid triangle) and mild portal vein congestion (arrow). (I) Normal saline (0.4 mL kg−1, i.p.) control group (n = 10) of kidney tissue, (J) DOX control group (DOX, n = 10) of kidney tissue, displaying significant oedema and vacuolation, hydropic degeneration (arrows) and necrosis atrophy (dotted arrows) in epithelial cells of the proximal and distal tubules, and also showing glomerular atrophy (solid triangle). (K) YQFM (0.481 g kg−1, i.p. n = 10) group of kidney tissue, (L) YQFM (0.481 g kg−1, i.p.) treated DOX group (n = 10) of kidney tissue. Bar = 50 μm. | |
To further characterize the hepatotoxicity induced by DOX histopathological examination of liver tissue was represented in Fig. 12E–H. Control group and YQFM group were in normal histological appearance (Fig. 12E and G). The liver section of DOX-intoxicated (Fig. 12F) displayed severe congestion of portal blood vessels, necrosis of hepatocytes, which showed obscure and fiberized boundary and inflammatory cell infiltration and indicated diffuse vacuolar degeneration and nuclear pyknosis. On the contrary, histological examination of livers from the YQFM treated DOX group (Fig. 12H) revealed less diffuse vacuolar degeneration and nuclear pyknosis and mild portal vein congestion, which indicated that pretreated with YQFM could reverse DOX-mediated toxic changes in liver tissue.
Histological changes in the rat kidneys administered with DOX alone or with YQFM were presented in Fig. 12I–L. There were no pathological damage observed in kidney sections from control group (Fig. 12I) and YQFM control group (Fig. 12K). In contrast, DOX-induced renal toxicity group (Fig. 12J) had significant oedema and vacuolation, hydropic degeneration and necrosis atrophy in epithelial cells of the proximal and distal tubules within glomerular atrophy. On the contrary, DOX co-administration with YQFM specifically attenuated the renal damage (Fig. 12L).
4. Discussion
The findings of present study showed that the effectiveness of the YQFM in ameliorating DOX-induced cardiotoxicity, hepatotoxicity and nephrotoxicity in rats. In this research, higher pathological damage of cardiac, liver and kidney in DOX administered group successfully caused by oxidative stress, inflammation and apoptosis. DOX-induced toxicity was assessed by echocardiography, cardiac function indices (LDH, CK-MB, AST and ALT) analysis, liver function indexes (AST, ALT and ALP), nephrotoxicity markers (BUN, Cr, nephrin, NPHS2 and TGF-β1) and histopathological examination. The results demonstrated that with pretreatment of YQFM rapid activation of antioxidant enzyme like SOD, GPx and CAT involved in the inhibition of DOX-induced toxicity by preventing ROS accumulation in cardiac, liver and kidney. What's more, YQFM attenuated DOX-induced toxicities was also dependent on its anti-inflammatory effects and antiapoptosis by decreasing levels of inflammatory biomarkers and downregulating apoptotic proteins. Therefore, present study proved that YQFM exhibited effects of decreasing DOX-induced cardiotoxicity, hepatotoxicity and nephrotoxicity, and its possible mechanism might associate with anti-oxidative stress, anti-inflammatory and anti-apoptosis activities.
Twelve active substances ginsenoside Rg1, Rf, Rb1, Re, Rd, Rh1, 20(S)-ginsenoside F2, 20(R)-ginsenoside Rg3, schisandrin, schizandrol B, schisandrin B and ophiopogonin D were identified from YQFM by HPLC-Q-TOF-MS, which have been proved the protection of heart, liver and kidney. For instance, ginsenoside Rg3 and Rg1 are powerful free radical scavengers and have tissue protection effects by increasing the ratio of Bcl-2 and BAX and preventing the cardiac toxicity of DOX.17 Ophiopogonin D is shown an effect of decreasing the generation of ROS due to mitochondrial damage.35 In addition, schisandrin is also reported to inhibit cell apoptosis, reduce reactive oxygen species and decrease LDH leakage.36 Ginsenoside Rg1 and other ginsenosides have the protection of liver and kidney via interfering with oxidative stress, inflammation and apoptosis.37,38
There are various adverse effects caused by DOX, such as body weight loss, heart index decreased and cardiac dysfunction, restricting its therapeutic usage. In our study, increase of PW, EF and FS were observed following pretreatment of YQFM compared with DOX group, indicating an amelioration effect of YQFM in cardiac function by preventing myocardial structure change. DOX-induced cardiotoxicity was usually caused by oxidative stress, which was attributed to the rich content of mitochondria in heart tissues.39 When cationic form DOX is combined with cardiolipin into a nearly-irreversible complex and reserved in the mitochondrial inner membrane, the combination may ruin the cardiolipin–protein interface which can affect mitochondrial function.40 YQFM has a powerful antioxidant activity which can remove lipid peroxide MDA to protect mitochondrial. Furthermore, apart from mitochondrial damage that depended on ROS, the oxidative stress is also induced by the interaction between NO synthases and DOX.41 The endogenous antioxidants like GPx and GSH are both decreased by DOX, while YQFM apparently increases the activities of those antioxidant enzymes, indicating YQFM activates antioxidant activity to reduce the damage of oxidative stress. Besides that, promoting the release of pro-inflammatory cytokines can also contribute to DOX-induced cardiotoxicity.42 As an important mediator of inflammation, NF-κB regulates the release of some other proinflammatory cytokines such as TNF-α, COX-2 and NO.43 This process possibly leads to profound pathological changes in the form of cardiomyopathy and transmural myocarditis.44,45 On the other hand, pretreatment of YQFM significantly downregulated these inflammatory factors levels. Moreover, as a common feature of DOX-induced cardiotoxicity, myocardial apoptosis has previously been demonstrated and it also has been proved by our present study.46 The proteins of Bcl-2 family are major regulators of apoptosis, including pro-apoptotic like BAX and anti-apoptotic like Bcl-2. BAX increases oxidative stress and helps induce cytochrome c to be released from mitochondria to cytosol. Contrary to BAX, Bcl-2 is a kind of protein that is related to outer mitochondrial membrane and restrained the release of cytochrome c.47 And the loss of mitochondrial membrane potential, which is caused by ROS, can also increase the secretion of cytochrome c.48 Then cytochrome c combines with apoptosis protease activation and motivates caspase-3 to induce the apoptotic degradation phase. Our results suggested (Fig. 10) that YQFM inhibited the myocardial injury not only via weakening oxidative stress and inflammation pathways but also through suppressing apoptosis. In our study, the expression of BAX, caspase-3 and cytochrome c were quite attenuated by the pretreatment of YQFM.
As for liver, the current research indicated that pretreatment of YQFM dramatically ameliorated DOX-induced hepatotoxicity as confirmed by analyzing hepatic-specific markers (ALT, AST and ALP) and histopathological examination. ROS is one of the most important factors of DOX-induced hepatotoxicity, which is also demonstrated by our result.49 Increasing production of ROS leads to an imbalance in the oxygen normal metabolism, which can induce apoptosis in the hepatocytes.50 And our research showed that there were a specifically enhance in the levels of GSH, GPx, SOD and CAT activity, while the lipid peroxide MDA was decreased due to the pretreatment of YQFM, which proved that pretreatment with YQFM was a good way to protect liver from ROS attack. Ginsenoside Rg3 has been reported an effect of preventing normal tissue injury by scavenging ROS, which explained part of reasons that YQFM could reduce DOX-induced liver injury.51 In present study, the results indicated that DOX induced hepatotoxicity via the expression of inflammatory and apoptotic markers.52 Evidence showed that DOX could inhibit the content of Bcl-2 in liver, and then promoted the release of cytochrome c. Moreover, cytochrome c activates the downstream apoptotic signaling like caspase-3 and leads to the apoptosis.53 Due to our study, YQFM reduced the expression of apoptosis BAX/Bcl-2 ratio, caspase-3 and cytochrome c in damaged-liver, demonstrating the protection to DOX-induced toxicity through inhibiting the release of apoptotic proteins.
Free radical generation, inflammation and apoptosis in renal tissues are incriminated to the main pathways responsible for DOX-induced nephrotoxicity.54 Adding an electron to the quinone moiety of DOX produces a semiquinone form which is usually reacting with molecular oxygen to produce ROS and leads to nephrotoxicity.55 The DOX-induced nephrotoxicity manifests itself through a significant increased of MDA showing excessive lipid peroxidation.10,56 Meanwhile, renal antioxidant enzymes activities were significantly increased by YQFM, which means the decreasing of MDA and hydroxyl radicals could mitigate mitochondrial damage and relieve kidney injury. Due to accumulative effect of ROS, the results of DOX-induced renal injury showed an increase in BUN, Cr and TGF-β1 with a significant decrease in the expression of nephrin and NPHS2, and obvious changes on renal histopathological. On the contrary, in our study, the recline of all organ injury index levels, the histological improvement and restoration of structure and kidney function demonstrated the treatment with YQFM in DOX-induced nephrotoxicity. Moreover, as an important enzyme used to produce NO, iNOS is significantly upregulated in the DOX-induced toxicity renal tissue. Whereupon, preventing the over production of NO to reduce producing of peroxynitrite can also be a possible mechanism for YQFM in treating DOX-induced nephrotoxicity.10,57 During present research, YQFM attenuated the amount of COX-2, NF-κB and TNF-α, which proved that reducing inflammatory factors was one of important reasons for the treatment of DOX-induced nephrotoxicity. Researchers demonstrated that elevation of apoptosis-related protein like BAX, caspase-3 and cytochrome c in DOX-intoxicated rats might be contributed to renal dysfunction.54,58 By activating caspase-3 in renal proximal tubular cells and causing mitochondrial pathway, DOX probably leads apoptosis, which can arise renal cell damage and disorganized renal histology.59 In present study, under the pretreatment of YQFM, expressions of BAX/Bcl-2 ratio, caspase-3 and cytochrome c were quite reduced, and then the inhibition of apoptosis could prevent tissue lesions and dysfunction, which was associated to the effect of ginsenosides included in YQFM.38,60
5. Conclusion
In our current study, it proved that YQFM had heart, liver and kidney protection by preventing DOX-induced toxicity of tissue damage. DOX and its semiquinone formation exposure could induce cardiotoxicity, hepatotoxicity and nephrotoxicity, which mainly caused structural damage and dysfunction of the key tissues. According to the results, YQFM might be a useful injection to treat DOX-induced tissue injury, as it could recover the function of heart, liver and renal and improve the cell viability via repairing the activity of antioxidant enzymes, downregulating the contents of inflammatory markers and preventing the expression of apoptosis-related proteins. The effects of YQFM may depend on its contained active substances which have ability to scavenge ROS, reduce the release of inflammatory factors and inhibit the expression of apoptotic markers (Fig. 13). Therefore, our results may help develop a new insight of YQFM and provide a new therapeutic schedule for the using of DOX in clinical.
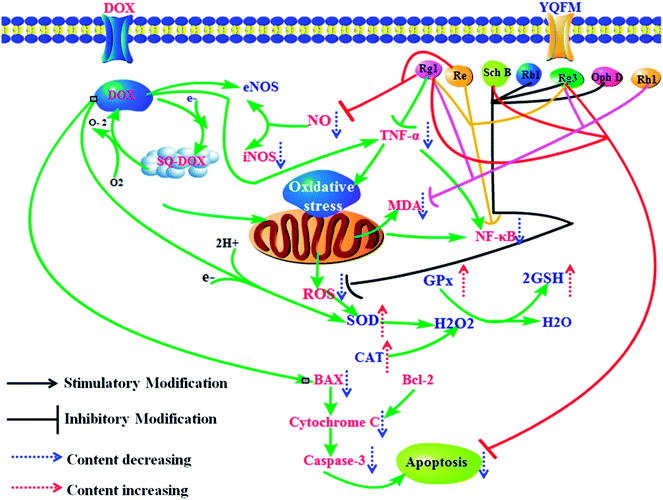 |
| Fig. 13 Potential mechanism of YQFM reduced DOX-induced heart, liver and kidney toxicity via preventing inflammation, oxidative stress, and apoptosis. | |
Conflicts of interest
The authors announce they have no conflicts of interests.
Abbreviation
ALP | Alkaline phosphatase |
ALT | Alanine aminotransferase |
AST | Aspartate aminotransferase |
BUN | Blood urea nitrogen |
CAT | Catalase |
CK-MB | Creatine kinase isoenzyme-MB |
COX-2 | Cyclooxygenase-2 |
Cr | Creatinine |
DHE | Dihydroethidium |
DOX | Doxorubicin hydrochloride |
EF | Ejection fraction |
FS | Fractional shortening |
GPx | Glutathione peroxidase |
GSH | Reduced glutathione |
H&E | Hematoxylin and eosin |
HPLC | High performance liquid chromatography |
iNOS | Inducible nitric oxide synthase |
LDH | Lactate dehydrogenase |
i.p. | Intraperitoneal injection |
IVS | Interventricular septal thickness |
LVDd | Left ventricular diameter in diastole |
LVDs | Left ventricular diameter in systole |
LVPW | Left ventricular posterior wall thickness |
MDA | Malondialdehyde |
NADPH– | Nicotinamide adenine dinucleotide phosphate– |
NF-κB | Nuclear factor κB |
NO | Nitric oxide |
PBS | Phosphate buffer saline |
ROS | Reactive oxygen species |
S.E.M | Standard error of the mean |
SOD | Superoxide |
SPE | Solid-phase extraction |
TCM | Traditional Chinese medicine |
TGF-β1 | Transforming growth factor β1 |
TIC | Total ion integrated chromatogram |
YQFM | Yiqi Fumai lyophilized injection |
Acknowledgements
This work was supported by the Science and Technology Support Program Foundation of Tianjin, China (15ZCZDSY01020) and National Natural Science Foundation of China Youth Fund Project (No. 81503467).
References
- V. K. Sonawane, U. B. Mahajan, S. D. Shinde, S. Chatterjee, S. S. Chaudhari, H. A. Bhangale, S. Ojha, S. N. Goyal, C. N. Kundu and C. R. Patil, Cardiovasc. Toxicol., 2018, 18, 1–12 CrossRef PubMed.
- T. Afsar, S. Razak, K. M. Batoo and M. R. Khan, BMC Complementary Altern. Med., 2017, 17, 554 CrossRef PubMed.
- K. Chatterjee and J. Zhang, Ann. Intern. Med., 1978, 115, 155–162 Search PubMed.
- X. Yi, J. Zhu, J. Zhang, Y. Gao, Z. Chen, S. Lu, Z. Cai, Y. Hong and Y. Wu, J. Pharm. Biomed. Anal., 2018, 152, 264–270 CrossRef CAS PubMed.
- A. M. Kabel, A. A. Alzahrani, N. M. Bawazir, R. O. Khawtani and H. H. Arab, J. Infect. Chemother., 2018, 8, 623–631 CrossRef PubMed.
- T. O. Omobowale, A. A. Oyagbemi, U. E. Ajufo, O. A. Adejumobi, O. E. Ola-Davies, A. A. Adedapo and M. A. Yakubu, J. Diet. Suppl., 2018, 15, 183–196 CrossRef CAS PubMed.
- T. O. Omobowale, A. A. Oyagbemi, U. E. Ajufo, O. A. Adejumobi, O. E. Oladavies, A. A. Adedapo and M. A. Yakubu, J. Diet. Suppl., 2017, 15, 1–14 Search PubMed.
- B. M. Barakat, H. I. Ahmed, H. I. Bahr and A. M. Elbahaie, Oxid. Med. Cell. Longevity, 2018, 2018, 8296451 Search PubMed.
- K. Nagai, S. Fukuno, K. Otani, Y. Nagamine, S. Omotani, Y. Hatsuda, M. Myotoku and H. Konishi, Pharmacology, 2018, 101, 219–224 CrossRef CAS PubMed.
- F. Benzer, F. M. Kandemir, S. Kucukler, S. Comakli and C. Caglayan, Arch. Physiol. Biochem., 2018, 1, 1–10 Search PubMed.
- Y. Xu, Y. Wang, G. Wang, X. Ye, J. Zhang, G. Cao, Y. Zhao, Z. Gao, Y. Zhang and B. Yu, Oxid. Med. Cell. Longevity, 2017, 2017, 1–17 Search PubMed.
- Q. Zhang, B. Wang, W. Liu and Y. Deng, Chinese Journal of Integrative Medicine on Cardio-/Cerebrovascular Disease, 2016, 8, 825–829 Search PubMed.
- Y. Zhao, Y. Li, L. Tong, X. Liang, H. Zhang, L. Li, G. Fan and Y. Wang, Front. Physiol., 2018, 9, 48 CrossRef PubMed.
- Q. Yuan, W. Jing, Q. H. Fang, Y. Y. Liu, J. Y. Fan, S. W. Zhang and Y. M. Ma, J. Inflammation, 2011, 8, 10 CrossRef PubMed.
- C. Liu, A. Ju, D. Zhou, D. Li, J. Kou, B. Yu and J. Qi, Molecules, 2016, 21, 640 CrossRef PubMed.
- J. T. Xie, Z. H. Shao, T. L. Vanden Hoek, W. T. Chang, J. Li, S. Mehendale, C. Z. Wang, C. W. Hsu, L. B. Becker, J. J. Yin and C. S. Yuan, Eur. J. Pharmacol., 2006, 532, 201–207 CrossRef CAS.
- L. Li, J. Ni, M. Li, J. Chen, L. Han, Y. Zhu, D. Kong, J. Mao, Y. Wang and B. Zhang, Drug Delivery, 2017, 24, 1617–1630 CrossRef CAS.
- C. Zhu, Y. Wang, H. Liu, H. Mu, Y. Lu, J. Zhang and J. Huang, Oncotarget, 2017, 8, 83792–83801 Search PubMed.
- Y. Gai, Z. Ma, X. Yu, S. Qu and D. Sui, Toxicol. Mech. Methods, 2012, 22, 584–591 CrossRef CAS PubMed.
- J. Li, Z. H. Shao, J. T. Xie, C. Z. Wang, S. Ramachandran, J. J. Yin, H. Aung, C. Q. Li, G. Qin, T. Vanden Hoek and C. S. Yuan, Arch. Pharmacal Res., 2012, 35, 1259–1267 CrossRef CAS PubMed.
- Y. D. Zhou, J. G. Hou, W. Liu, S. Ren, Y. P. Wang, R. Zhang, C. Chen, Z. Wang and W. Li, Int. Immunopharmacol., 2018, 59, 21–30 CrossRef CAS PubMed.
- H. R. Zheng, Y. Chu, D. Z. Zhou, A. C. Ju, W. Li, X. Li, Y. Xia, N. Polachi, D. K. Li and S. P. Zhou, J. Chromatogr. B: Biomed. Sci. Appl., 2017, 1072, 282–289 CrossRef.
- E. M. Mantawy, W. M. Elbakly, A. Esmat, A. M. Badr and E. Eldemerdash, Eur. J. Pharmacol., 2014, 728, 107–118 CrossRef CAS PubMed.
- J. Xiao, G. B. Sun, B. Sun, Y. Wu, L. He, X. Wang, R. C. Chen, L. Cao, X. Y. Ren and X. B. Sun, Toxicology, 2012, 292, 53 CrossRef CAS PubMed.
- A. Kumral, M. Giris, M. Soluk-Tekkesin, V. Olgac, S. Dogru-Abbasoglu, U. Turkoglu and M. Uysal, Hum. Exp. Toxicol., 2016, 35, 635–643 CrossRef CAS PubMed.
- E. Tatlidede, O. Sehirli, A. VelioäğLu-Öğünç, S. Cetinel, B. C. YeäŸEn, A. Yarat, S. SüLeymanoğLu and G. Sener, Free Radical Res., 2009, 43, 195–205 CrossRef CAS PubMed.
- R. Villarreal, A. Mitrofanova, D. Maiguel, X. Morales, J. Jeon, F. Grahammer, I. B. Leibiger, J. Guzman, A. Fachado and T. H. Yoo, J. Am. Soc. Nephrol., 2016, 27, 1029–1041 CrossRef CAS PubMed.
- M. Tabatabaeifar, T. Wlodkowski, I. Simic, H. Denc, G. Mollet, S. Weber, J. J. Moyers, B. Brühl, M. J. Randles and R. Lennon, PLoS One, 2017, 12, e0186574 CrossRef PubMed.
- M. Abbate, C. Zoja, M. Morigi, D. Rottoli, S. Angioletti, S. Tomasoni, C. Zanchi, L. Longaretti, R. Donadelli and G. Remuzzi, Am. J. Pathol., 2002, 161, 2179–2193 CrossRef CAS PubMed.
- Y. F. Xin, L. L. Wan, J. L. Peng and C. Guo, Food Chem. Toxicol., 2011, 49, 259–264 CrossRef CAS PubMed.
- R. A. Shaker, S. H. Abboud, H. C. Assad and N. Hadi, BMC Pharmacol. Toxicol., 2018, 19, 3 CrossRef PubMed.
- Y. Zhang, C. Wang, J. Zhou, A. Sun, L. K. Hueckstaedt, J. Ge and J. Ren, BBA, Mol. Basis Dis., 2017, 1863, 1919–1932 CrossRef CAS PubMed.
- S. Menon, L. Lawrence, V. P. Sivaram and J. Padikkala, J. Ayurveda Integr. Med., 2018, 6, 007 Search PubMed.
- R. Wang, Y. Hu, Q. Du, L. Liu, W. Chen, X. Shen, T. Shao and Y. Wu, Pharmacol. Clin. Chin. Mater. Med., 2001, 17, 3–4 Search PubMed.
- Y. Y. Zhang, C. Meng, X. M. Zhang, C. H. Yuan, M. D. Wen, Z. Chen, D. C. Dong, Y. H. Gao, C. Liu and Z. Zhang, J. Pharmacol. Exp. Ther., 2015, 352, 166–174 CrossRef PubMed.
- F. Li, Y. S. Tan, H. L. Chen, Y. Yan, K. F. Zhai, D. P. Li, J. P. Kou and B. Y. Yu, J. Pharmacol. Sci., 2015, 129, 1–8 CrossRef CAS PubMed.
- Y. Xin, J. Wei, M. Chunhua, Y. Danhong, Z. Jianguo, C. Zongqi and B. Jian-An, Phytomedicine, 2016, 23, 583–588 CrossRef CAS PubMed.
- J. Y. Park, P. Choi, T. Kim, H. Ko, H. K. Kim, K. S. Kang and J. Ham, J. Agric. Food Chem., 2015, 63, 5964–5969 CrossRef CAS PubMed.
- J. Yu, C. Wang, Q. Kong, X. Wu, J. J. Lu and X. Chen, Phytomedicine, 2018, 40, 125–139 CrossRef CAS PubMed.
- M. Schlame, D. Rua and M. L. Greenberg, Prog. Lipid Res., 2000, 39, 257–288 CrossRef CAS.
- G. Akolkar, D. da Silva Dias, P. Ayyappan, A. K. Bagchi, D. S. Jassal, V. M. C. Salemi, M. C. Irigoyen, K. De Angelis and P. K. Singal, Am. J. Physiol.: Heart Circ. Physiol., 2017, 313, H795–H809 CrossRef CAS PubMed.
- Y. P. Yuan, Z. G. Ma, X. Zhang, S. C. Xu, X. F. Zeng, Z. Yang, W. Deng and Q. Z. Tang, J. Mol. Cell. Cardiol., 2018, 114, 38–47 CrossRef CAS PubMed.
- T. A. Abd El-Aziz, R. H. Mohamed, H. F. Pasha and H. R. Abdel-Aziz, Clin. Exp. Med., 2012, 12, 233–240 CrossRef CAS PubMed.
- G. Minotti, P. Menna, E. Salvatorelli, G. Cairo and L. Gianni, Pharmacol. Rev., 2004, 56, 185–229 CrossRef CAS PubMed.
- F. Benzer, F. M. Kandemir, M. Ozkaraca, S. Kucukler and C. Caglayan, J. Biochem. Mol. Toxicol., 2018, 32, e22030 CrossRef PubMed.
- S. Kobayashi, P. Volden, D. Timm, K. Mao, X. Xu and Q. Liang, J. Biol. Chem., 2010, 285, 793–804 CrossRef CAS PubMed.
- J. Yang, X. Liu, K. Bhalla, C. N. Kim, A. M. Ibrado, J. Cai, T. Peng, D. P. Jones and X. Wang, Science, 1997, 275, 1129–1132 CrossRef CAS PubMed.
- H. N. Sabbah, Cardiovasc. Res., 2000, 45, 704–712 CrossRef CAS.
- S. Rashid, N. Ali, S. Nafees, S. T. Ahmad, W. Arjumand, S. K. Hasan and S. Sultana, Toxicol. Mech. Methods, 2013, 23, 337–345 CrossRef CAS PubMed.
- A. M. Kabel, A. A. Alzahrani, N.
M. Bawazir, R. O. Khawtani and H. H. Arab, J. Infect. Chemother., 2018, 24, 623–631 CrossRef PubMed.
- C. K. Lee, K. K. Park, A. S. Chung and W. Y. Chung, Food Chem. Toxicol., 2012, 50, 2565–2574 CrossRef CAS PubMed.
- A. M. Kabel, Biomed. Pharmacother., 2018, 97, 439–449 CrossRef CAS PubMed.
- T. K. Dua, S. Dewanjee, M. Gangopadhyay, R. Khanra, M. Zia-Ul-Haq and V. De Feo, J. Transl. Med., 2015, 13, 81 CrossRef PubMed.
- E. M. El-Sayed, A. M. Mansour and W. S. El-Sawy, J. Biochem. Mol. Toxicol., 2017, 31, e21940 CrossRef PubMed.
- N. A. El-Shitany, S. El-Haggar and K. El-desoky, Food Chem. Toxicol., 2008, 46, 2422–2428 CrossRef CAS PubMed.
- A. S. Nazmi, S. J. Ahmad, K. K. Pillai, M. Akhtar, A. Ahmad and A. K. Najmi, J. Saudi Chem. Soc., 2016, 20, S573–S578 CrossRef CAS.
- Z. Su, J. Ye, Z. Qin and X. Ding, Sci. Rep., 2015, 5, 18314 CrossRef CAS PubMed.
- M. L. Bajt, S. L. Vonderfecht and H. Jaeschke, Toxicol. Appl. Pharmacol., 2001, 175, 243–252 CrossRef CAS PubMed.
- M. H. Hassan, M. Ghobara and G. M. Abd-Allah, J. Biochem. Mol. Toxicol., 2014, 28, 337–346 CrossRef CAS PubMed.
- M. S. Han, I. H. Han, D. Lee, J. M. An, S. N. Kim, M. S. Shin, N. Yamabe, G. S. Hwang, H. H. Yoo, S. J. Choi, K. S. Kang and H. J. Jang, J. Ginseng Res., 2016, 40, 135–140 CrossRef PubMed.
Footnote |
† Electronic supplementary information (ESI) available. See DOI: 10.1039/c8ra07163b |
|
This journal is © The Royal Society of Chemistry 2018 |
Click here to see how this site uses Cookies. View our privacy policy here.