DOI:
10.1039/C8RA07140C
(Paper)
RSC Adv., 2018,
8, 35211-35217
Evaluation of xylooligosaccharide production from residual hemicelluloses of dissolving pulp by acid and enzymatic hydrolysis†
Received
27th August 2018
, Accepted 9th October 2018
First published on 15th October 2018
Abstract
Xylooligosaccharides (XOS) are useful food and pharmaceutical additives, which can be produced from various xylans. However, the XOS prepared from lignocellulosic materials are difficult to purify due to the complexity of the degradation products. Thus, hemicelluloses with a high-purity will be the preferred feedstock for XOS production. In this work, acid hydrolysis and enzymatic hydrolysis were applied to prepare XOS from the residual hemicelluloses of the dissolving pulp. The results showed that the highest XOS yield (45.18%) obtained from the acid hydrolysis was achieved with 1% sulfuric acid at 120 °C for 60 min, and xylohexaose accounted for 47% of the XOS. For enzymatic hydrolysis, under optimal conditions, the highest XOS yield of 42.96% was observed, and xylobiose and xylotriose comprised 90.5% of the XOS. It is suggested that the distribution of the XOS could be controlled significantly according to the enzymatic or acid hydrolysis conditions used.
1. Introduction
Generally, XOS are a mixture of neutral oligosaccharides made up of two to ten xylose units linked by β-1,4 bonds.1,2 XOS have various features in common, including non-toxicity, non-digestibility, low available energy, low sweetness, and so on.3 Furthermore, XOS have many biological properties, such as immunomodulatory activity, anti-cancerous activity, anti-microbial activity, blood- and skin-related effects, antiallergy properties, anti-infection properties, anti-inflammatory properties, etc.4 Previous reports showed that XOS can improve the intestinal microbial environment by increasing the number of bifidobacteria, which promote metabolism and reduce the risk of colon cancer.5,6 Additionally, XOS can effectively improve the blood ammonia levels in patients with liver cirrhosis.7 Nowadays, XOS have been widely used as ingredients for functional foods to improve bowel function, lipid metabolism and calcium absorption, prevent tooth decay, increase the presence of beneficial bacteria, etc.5,8,9
Given the importance of XOS, the production of XOS from various lignocellulosic materials by different methods has been extensively explored over the last decade.10–20 Typically, XOS can be produced from various xylans by chemical methods, enzymatic hydrolysis or a combination of chemical and enzymatic treatments.4 Álvarez et al.11 collected the hemicelluloses from the steam explosion pretreated wheat straw and further investigated the obtained hemicelluloses for XOS production by enzymatic hydrolysis. The results indicated that over 90% xylans could be transformed into XOS by using endo-xylanase and β-glucosidase. Bian et al.15 explored the production of XOS from sugarcane bagasse hemicelluloses by microwave-assisted acid hydrolysis, and the maximum XOS yield of 290.2 mg g−1 hemicelluloses was obtained with 0.24 M H2SO4 for 31 min. Yang et al.19 studied the production of XOS from corncob by a combination of acid pretreatment and enzymatic hydrolysis. The results showed that the cumulative yield of XOS after enzymatic hydrolysis was close to 67.7 g/100 g (based on xylan in raw material). However, the isolation and purification of the XOS from the complex hydrolysate of biomass containing the lignin is difficult and costly.
Dissolving pulp is a highly purified pulp with a high cellulose content (95–98%) and very low hemicelluloses and lignin content, which can be used to manufacture various cellulose-based products, such as cellulose ethers, cellulose esters, viscose fiber, etc.21–23 With the increasing demand for dissolving pulp, large quantities of chemical pulp were used to prepare dissolving pulp for viscose fiber production.22,24 Thus, large amounts of hemicelluloses were generated from the alkali extraction stage of the chemical pulp. These hemicelluloses can be easily separated from the alkali solution by acidification or membrane filtration to give hemicelluloses with a high purity and uniform structures. These high-purity hemicelluloses extracted from the dissolving pulp are promising candidate for XOS production. To date, only few studies investigated the production of XOS from the hemicelluloses of the dissolving pulp.25,26
In this work, the structural properties of the hemicelluloses extracted from the dissolving pulp were first characterized by NMR. Then, the hemicelluloses were hydrolyzed by acid and xylanase, respectively, under different conditions to obtain XOS. The XOS yields and the products distribution were comparatively evaluated during the acid hydrolysis and enzymatic hydrolysis processes. The effects of sulfuric acid concentration, hydrolysis temperature, and reaction time on the XOS yield were investigated in acid hydrolysis process. The influences of enzyme concentration and incubation time on the yield of XOS were studied in the process of enzymatic hydrolysis.
2. Materials and methods
2.1. Materials
The xylan-rich hemicelluloses extracted from the dissolving pulp were kindly provided by a viscose fiber mill (Xinjiang Fu Lida Fiber Co. Ltd, China). A typical production process for the hemicelluloses from the chemical pulp was conducted in three steps: (i) the pulp boards were soaked in aqueous alkali to dissolve the hemicelluloses, and the cellulose-rich solid was separated from the mixture; (ii) the above solution was treated by membrane filtration to recover most of the alkaline and water, resulting in concentrated hemicelluloses solutions; (iii) sulfuric acid was added to the concentrated hemicelluloses solutions to precipitate the hemicelluloses.26 Xylanase was kindly supplied by Utel biochemical Co. Ltd. Solvents and reagents were all of analytical grade and used as received.
2.2. Acid hydrolysis
The hemicelluloses (1.0 g) were suspended in different concentrations of dilute sulfuric acid solution with a solid to liquor ratio of 1
:
20 (g mL−1) in 35 mL high-pressure flask. The reaction was carried out with different acid concentrations (0.1, 0.3, 0.7, 0.9, 1.0, 1.2, 1.5, and 2.0 wt%) at moderate temperatures (70, 90, 105, 120, and 135 °C) for the scheduled times (15, 30, 45, 60, and 75 min) under stirring. As soon as the acid hydrolysis was completed, the reactant was cooled to room temperature for further analysis. The yields of xylose and XOS, including xylobiose (X2), xylotriose (X3), xylotetraose (X4), xylopentaose (X5), and xylohexaose (X6), were further detected. All experiments were performed in duplicate, and the results were averaged.
2.3. Enzymatic hydrolysis
The hemicelluloses were subjected to enzymatic hydrolysis by endo-xylanase in 250 mL conical flasks. The concentration of hemicelluloses was adjusted to 2 wt%. In order to optimize the production of XOS by the endo-xylanase, different enzymatic hydrolysis times (1, 2, 6, 8, 10, 12, 20, and 24 h) and enzyme dosages (50, 80, 120, and 150 IU g−1 substrate) were evaluated. The enzymatic hydrolysis was carried out at pH 5 and 50 °C in a 50 mM sodium acetate buffer solution in the incubator with the shaking speed of 150 rpm. At certain time intervals, samples were taken and boiled at 100 °C for 5 min to inactivate the enzyme. After that, the content of xylose and XOS in the hydrolysate was quantified.
2.4. Analytical methods
The compositions of hemicelluloses were determined according to the standard laboratory analytical procedures from the National Renewable Energy Laboratory (NREL/TP-510-42618).27 Released monosaccharide, uronic acid and oligosaccharide were quantified by high-performance anion exchange chromatography (HPAEC, Dionex ICS 3000) using a CarbopacTM PA-100 column (4 × 250 mm, Dionex) with a PA-100 guard column (4 × 50 mm, Dionex).17 The amount of hexoses and pentoses is multiplied by a constant factor of 0.9 and 0.88, respectively, to obtain the content of sugars in polymeric form. The by-products were examined using high-performance liquid chromatography (HPLC, Agilent 1200 series, US) with the method described by a previous literature.28 The molecular weights of hemicelluloses were determined by gel permeation chromatography (GPC) with a refraction index detector using a PL aquagel-OH 50 (300 × 7.7 mm) column. The column was operated at 30 °C, and eluted with 0.02 M NaCl in 0.005 M sodium phosphate buffer (pH = 7.5) at a flow rate of 0.5 mL min−1. All the hemicellulosic samples (4 mg) were dissolved in 2 mL of sodium phosphate buffer (pH = 7.5). PL pullulan polysaccharide standards (peak average molecular weights of 783, 12
200, 100
000, 1
600
000, Polymer Laboratories Ltd.) were used to calibrate the data of GPC. The solution-state NMR spectra of the samples were recorded on a Bruker NMR spectrometer (AVIII, 400 MHz, Germany) according to the previous report.29 The XOS obtained from the acid and enzymatic hydrolysis were analyzed by HPAEC according to the procedure described in a previous publication.15 The XOS and xylose yield was calculated by following equations:
3. Results and discussion
3.1. Physicochemical properties of the hemicelluloses extracted from the dissolving pulp
The determination of the physicochemical properties of the hemicelluloses is critical for the XOS production.30 The hemicelluloses extracted from the dissolving pulp were characterized using GPC (Table S1†), 1H NMR (Fig. S1†), and 13C NMR (Fig. S2†). Additionally, more detailed structural information was analyzed by the 2D-HSQC NMR technique (Fig. 1). The chemical composition of the hemicelluloses was determined by HPAEC, and the results were presented in Table 1. Clearly, the hemicelluloses were mainly composed of xylan (82.04%), and a small amount of glucose, galactose, arabinose, and glucuronic acid was also detected. The weight-average molecular weight (Mw) and polydispersity index (Mw/Mn) of hemicelluloses was 14343.5 g mol−1 and 1.54, respectively, implying that the hemicelluloses were relatively uniform polymers. The major cross-signals at 102.4/4.30, 73.6/3.19, 75.1/3.37, 75.8/3.68, 63.3/3.99, and 63.3/3.27 ppm observed in the HSQC spectrum of the hemicelluloses, are originated from the C1–H1, C2–H2, C3–H3, C4–H4, C5eq–H5eq, and C5ax–H5ax of the (1→4)-linked β-D-Xylp units, respectively.31 The results indicated that the hemicelluloses extracted from the dissolving pulp were mainly comprised of (1→4)-linked β-D-xylans.
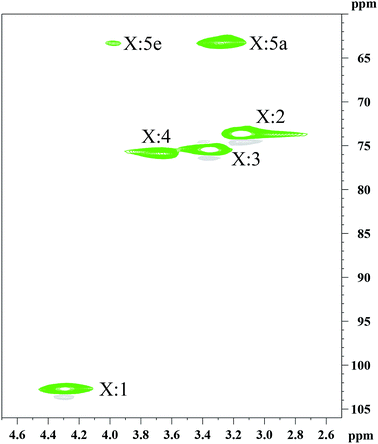 |
| Fig. 1 2D-HSQC NMR spectrum of the hemicelluloses extracted from the dissolving pulp. | |
Table 1 The chemical composition of the hemicelluloses extracted from the dissolving pulp
Composition |
Xylan |
Glucan |
Galactan |
Arabinan |
Glucuronic acid |
Ash |
Klason lignin |
Concentration (%) |
82.04 |
3.24 |
0.16 |
0.1 |
Trace |
3.26 |
1.49 |
3.2. Acid hydrolysis of the hemicelluloses for XOS production
The compositions of the hydrolysate from the hemicelluloses were determined by HPAEC. It should be noted that the content of XOS was only determined from X2 to X6 because of the lack of the XOS standard larger than X6.15,32 The effects of reaction conditions on the XOS and xylose yields during the acid hydrolysis process are presented in Fig. 2. The yields of the XOS and xylose under different sulfuric acid concentrations at 120 °C for 60 min are shown in Fig. 2a and d. It was observed that the content of X6 was much higher than other XOS when the acid concentration was lower than 1.5%. However, results from Zhang et al. showed that the X4 content was the highest one when acetic acid was used at 120 °C for 30 min.25 It suggested that the distribution of the XOS could be varied significantly with the acid used.12 The content of X4, X5, X6, and total XOS (X2 + X3 + X4 + X5 + X6) in hydrolysate increased with the increase of the sulfuric acid concentration from 0.1 to 1%, and then decreased when the acid concentration was higher than 1%, while the yields of xylose, X2, and X3 increased continuously with the increase of the acid concentration. This indicated that the hemicelluloses first degraded into XOS with relatively high DP values during the acid hydrolysis process, and then these XOS further hydrolyzed into XOS with low DP values and xylose. The degradation of the hemicelluloses and the formation of XOS and xylose are closely related to the acid concentration. The highest yield of XOS (45.18%) was achieved with 1% sulfuric acid at 120 °C for 60 min.
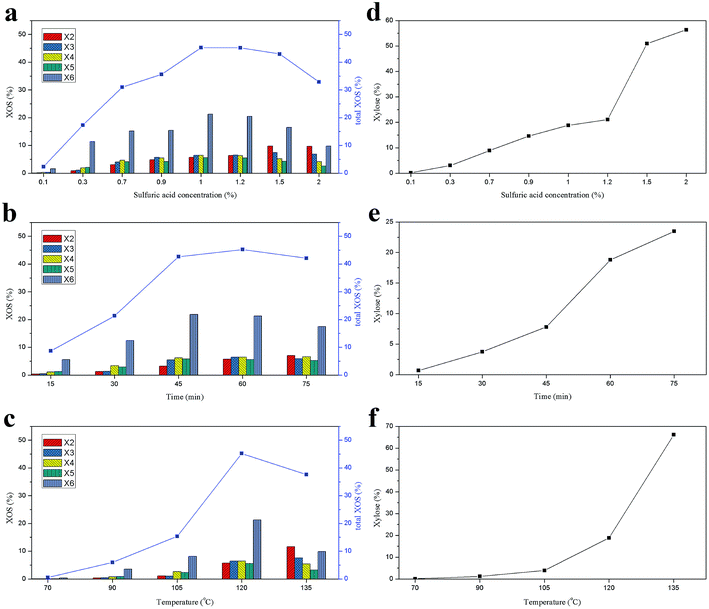 |
| Fig. 2 Effects of sulfuric acid concentration (a and d), reaction time (b and e), and reaction temperature (c and f) on the yields of XOS and xylose. | |
The effect of the reaction time of acid hydrolysis on the yields of XOS and xylose at 120 °C with 1% sulfuric acid is illustrated in Fig. 2b and e. It was found that the major component of the XOS was still X6. The total XOS yield increased gradually as the hydrolysis time increased from 15 to 60 min, but it began to decrease when the reaction time extended to 75 min. Meanwhile, similar trend was also observed for X6. However, the yield of xylose increased significantly with the prolonging of the reaction time. Obviously, a suitable reaction time was required to achieve a high yield of XOS.33 In this work, the optimal reaction time for the acid hydrolysis of hemicelluloses at 120 °C with 1% sulfuric acid was 60 min. Additionally, the influence of the reaction temperature on the XOS yield was also explored at constant acid concentration (1%) and reaction time (60 min) and the results are shown in Fig. 2c and f. It was found that when the temperature was lower than 120 °C, the yield of XOS was increased with the reaction temperature. However, when the temperature was beyond 120 °C, the total XOS yield declined from 45.18% (120 °C) to 37.61% (135 °C), and the yield of xylose dramatically increased from 18.78 to 66.13%. It suggested that a relatively high yield of XOS could be obtained at 120 °C under the given conditions. Therefore, in this work, an optimized XOS yield by acid hydrolysis could be obtained at 120 °C with 1% sulfuric acid for 60 min.
3.3. Enzymatic hydrolysis of the hemicelluloses for XOS production
Enzymatic hydrolysis is a relatively mild method for the production of mono- and oligosaccharides from polysaccharides. It has been reported that considerable yield of XOS could be obtained from lignocellulosic materials by enzymatic hydrolysis.17,34 In this work, the effects of enzyme digestion time and enzyme dosage on the production of XOS from the hemicelluloses of the dissolving pulp were investigated. The yield of the XOS under different reaction conditions is shown in Fig. 3. Overall, when the enzymatic hydrolysis was conducted at 50 IU g−1 substrate, the yield of the XOS gradually increased with the extending of the digestion time, indicating that relatively long reaction time could be used to improve the yield of XOS. However, as the enzyme concentration increased from 80 to 150 IU g−1 substrate, the XOS yield dramatically increased in the first 8 h, and then it decreased to some degree. This may result from the inhibition of the end-products to xylanase activity and the conversion of XOS to xylose.11,17,35 As shown in Fig. 3, the hydrolysis rate and yield of XOS significantly increased with the enzyme concentration varied from 50 to 120 IU g−1 substrate. Samanta and Akpinar et al.34,36 also respectively found that the xylan hydrolysis yield and rate increased with the enzyme dosage. However, the yield of XOS was almost unchanged when the enzyme concentration further increased from 120 to 150 IU g−1 substrate. Similar results had been observed by Yang et al.17 when triploid Populas tomentosa was used to prepare XOS. It was probably because the ability of enzyme protein to bind its substrate reached a plateau at a certain enzyme concentration.37 Based on the results obtained, the highest XOS yield (42.96%) from enzymatic hydrolysis was achieved at 120 IU g−1 substrate enzyme loading for 8 h.
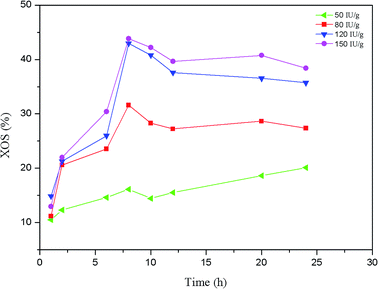 |
| Fig. 3 Effect of enzyme concentration on the yield of XOS. | |
The yields of XOS (X2–X6) and xylose as a function of reaction time under the optimal enzymatic dosage (120 IU g−1 substrate) are shown in Fig. 4. As illustrated in Fig. 4a, the XOS of the enzymatic hydrolysate was mainly composed of X2 and X3, and only a small quantity of X4, X5, and X6 was detected. Furthermore, the XOS yield gradually increased with the enzyme digestion time extended from 1 to 8 h, and the highest yield of X2 (20.32%) and X3 (18.56%) was achieved at 8 h. When the enzyme digestion time further extended, the yield of X2 and X3 decreased continually but changed slightly after 12 h. In addition, the yields of X4, X5, and X6 changed slightly, and similar results had been reported by Bian et al.38 The xylose in the hydrolysate was also detected and shown in Fig. 4b. A small amount of xylose was observed in the enzymatic hydrolysate of hemicelluloses, and its content increased significantly with the enzyme digestion time. This suggested that the xylanase employed in this work contained a large amount of endo-xylanase and few xylosidase and/or exo-xylanase.39 Most of the endo-xylanase is used to degrade of the xylan main chain to XOS.5 When the enzyme digestion time was greater than 8 h, the yield of xylose increased rapidly. This result was in accord with the decreasing of XOS yield after 8 h. Clearly, a small quantity of XOS was degraded to xylose by xylosidase.35
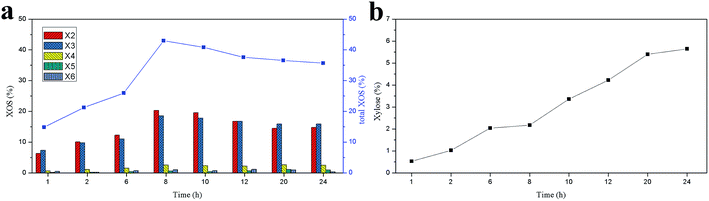 |
| Fig. 4 Effect of reaction time on the yields of XOS (a) and xylose (b) under the optimum enzyme dosage. | |
3.4. Comparison of the acid hydrolysis and enzymatic hydrolysis
For acid hydrolysis, generally, a relatively high temperature and corrosion-resistant equipment may be required due to the utilization of sulfuric acid. In contrast, the reaction condition for enzymatic hydrolysis was mild but relatively long reaction time was needed. However, the XOS yield from the acid hydrolysis and enzymatic hydrolysis was similar, and the XOS yield from acid hydrolysis (45.18%) was slightly higher than that from enzymatic hydrolysis (42.96%). Furthermore, it should be noted that the XOS from the acid hydrolysis of hemicelluloses were mainly consisted of X6, which accounted for 47% of XOS, and a quantity of X2, X3, X4, and X5 were also contained in the hydrolysate. However, X2 and X3 were the major ingredients in the enzymatic hydrolysate, which took up 47.3 and 43.25% of the XOS, respectively. Additionally, the xylose yield from the enzymatic hydrolysis was much lower than that from the acid hydrolysis. These suggested that the corresponding enzymatic hydrolysis or acid hydrolysis method can be used to obtain the optimal XOS yield according to the XOS distribution required.
4. Conclusions
The residual hemicelluloses extracted from the dissolving pulp, mainly consisted of β-D-Xylp units, were applied to produce XOS. The influences of acid hydrolysis and enzymatic hydrolysis of hemicelluloses on the production of XOS were evaluated. The acid hydrolysis performed with 1% sulfuric acid at 120 °C for 60 min produced the highest XOS yield of 45.18%. Meanwhile, a maximum XOS yield of 42.96% was obtained with 120 IU g−1 substrate for 8 h. Although the yields of XOS from the acid and enzymatic hydrolysis were similar, the main products of XOS in the hydrolysate were obviously different. The major ingredient of the XOS from acid hydrolysis was X6, while X2 and X3 were the main products of the enzymatic hydrolysis.
Conflicts of interest
The authors declare no competing interests.
Acknowledgements
This work was supported by the Fundamental Research Funds for the National Science Foundation of China for Key Project (31430092), the National Natural Science Foundation of China (31700518), National Key Research and Development Program of China (Grant no. 2017YFB0307903), and the Fundamental Research Funds for the Central Universities (2015ZCQ-CL-02).
References
- A. A. Aachary and S. G. Prapulla, Corncob-induced endo-1,4-β-d-xylanase of Aspergillus oryzae MTCC 5154: production and characterization of xylobiose from glucuronoxylan, J. Agric. Food Chem., 2008, 56, 3981–3988 CrossRef CAS PubMed.
- H. Mäkeläinen, S. Forssten, M. Saarinen, J. Stowell, N. Rautonen and A. Ouwehand, Xylo-oligosaccharides enhance the growth of bifidobacteria and Bifidobacterium lactis in a simulated colon model, Benefic. Microbes, 2010, 1, 81–91 CrossRef PubMed.
- F. Peng, P. Peng, F. Xu and R. C. Sun, Fractional purification and bioconversion of hemicelluloses, Biotechnol. Adv., 2012, 30, 879–903 CrossRef CAS PubMed.
- A. A. Aachary and S. G. Prapulla, Xylooligosaccharides (XOS) as an Emerging Prebiotic: Microbial Synthesis, Utilization, Structural Characterization, Bioactive Properties, and Applications, Compr. Rev. Food Sci. Food Saf., 2011, 10, 2–16 CrossRef CAS.
- A. F. A. Carvalho, P. d. O. Neto, D. F. da Silva and G. M. Pastore, Xylo-oligosaccharides from lignocellulosic materials: Chemical structure, health benefits and production by chemical and enzymatic hydrolysis, Food Res. Int., 2013, 51, 75–85 CrossRef CAS.
- Y. C. Chung, C. K. Hsu, C. Y. Ko and Y. C. Chan, Dietary intake of xylooligosaccharides improves the intestinal microbiota, fecal moisture, and pH value in the elderly, Nutr. Res., 2007, 27, 756–761 CrossRef CAS.
- M. Kajihara, S. Kato, M. Konishi, Y. Yamagishi, Y. Horie and H. Ishii, Xylooligosaccharide decreases blood ammonia levels in patients with liver cirrhosis, Am. J. Gastroenterol., 2000, 95, 2514 CrossRef.
- C. Grootaert, J. A. Delcour, C. M. Courtin, W. F. Broekaert, W. Verstraete and T. V. D. Wiele, Microbial metabolism and prebiotic potency of arabinoxylan oligosaccharides in the human intestine, Trends Food Sci. Technol., 2007, 18, 64–71 CrossRef CAS.
- J. Wang, X. P. Yuan, B. G. Sun, Y. P. Cao, Y. Tian and C. T. Wang, On-line separation and structural characterisation of feruloylated oligosaccharides from wheat bran using HPLC-ESI-MSn, Food Chem., 2009, 115, 1529–1541 CrossRef CAS.
- X. Xiao, C. Z. Wang, J. Bian and R. C. Sun, Optimization of bamboo autohydrolysis for the production of xylo-oligosaccharides using response surface methodology, RSC Adv., 2015, 5, 106219–106226 RSC.
- C. Álvarez, A. González, M. J. Negro, I. Ballesteros, J. M. Oliva and F. Sáez, Optimized use of hemicellulose within a biorefinery for processing high value-added xylooligosaccharides, Ind. Crops Prod., 2017, 99, 41–48 CrossRef.
- Q. Lin, H. Li, J. Ren, A. Deng, W. Li, C. Liu and R. Sun, Production of xylooligosaccharides by microwave-induced, organic acid-catalyzed hydrolysis of different xylan-type hemicelluloses: Optimization by response surface methodology, Carbohydr. Polym., 2017, 157, 214–225 CrossRef CAS PubMed.
- M. C. R. Mano, I. A. Neri-Numa, J. B. da Silva, B. N. Paulino, M. G. Pessoa and G. M. Pastore, Oligosaccharide biotechnology: an approach of prebiotic revolution on the industry, Appl. Microbiol. Biotechnol., 2018, 102, 17–37 CrossRef CAS PubMed.
- P. Vejdovszky, J. Oberlerchner, T. Zweckmair, T. Rosenau and A. Potthast, Preparation and Analysis of Cello- and Xylooligosaccharides, Cellulose Chemistry and Properties: Fibers, Nanocelluloses and Advanced Materials, Springer, Cham, 2015, pp. 53–92 Search PubMed.
- J. Bian, P. Peng, F. Peng, X. Xiao, F. Xu and R. C. Sun, Microwave-assisted acid hydrolysis to produce xylooligosaccharides from sugarcane bagasse hemicelluloses, Food Chem., 2014, 156, 7–13 CrossRef CAS PubMed.
- F. Mandelli, L. B. Brenelli, R. F. Almeida, R. Goldbeck, L. D. Wolf, Z. B. Hoffmam and F. M. Squina, Simultaneous production of xylooligosaccharides and antioxidant compounds from sugarcane
bagasse via enzymatic hydrolysis, Ind. Crops Prod., 2014, 52, 770–775 CrossRef CAS.
- H. Yang, K. Wang, X. Song and F. Xu, Production of xylooligosaccharides by xylanase from Pichia stipitis based on xylan preparation from triploid Populas tomentosa, Bioresour. Technol., 2011, 102, 7171–7176 CrossRef CAS PubMed.
- D. J. Rose and G. E. Inglett, Production of feruloylated arabinoxylooligosaccharides from maize (Zea mays) bran by microwave-assisted autohydrolysis, Food Chem., 2010, 119, 1613–1618 CrossRef CAS.
- R. Yang, S. Xu, Z. Wang and W. Yang, Aqueous extraction of corncob xylan and production of xylooligosaccharides, LWT--Food Sci. Technol., 2005, 38, 677–682 CrossRef CAS.
- M. J. Vazquez, J. L. Alonso, H. Dominguez and J. C. Parajo, Xylooligosaccharides: manufacture and applications, Trends Food Sci. Technol., 2000, 11, 387–393 CrossRef CAS.
- C. Tian, L. Q. Zheng, Q. X. Miao, C. Y. Cao and Y. H. Ni, Improving the reactivity of kraft-based dissolving pulp for viscose rayon production by mechanical treatments, Cellulose, 2014, 21, 3647–3654 CrossRef CAS.
- G. Schild and H. Sixta, Sulfur-free dissolving pulps and their application for viscose and lyocell, Cellulose, 2011, 18, 1113–1128 CrossRef CAS.
- L. P. Christov, M. Akhtar and B. A. Prior, The potential of biosulfite pulping in dissolving pulp production, Enzyme Microb. Technol., 1998, 23, 70–74 CrossRef CAS.
- D. J. Mozdyniewicz, G. Schild and H. Sixta, Alkaline steeping of dissolving pulp. Part II: Soluble compounds in the press lye, Cellulose, 2014, 21, 2889–2900 CrossRef CAS.
- H. Zhang, X. Zhou, Y. Xu and S. Yu, Production of Xylooligosaccharides from Waste Xylan, Obtained from Viscose Fiber Processing, by Selective Hydrolysis Using Concentrated Acetic Acid, J. Wood Chem. Technol., 2016, 37, 1–9 CrossRef CAS.
- Y. Zhang, G. Yu, B. Li, X. Mu, H. Peng and H. Wang, Hemicellulose isolation, characterization, and the production of xylo-oligosaccharides from the wastewater of a viscose fiber mill, Carbohydr. Polym., 2016, 141, 238–243 CrossRef CAS PubMed.
- A. Sluiter, B. Hames, R. Ruiz, C. Scarlata, J. Sluiter, D. Templeton, D. Crocker, Determination of structural carbohydrates and lignin in biomass, Laboratory Analytical Procedure, 2008, vol. 1617, pp. 1–16 Search PubMed.
- H. Yang, K. Wang, F. Xu, R. Sun and Y. Lu, H2SO4-Catalyzed Hydrothermal Pretreatment of Triploid Poplar to Enhance Enzymatic Hydrolysis, Ind. Eng. Chem. Res., 2012, 51, 11598–11604 CrossRef CAS.
- S. L. Sun, J. L. Wen, M. G. Ma and R. C. Sun, Successive alkali extraction and structural characterization of hemicelluloses from sweet sorghum stem, Carbohydr. Polym., 2013, 92, 2224–2231 CrossRef CAS PubMed.
- J. C. Parajó, G. Garrote, J. M. Cruz and H. Dominguez, Production of xylooligosaccharides by autohydrolysis of lignocellulosic materials, Trends Food Sci. Technol., 2004, 15, 115–120 CrossRef.
- S. L. Sun, J. L. Wen, M. G. Ma, X. L. Song and R. C. Sun, Integrated biorefinery based on hydrothermal and alkaline treatments: Investigation of sorghum hemicelluloses, Carbohydr. Polym., 2014, 111, 663–669 CrossRef CAS PubMed.
- P. Gullon, M. J. Gonzalezmunoz and J. C. Parajo, Manufacture and prebiotic potential of oligosaccharides derived from industrial solid wastes, Bioresour. Technol., 2011, 102, 6112–6119 CrossRef CAS PubMed.
- X. Xiao, J. Bian, X. P. Peng, H. Xu, B. Xiao and R. C. Sun, Autohydrolysis of bamboo (Dendrocalamus giganteus Munro) culm for the production of xylo-oligosaccharides, Bioresour. Technol., 2013, 138, 63–70 CrossRef CAS PubMed.
- A. K. Samanta, N. Jayapal, A. P. Kolte, S. Senani, M. Sridhar, K. P. Suresh and K. T. Sampath, Enzymatic production of xylooligosaccharides from alkali solubilized xylan of natural grass (Sehima nervosum), Bioresour. Technol., 2012, 112, 199–205 CrossRef CAS PubMed.
- W. Gong, H. Zhang, L. Tian, S. Liu, X. Wu, F. Li and L. Wang, Determination of the modes of action and synergies of xylanases by analysis of xylooligosaccharide profiles over time using fluorescence-assisted carbohydrate electrophoresis, Electrophoresis, 2016, 37, 1640–1650 CrossRef CAS PubMed.
- O. Akpinar, O. Ak, A. Kavas, U. Bakir and L. Yilmaz, Enzymatic production of xylooligosaccharides from cotton stalks, J. Agric. Food Chem., 2007, 55, 5544–5551 CrossRef CAS PubMed.
- A. B. Boraston, B. W. Mclean, G. Chen, A. Li, R. A. J. Warren and D. G. Kilburn, Co-operative binding of triplicate carbohydrate-binding modules from a thermophilic xylanase, Mol. Microbiol., 2002, 43, 187–194 CrossRef CAS PubMed.
- J. Bian, F. Peng, X. P. Peng, P. Peng, F. Xu and R. C. Sun, Structural features and antioxidant activity of xylooligosaccharides enzymatically produced from sugarcane bagasse, Bioresour. Technol., 2013, 127, 236–241 CrossRef CAS PubMed.
- M. J. Vázquez, J. L. Alonso, H. Domínguez and J. C. Parajó, Enzymatic processing of crude xylooligomer solutions obtained by autohydrolysis of eucalyptus wood, Food Biotechnol., 2002, 16, 91–105 CrossRef.
Footnote |
† Electronic supplementary information (ESI) available. See DOI: 10.1039/c8ra07140c |
|
This journal is © The Royal Society of Chemistry 2018 |