DOI:
10.1039/C8RA06928J
(Paper)
RSC Adv., 2018,
8, 41117-41130
Optimization extraction and functional properties of soluble dietary fiber from pineapple pomace obtained by shear homogenization-assisted extraction
Received
19th August 2018
, Accepted 3rd December 2018
First published on 7th December 2018
Abstract
Response surface methodology (RSM) was used to optimize the extraction conditions for shear homogenization-assisted extraction of soluble dietary fiber from pineapple pomace (s-SDF), and the absorption capacities and antioxidant activities of the obtained s-SDF were also investigated. The optimum extraction conditions consisted of a cutting speed of 9000 rpm, a cutting time of 20 min, a cellulase content of 5.0%, a hydrolysis time of 120 min, a pH value of 4.5, a hydrolysis temperature of 50 °C, and a raw material to water ratio of 1
:
45 g mL−1. Under these conditions, the theoretical and actual extraction yields of s-SDF were 8.80% and 8.76%, respectively. An absorption capacity analysis indicated that s-SDF exhibited higher absorption abilities to sodium cholate, cholesterol and fat. In addition, s-SDF possessed higher antioxidant activities, showing a positive concentration effect relationship for DPPH˙, ABTS+, ·OH and O2−˙. The concentration of 1.0 mg mL−1 scavenged 76.72% DPPH˙, 58.40% ABTS+, 23.47% ·OH and 48.47% O2−˙, respectively, and the reduction power was 0.70. These results indicated that pineapple pomace is a potential source of natural dietary fiber and a potential functional food ingredient.
1 Introduction
Pineapple (Ananas comosu), which belongs to the Bromeliaceae family, is a tropical fruit popular throughout the world due to its rich nutrients and medicinal properties, including antioxidant, anticancer and hypoglycemic activities.1 In 2013, pineapple production was around 210
000 t mainly in Latin America and the Caribbean. This fruit is eaten fresh or used to produce concentrated juices, fruits salads and jams. After processing, many thousands of tons of pomace (25–35% of the fruit weight) are generated as an agricultural by-product. Pineapple pomace contains high concentrations of dietary fiber (DF), saccharides, mineral substances and proteins, which is perishable without treatment, resulting in not only a considerable waste of resources but also environmental pollution.2,3 Therefore, studying the deep processing and utilization of this surplus waste has important practical and economic significance.
Dietary fiber (DF), a carbohydrate polymer with beneficial physiological properties, is generally derived from certain cereals, fruits, vegetables and legumes.4 Defined as the edible portion of plants or carbohydrate analogs, DF is resistant to digestion and absorption in the human small intestine, while it can be partially or completely fermented in the large intestine.5 Recently, DF has received more and more attention for its low cost and beneficial physiological effects on human health,6 including reduction of the risk of diabetes, carcinoma of colon, arteriosclerosis, obesity, and cardiovascular disease, etc.7–12 Based on its water solubility, DF can be largely divided into insoluble dietary fiber (IDF) and soluble dietary fiber (SDF).13 Beneficial effects of SDF include adsorbing heavy metal, bile acids and cholesterol, reducing the postprandial blood glucose levels, improving the intestinal microflora, and preventing diabetes and other cardiovascular diseases. While cellulose, lignin and insoluble hemicelluloses were the main components of the IDF with high water swelling capacity and water holding capacity enhances the body's satiety and increases fecal bulk, thereby reducing the risk of obesity, colon cancer and constipation.14 Compared with IDF, SDF not only exhibits more physicochemical and functional properties, but also possesses a higher viscosity and provides better texture and taste, hence, it is easier to applying in food processing. However, the resources shortage of SDF increases the cost of products production and restricts the development of industry chain. Therefore, searching for SDF from agricultural by-products is an important technical research issue in the development of food industry.
The extraction method is an important factor for the uses of plant active components. Processing conditions change the composition and structure of SDF, which, in turn, lead to desirable and undesirable effects on their physicochemical and functional properties. Nowadays, chemicals, enzymatic, thermal, fermentation and mechanical methods have been widely used to extract SDF from pineapple pomace,15–17 but some of these methods have many drawbacks such as long reaction time for chemical treatment, great solvent consumption, high requirements of temperature, and introduction of a large number of ions during the reaction process.5 Advances in processing technology have provided alternative treatments such as homogenization and extrusion to obtain SDF, resulting in low energy consumption, environmentally friendly and economically viable ways of utilizing by-products.5 The functional properties of dietary fiber was related to its particle size. Generally, a smaller particle size corresponds to larger surface area and higher yields, which can be achieved by homogenization. Shear homogenization-assisted extraction method used a mixture of homogenization and hydrolysis to obtain highly bioactive SDF. So far very few studies have been performed about the application of the shear homogenization-assisted extraction technique to extract dietary fiber from pineapple pomace.
In this study, shear homogenization-assistant cellulase extraction method was applied to extract s-SDF from pineapple pomace, and the extraction conditions were optimized by Box–Behnken design based on single-factor experiments. Then, we compared the properties [cholesterol binding capacity (CBC), sodium cholate binding capacity (SCBC), nitrite ion absorption capacity (NIAC), oil absorption capacity (OAC) and glucose absorption capacity (GAC)] of s-SDF with those of u-SDF (ultrasonic-assisted extraction method), a-SDF (acidic extraction method) and c-SDF (cellulase-assisted alkaline extraction method). Furthermore, the potential antioxidant activities of s-SDF were also investigated. These results can provide a technical reference for the efficient utilization of pineapple pomace.
2 Materials and methods
2.1 Materials
Ripened pineapple fruits were purchased from a commercial orchards located in Xuwen country (Guangdong, China). α-Amylase, neutral protease and cellulase R-10 were bought in Novozymes Biotechnology Co., Ltd. (Copenhagen, Danish). 1,1-Diphenyl-2-picrylhydrazyl (DPPH), glucose, cholesterol and sodium cholate were purchased from Sigma Aldrich Chemical Co. (Milwaukee, WI, USA). All other reagents were of analytical grade.
2.2 Preparation of SDF
2.2.1 Pretreatment of pineapple pomace. The pineapple fruits were separated into edible and inedible portions (pomace, stem and leaves), and the pomace was collected and dried in an air-oven at 50 °C for 36 h, smashed in a blender, and then sieved at 60 mesh. The dried samples were immersed in petroleum ether for 2 h to remove some lipids and colors. The residues were dried at room temperature (RT) and stored in a desiccator.
2.2.2 Shear homogenization-assisted extraction method (s-SDF). The pretreated powder (2.0 g) was thoroughly dispersed in distilled water, 3.0% (w/w) of α-amylase was added and hydrolyzed at 65 °C with constant stirring for 1 h. After the temperature of the hydrolysate was cooled down to 50 °C, 0.2% (w/w) of neutral protease was added and incubated for 40 min. And then the reaction solution was homogenised, adjusted the required pH and mixed with cellulase to continue to hydrolyze in a water bath. Afterwards, the mixture was quenched at 95 °C for 5 min and filtered after cooled down to RT. The filtrate was vacuum condensed and precipitated with 4 volumes of 95% (v/v) ethanol at 4 °C for 12 h. The sediment was collected, washed with 95% ethanol for 2–3 times and freeze–dried to obtain soluble dietary fiber (s-SDF). Univariate experiments of shear homogenization-assisted extraction of s-SDF from pineapple pomace were shown in Table 1, and the extraction yield of s-SDF was calculated by the following formula: |
 | (1) |
where W1 and W were the weight of s-SDF and dried powder of pineapple pomace, respectively.
Table 1 Univariate experiments of homogenization-assisted extraction of s-SDF from pineapple pomace
Extraction factors |
Level of factors |
Extraction conditions |
Cutting speed (rpm) |
5000, 6000, 7000, 8000, 9000, 10 000 |
10 min, 5.0% cellulase, pH 5.0, 55 °C, 2 h, 1 : 40 g mL−1 |
Cutting time (min) |
10, 20, 30, 40, 50, 60 |
9000 rpm, 5.0% cellulase, pH 5.0, 55 °C, 120 min, 1 : 40 g mL−1 |
The content of cellulase (%) |
1, 2, 3, 4, 5, 6 |
9000 rpm, 20 min, pH 5.0, 55 °C, 120 min, 1 : 40 g mL−1 |
Hydrolysis time (min) |
30, 60, 90, 120, 150, 180 |
9000 rpm, 20 min, 5.0% cellulase, pH 5.0, 55 °C, 1 : 40 g mL−1 |
pH value |
3.0, 3.5, 4.0, 4.5, 5.0, 5.5, 6.0 |
9000 rpm, 20 min, 5.0% cellulase, 55 °C, 120 min, 1 : 40 g mL−1 |
Hydrolysis temperature (°C) |
40, 45, 50, 55, 60, 65 |
9000 rpm, 20 min, 5.0% cellulase, pH 4.5, 120 min, 1 : 40 g mL−1 |
Raw material to water ratio (g mL−1) |
1 : 30, 1 : 35, 1 : 40, 1 : 45, 1 : 50, 1 : 55 |
9000 rpm, 20 min, 5.0% cellulase, pH 4.5, 55 °C, 120 min |
2.2.3 Ultrasonic-assisted extraction method (u-SDF). The procedure of ultrasonic-assisted extraction was performed using a PS-30ALD ultrasonic cleaning bath (Kunshan Ultrasonic Instrument Co. Ltd., Jiangsu, China). 2.0 g powder was processed in 80 mL deionized water by multiple enzymes (α-amylase and neutral protease) hydrolysis in sequence. Then, cellulase was added to the hydrolysate solutions and placed it into an ultrasonic cleaning bath, and then extracted under controlled conditions (the additive quantity of cellulase of 5%, hydrolysis temperature of 50 °C, hydrolysis time of 40 min, pH value of 4.5, and ultrasonic power of 180 W). After inactivation of enzymes, the supernatant was condensed, alcohol precipitated and freeze–dried to achieve u-SDF.
2.2.4 Acidic extraction method (a-SDF). Pineapple pomace (2.0 g) was suspended in 30 times volume of oxalic acid solution (3%, w/v) with constant stirring using a water bath at 90 °C for 2 h, then filtered, condensed and precipitated by 95% ethanol, the a-SDF was obtained by dried in a lyophilizer.
2.2.5 Cellulase-assisted alkaline extraction method (c-SDF). 2.0 g of pineapple pomace was suspended in 80 mL of distilled water and mixed with 6% cellulase, adjusted pH value to 4.5. After incubation at 55 °C for 1 h, the mixture was heated at 95 °C for 5 min and then filtered, the residue was treated by NaOH solution at 60 °C and pH 11.0 for 1.5 h. After extraction, merged the above supernatant, condensed, precipitated and freeze–dried to obtain c-SDF.
2.3 Optimization of s-SDF extraction
Seven single-factor experiments were done to determine the preliminary range of the extraction variables, including cutting speed (X1, 5000–10
000 rpm), cutting time (X2, 10–60 min), the content of cellulase (X4, 1–6%), hydrolysis time (X5, 30–180 min), pH value (X7, 3.0–6.0), hydrolysis temperature (X8, 40–65 °C) and raw material to water ratio (X10, 1
:
40–1
:
50 g mL−1). And then the main influencing factors were screened out from seven factors affecting the extraction yield of s-SDF by Plackett–Burman (PB) design. The whole design consisting of 10 factors and 12 experimental points, each factor was set at two levels of low (−1) and high (+1). In addition, three empty terms (X3, X6 and X9) were arranged for error analysis. The factors and levels are shown in Table 2. According to the results of PB design, the positive and negative effects of significant factors were selected to determine the change direction and step size of the steepest climbing test, and to approach the maximum response area quickly.18
Table 2 Factors and levels of the Plackett–Burman design
No. |
Factors |
Levels |
Low level (−1) |
High level (+1) |
X1 |
Cutting speed (rpm) |
8000 |
10 000 |
X2 |
Cutting time/min |
10 |
30 |
X3 |
Empty column 1 |
|
|
X4 |
The content of cellulase (%) |
4.0 |
6.0 |
X5 |
Hydrolysis time (min) |
90 |
150 |
X6 |
Empty column 2 |
|
|
X7 |
pH value |
4.0 |
5.0 |
X8 |
Hydrolysis temperature (°C) |
50 |
60 |
X9 |
Empty column 3 |
|
|
X10 |
Raw material to water ratio (g mL−1) |
1 : 40 |
1 : 50 |
The optimal conditions for shear homogenization-assisted cellulase extraction with the standard of three-level-three-factor Box–Behnken design were determined by response surface methodology (RSM). The content of cellulase (A), hydrolysis time (B) and hydrolysis temperature (C) were selected as independent variables, the extraction yield of s-SDF (Y) was taken as the response value, while the cutting speed, cutting time, pH value and raw material to water ratio were fixed to 9000 rpm, 20 min, 4.5 and 1
:
45 g mL−1, respectively. The whole design consisting of 17 experimental points including 12 factorial points and 5 central points, and the factors and levels are shown in Table 3. The relationships and interrelationships of the variables were based on the following second-order polynomial model:
|
Y = β0 + ∑βixi + ∑βiixi2 + ∑βijxixj
| (2) |
where
Y was the predicted response,
xi and
xj were the independent variable,
βi,
βj,
βii and
βj were the model intercept, linear, quadratic and interaction coefficients, respectively.
Table 3 Factors and levels of the Box–Behnken design
Level |
Factors |
A, the content of cellulase (%) |
B, hydrolysis time (min) |
C, hydrolysis temperature (°C) |
−1 |
4.5 |
100 |
50 |
0 |
5.0 |
120 |
55 |
1 |
5.5 |
140 |
60 |
2.4 Absorption capacity
2.4.1 Cholesterol binding capacity (CBC). Cholesterol binding capacity of SDF was investigated according to the procedure method described with minor modifications.14 Fresh egg yolk was diluted with 9 times volume of distilled water, and homogenized by stirring. The mixtures of 1.0 g SDF with 50 mL of diluted yolk at pH 2.0 and 7.0, respectively (similar to the pH conditions in the stomach and small intestine, respectively), were shaken at 37 °C for 2 h in a thermostatic oscillator. At the end of absorption, the mixture was centrifuged at 5000 rpm for 20 min. The supernatant was collected and diluted with 5 times volume of glacial acetic acid. Briefly, 0.2 mL of supernatant dilution solution was mixed with glacial acetic acid (0.8 mL) and o-phthalaldehyde (0.2 mL) in a 10 mL test tube in sequence, and then, 4.0 mL of mixed acid (glacial acetic acid and sulfuric acid, 1
:
1, v/v) was added. After reacting for 10 min, the absorbance at 550 nm was determined with a UV-1200 spectrophotometer (Shanghai, China). A linear relationship between cholesterol concentrations and the absorbances was as follows: y = 0.0129x − 0.0294 (R2 = 0.9926), where y represents the absorbance, x represents the cholesterol concentration (μg mL−1). The CBC was calculated as follows: |
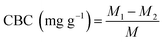 | (3) |
where M1 and M2 were the content of cholesterol in the yolk without or with SDF, M was the weight of the sample.
2.4.2 Sodium cholate binding capacity (SCBC). Following the method of Xu et al.19 with minor modifications. 0.5 g of samples were mixed with 50 mL of NaCl solution (0.15 mol L−1, pH 7.0) containing 0.2 g sodium cholate and shaken at 37 °C for 2 h, then centrifuged at 4000 rpm for 20 min. The supernatant (1 mL) was collected and mixed with 6 mL of 45% sulfuric acid and 1 mL of 0.3% furaldehyde. After incubation at 65 °C for 30 min, the absorbance at 620 nm was measured. A linear relationship between sodium cholate concentrations and the absorbances was as follows: y = 1.2785x + 0.0262 (R2 = 0.9993), where y represents the absorbance, x represents the sodium cholate concentration (mg mL−1). SCBC was calculated using the following equation: |
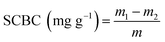 | (4) |
where m1 was the content of sodium cholate in the solution before absorption, m2 was the content of sodium cholate in the solution after absorption, and m was the weight of sample.
2.4.3 Nitrite ion absorption capacity (NIAC). The assays for absorption rate of SDF to nitrite ion were carried out according to the method described with slight modifications.20 0.25 g of SDF samples were mixed with 50 mL sodium nitrite solution (50 μg mL−1) in a 100 mL conical flask, the pH was adjusted to 2.0 and 7.0 (simulsting the pH conditions in the stomach and small intestine), respectively. The mixture was shaken at 37 °C for 2 h in a thermostatic oscillator, then centrifuged at 4000 rpm for 20 min. 0.6 mL of the supernatant was collected and mixed with 2 mL of p-aminobenzene sulfonic acid (0.4%, v/v), 1.0 mL of N-1-naphthylethylenediamine dihydrochloride (0.2%, v/v) in a 50 mL volumetric flask. After incubation at RT for 15 min, the absorbance at 538 nm was determined. The relationship between sodium nitrite concentrations and the absorbances was estimated as follows: y = 0.6587x + 0.0095 (R2 = 0.999), where y represents the absorbance, x represents the sodium nitrite concentration (μg mL−1). The NIAC was calculated using the following equation: |
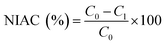 | (5) |
where C0 and C1 were the concentration of sodium nitrite in the buffer without or with SDF, respectively.
2.4.4 Oil absorption capacity (OAC). The OAC was determined according to the method described by Wang et al.22 with slight modifications. 0.50 g of SDF (recorded as W1) was mixed with 10 mL of peanut oil in a 15 mL of centrifuge tube, and maintained for 24 h at RT, and then the mixture was centrifuged at 5000 rpm for 10 min. After the supernatant was discarded, the residue was collected and weighed (recorded as W2). The OAC was calculated using the following equation: |
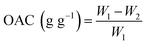 | (6) |
2.4.5 Glucose absorption capacity (GAC). The GAC of SDF was determined according to the method described by C. F. Chau et al.21 with some modifications. 0.25 g of SDF was mixed with 50 mL of glucose solution (100 mmol L−1) in a 100 mL triangular bottle and incubated in a thermostatic oscillator at 37 °C for 6 h. After centrifugation at 4500 rpm for 20 min, the supernatant was collected and the concentration of glucose was determined at 540 nm by colorimetric method. A standard curve of glucose concentrations to the absorbances as follows: y = 2.8477x + 0.1448 (R2 = 0.9981), where y represents the absorbance, x represents the concentration of glucose (mg mL−1). GAC was calculated according to the following equation: |
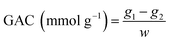 | (7) |
where g1 and g2 were the glucose content before or after absorption, and w was the weight of sample.
2.5 Assay of antioxidant activity of s-SDF in vitro
The s-SDF samples were diluted with distilled water into different concentrations of 0.2, 0.4, 0.6, 0.8 and 1.0 mg mL−1. The antioxidant activities were evaluated by scavenging abilities of DPPH˙, ABTS+, hydroxyl radicals, superoxide anion radicals and reducing power.
2.5.1 DPPH radical (DPPH˙) scavenging activity. The DPPH radical scavenging activity was determined according to the reported method with slight modifications.23 Briefly, 2.0 mL of s-SDF sample solutions with different concentrations (0.2–1.0 mg mL−1) were mixed with 2 mL of DPPH ethanol solution (0.2 mmol L−1). After reacted for 30 min in the dark at RT, the absorbance of the mixture was measured at 517 nm using a UV-1200 spectrophotometer. Ascorbic acid (Vc) was used as positive control. The DPPH radical scavenging activity was calculated by the following formula: |
 | (8) |
where Ai was the absorbance of the sample, Aj was the absorbance of the sample only (ethanol instead of DPPH solution), A0 was the absorbance of the control (distilled water instead of s-SDF solution).
2.5.2 ABTS+ radical scavenging activity. The ABTS+ radical scavenging activity was measured as described by Martínez R. et al.24 with slight modifications. Briefly, equal volumes of ABTS solution (7 mmol L−1) and potassium persulfate solution (2.45 mmol L−1) were added to a flask, and reacted in the dark for 12–16 h at 4 °C. In the moment of use, the mixture solution was diluted with methyl alcohol to an absorbance of 0.70 ± 0.02 at 732 nm. 50 μL of s-SDF solution with various concentrations (0.2–1.0 mg mL−1) were mixed with 4 mL of ABTS+ solution. After reacted at RT for 6 min in the dark, the absorbance at 732 nm was measured using Vc as positive control. The ABTS+ radical scavenging activity was calculated by the following formula: |
 | (9) |
where A0 was the absorbance of the control without sample, A1 was the absorbance of sample, and A2 was the absorbance of sample blank without ABTS+.
2.5.3 Hydroxy radical (·OH) scavenging activity. The hydroxyl radical scavenging activity was determined according to the previous method with some modifications.25 Firstly, 1 mL of s-SDF sample (0.2–1.0 mg mL−1), 0.5 mL of salicylic acid–ethanol (9 mmol L−1), and 0.5 mL of FeSO4 (9 mmol L−1) were mixed in a 15 mL centrifuge tube, 5 mL of H2O2 (8.8 mmol L−1) was added finally to start the reactions, and then incubated at 37 °C for 30 min. Afterwards, the absorbance of the mixture was measured at 510 nm using Vc as positive control, and the hydroxy radical scavenging activity was calculated according to the following formula: |
 | (10) |
where A0 was the absorbance of the blank (distilled water instead of s-SDF sample), Aj was the background absorbance (distilled water instead of FeSO4), and Ai was the absorbance of sample.
2.5.4 Superoxide anion radical (O2−˙) scavenging activity. The superoxide anion scavenging activity was measured as described in the previous study.26 with some modifications in this test. 1 mL s-SDF solution at various concentrations (0.2–1.0 mg mL−1) were mixed with 4 mL Tris–HCl buffer solution (0.05 mol L−1, pH 8.2) and 0.4 mL pyrogallol solution (25 mmol L−1) in sequence. After the mixtures were reacted at RT for 5 min, 100 μL of HCl (10 mol L−1) was added to terminate the reaction. The absorbance at 325 nm was measured. Vc was used as positive control, and the superoxide anion radical scavenging activity was calculated using the equation as following: |
 | (11) |
where Aq was the absorbance of the control (distilled water instead of s-SDF sample), Am was the absorbance of s-SDF sample in reactive system, and An was the absorbance of sample only (distilled water instead of pyrogallol).
2.5.5 Reducing power. The reducing power of s-SDF sample was carried out by the previous method.27 Briefly, 1.0 mL of s-SDF solution at various concentrations (0.2–1.0 mg mL−1) in distilled water were mixed with 2.5 mL phosphate buffer (0.2 mol L−1, pH 6.6), and 2.5 mL 1% K3Fe(CN)6 solution. Subsequently, the mixtures were incubated at 50 °C for 20 min in the water bath. After cooled to RT, 2.5 mL of 10% trichloroacetic acid was added, and then centrifuged at 5000 rpm for 15 min. 2.5 mL mixture supernatant was collected and mixed with 2.5 mL of distilled water and 0.5 mL of ferric chloride (FeCl3). The absorbance at 700 nm was determined after 10 min. Vc was used as positive control. Increased absorbance of the reaction mixture demonstrated the increase of reducing ability. |
Reducing power = A1 − A2
| (12) |
where A1 was the absorbance of sample, and A2 was the absorbance of sample only (distilled water instead of ferric chloride).
2.6 Statistical analysis
A software of Design-Expert 8.0.6 was used for RSM experiment design and data analysis. All of the results were expressed as the mean values ± standard deviation (SD). The differences between means were assessed by analysis of variance (ANOVA) with Dunca's test using a significance level of p < 0.05.
3 Results and discussion
3.1 Single-factor experiment
Taking the extraction yield of s-SDF as the assessment index, a single-factor experiment was performed to determine the preliminary range of the extraction variables (Fig. 1). As shown in Fig. 1a, the extraction yield of s-SDF first increased to its maximum level as the cutting speed was increased to 9000 rpm and then decreased with further increases the cutting speed, which may be interpreted that increasing cutting speed decreases particle size and increases specific surface area of pineapple pomace, and accelerating the mass transfer of intracellular substances. However, excessively cutting speed could promote the degradation of s-SDF. Hence, 9000 rpm was chosen as the optimum cutting speed. The analysis of the effect of cutting time on the extraction yield of s-SDF (Fig. 1b) revealed that the extraction yield of s-SDF presented a relatively rapid increase as the cutting time was increased from 10 to 20 min, and then slightly decreased as cutting time was over 20 min, indicating a longer cutting time could decrease the particle size of pineapple pomace and increase the mass transfer rate of s-SDF. Therefore, the optimum cutting time of 20 min was chosen in the present work. According to Fig. 1c, the extraction yield of s-SDF increased rapidly with the content of cellulase ranged from 1% to 5%, beyond which it slightly decreased. That could be due to the increase of the content of cellulase might increase the contact chances of cellulase to substrate, which benefitted the dissolution of s-SDF. However, high additive quantity of cellulase could decrease the hydrolysis rate of the substrate and increase the produce cost. Thus, the content of cellulase of 5% was chosen in this experiment. According to Fig. 1d, the extraction yield slowly increased during the initial 120 min and then it decreased on further increase of hydrolysis time. s-SDF dissolution requires a certain time, but thermal degradation could occur if the hydrolysis time is too long, which could affect the s-SDF extraction yield. Therefore, the optimum hydrolysis time was 120 min. As shown in Fig. 1e, there was a rapid increase in the extraction yield of s-SDF when the pH value increased from 3.0 to 4.5, and then it decreased slowly on further increase of pH. This tendency could be explained by the fact that pH value affected the dissolution of s-SDF by influencing the cellulase activity, weakly acidic or neutral environment could decrease the cellulase activity. Hence, 4.5 was chosen as the optimum pH value. The effect of hydrolysis temperature (Fig. 1f) revealed that the yield of s-SDF first increased then tends to decrease with increasing of hydrolysis temperature, where an optimum value was 55 °C. Both the mass transfer rate and the density of the solvent might be affected by the temperature. Proper high temperature could increase cellulase activity and molecular movement, accelerating the dissolution of SDF. However, higher hydrolysis temperature may reduce the enzyme activity and promote the degradation of SDF. Therefore, 55 °C was chosen as the optimum hydrolysis temperature. Fig. 1g shown the effect of raw material to water ratio on the extraction yield of s-SDF. The extraction yield first increased then tends to keep constant with increasing of raw material to water ratio from 1
:
30 to 1
:
55 g mL−1, where an optimum value was 1
:
45 g mL−1. A larger ratio of raw material to water implied greater concentration difference between the interior plant cells and the exterior solvent, thereby dissolving more SDF from the material. However, too much solvents could increase the operation difficulty of subsequent concentration. Therefore, the optimum raw material to water ratio of 1
:
45 g mL−1 was chosen.
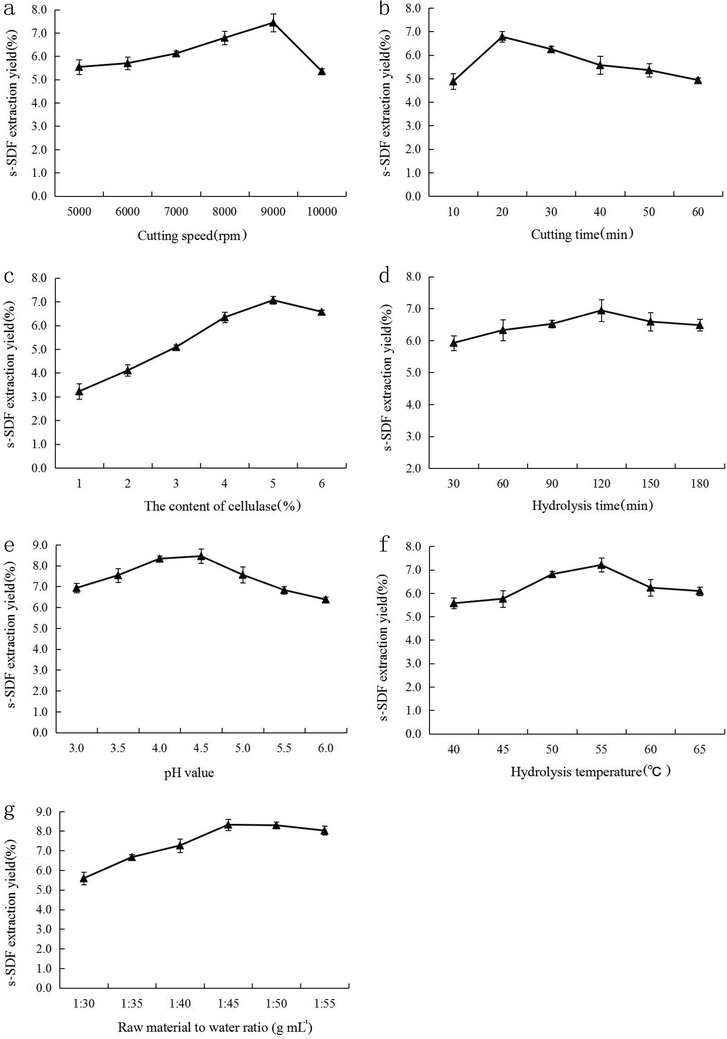 |
| Fig. 1 The effects of cutting speed (a), cutting time (b), the content of cellulase (c), hydrolysis time (d), pH value (e), hydrolysis temperature (f) and raw material to water ratio (g) on the extraction yield of s-SDF. | |
3.2 Determination of significance factors
The Plackett–Burman design and corresponding results were shown in Table 4. The regression equation between extraction factors and the extraction yield of s-SDF was arranged as follows:
Y = 6.71 + 0.01X1 + 0.02X2 − 0.62X4 − 0.10X5 − 0.06X7 + 0.11X8 − 0.02X10. |
where Y was the extraction yield of s-SDF, X1, X2, X4, X5, X7, X8 and X10 were cutting speed, cutting time, the content of cellulase, hydrolysis time, pH value, hydrolysis temperature and raw material to water ratio, respectively.
Table 4 Plackett–Burman design and corresponding results
No. |
X1 |
X2 |
X3 |
X4 |
X5 |
X6 |
X7 |
X8 |
X9 |
X10 |
s-SDF extraction yield (%) |
1 |
1 |
−1 |
1 |
−1 |
−1 |
−1 |
1 |
1 |
1 |
−1 |
5.72 |
2 |
1 |
1 |
−1 |
1 |
−1 |
−1 |
−1 |
1 |
1 |
1 |
7.31 |
3 |
−1 |
1 |
1 |
−1 |
1 |
−1 |
−1 |
−1 |
1 |
1 |
6.02 |
4 |
1 |
−1 |
1 |
1 |
−1 |
1 |
−1 |
−1 |
−1 |
1 |
7.05 |
5 |
1 |
1 |
−1 |
1 |
1 |
−1 |
1 |
−1 |
−1 |
−1 |
7.54 |
6 |
1 |
1 |
1 |
−1 |
1 |
1 |
−1 |
1 |
−1 |
−1 |
5.89 |
7 |
−1 |
1 |
1 |
1 |
−1 |
1 |
1 |
−1 |
1 |
−1 |
7.38 |
8 |
−1 |
−1 |
1 |
1 |
1 |
−1 |
1 |
1 |
−1 |
1 |
7.27 |
9 |
−1 |
−1 |
−1 |
1 |
1 |
1 |
−1 |
1 |
1 |
−1 |
7.45 |
10 |
1 |
−1 |
−1 |
−1 |
1 |
1 |
1 |
−1 |
1 |
1 |
6.73 |
11 |
−1 |
1 |
−1 |
−1 |
−1 |
1 |
1 |
1 |
−1 |
1 |
6.00 |
12 |
−1 |
−1 |
−1 |
−1 |
−1 |
−1 |
−1 |
−1 |
−1 |
−1 |
6.19 |
The variance analysis of the regression equation was presented in Table 5. The low p-value indicating that the model was highly significant. The high value of determination coefficient (R2 = 0.9848) and adjusted determination coefficient (Radj2 = 0.8329) indicating that 83.29% of the variations could be explained by the model, and the regression equation had a good fitting degree. The influential order of the seven factors on the extraction yield of s-SDF was arranged as follows: X4 > X8 > X5 > X7 > X2 > X10 > X1. Therefore, the three key factors, i.e., the content of cellulase, hydrolysis temperature and hydrolysis time were selected for subsequent optimization and analysis of the RSM, while other factors were fixed at the specific values as follows: cutting speed of 9000 rpm, cutting time of 20 min, raw material to water ratio of 1
:
45 g mL−1, and pH value of 4.5.
Table 5 The variance analysis of Plackett–Burman design
Factors |
Coefficient |
Standard error |
T value |
P value |
Importance |
Model |
6.712 |
0.082 |
81.36 |
0.008 |
— |
X1 |
0.006 |
0.117 |
0.07 |
0.955 |
7 |
X2 |
0.022 |
0.117 |
0.27 |
0.830 |
5 |
X4 |
−0.621 |
0.117 |
−7.53 |
0.084 |
1 |
X5 |
−0.104 |
0.117 |
−1.26 |
0.426 |
3 |
X7 |
−0.061 |
0.117 |
−0.74 |
0.596 |
4 |
X8 |
0.106 |
0.117 |
1.28 |
0.422 |
2 |
X10 |
−0.0175 |
0.117 |
−0.21 |
0.867 |
6 |
S = 0.4070 |
R2 = 0.9848 |
Radj2 = 0.8329 |
|
|
|
The steepest ascent direction and step size were determined according to the variable relationship of three important factors of PB design. The content of cellulase (A) and hydrolysis time (B) exhibited negative effects, whereas hydrolysis temperature (C) showed a positive effect. The steepest ascent test and corresponding results were shown in Table 6, the extraction yield of s-SDF first increased and then decreased with increasing of hydrolysis temperature, and the decrease of the content of cellulase and hydrolysis time, the maximum extraction yield may appear in test 3. Then the conditions of test 3 was used as the center point to carry out the subsequent Box–Behnken design (BBD).
Table 6 Slope climbing test and corresponding results
No. |
Factors |
s-SDF extraction yield (%) |
The content of cellulase (%) |
Hydrolysis time (min) |
Hydrolysis temperature (°C) |
1 |
6.0 |
160 |
45 |
7.33 |
2 |
5.5 |
140 |
50 |
7.48 |
3 |
5.0 |
120 |
55 |
8.06 |
4 |
4.5 |
100 |
60 |
7.00 |
5 |
4.0 |
80 |
65 |
4.64 |
3.3 Optimization of SDF extraction conditions
RSM was used to optimize the extractions conditions of s-SDF. The design matrix and the corresponding results of BBD were shown in Table 7. The data were analyzed by multiple regression equation analysis to obtain the following second-order polynomial equation:
Y = 8.556 + 0.748A − 0.247B − 0.346C + 0.202AB − 0.390AC + 0.102BC − 1.398A2 − 1.025B2 − 0.403C2 |
where Y was the extraction yield of s-SDF, and A, B and C were the content of cellulase, hydrolysis time and hydrolysis temperature, respectively.
Table 7 Design and results of Box–Behnken experiment
No. |
Factors |
s-SDF extraction yield (%) |
A |
B |
C |
1 |
−1 |
−1 |
0 |
5.59 |
2 |
1 |
−1 |
0 |
6.74 |
3 |
−1 |
1 |
0 |
5.12 |
4 |
1 |
1 |
0 |
7.08 |
5 |
−1 |
0 |
−1 |
5.92 |
6 |
1 |
0 |
−1 |
8.14 |
7 |
−1 |
0 |
1 |
6.15 |
8 |
1 |
0 |
1 |
6.81 |
9 |
0 |
−1 |
−1 |
8.11 |
10 |
0 |
1 |
−1 |
6.98 |
11 |
0 |
−1 |
1 |
7.07 |
12 |
0 |
1 |
1 |
6.35 |
13 |
0 |
0 |
0 |
8.56 |
14 |
0 |
0 |
0 |
8.87 |
15 |
0 |
0 |
0 |
8.41 |
16 |
0 |
0 |
0 |
8.32 |
17 |
0 |
0 |
0 |
8.62 |
The variance analysis of the quadratic regression model was shown in Table 8. The model had a high F-value (27.76) and a low p-value (<0.001) indicated that the quadratic model was highly significant (p < 0.01), the high value of R2 (0.9728) and the Radj2 (0.9377) also clearly demonstrated that the model was statistically significant. Meanwhile, the p-value of lack of fit was 0.1519 indicating that the extraction yield of s-SDF can be accurately predicted using the quadratic model. Additionally, The linear coefficients A and B, quadratic term coefficients A2, B2 and C2, and the interaction terms AC of the regression equation were significant with small p values (p < 0.05), whereas the other term coefficients were insignificant (p > 0.05). The influential order of the three factors on the extraction yield of s-SDF was arranged as A > C > B, that is the content of cellulase > hydrolysis temperature > hydrolysis time.
Table 8 ANOVA for response surface quadratic modela
Variance sources |
Sum of squares |
DF |
Mean squares |
F value |
P value |
Significance |
** Indicates extremely significant differences (p < 0.01); * indicates significant differences (p < 0.05). |
Model |
21.30 |
9 |
2.37 |
27.76 |
0.0001 |
** |
A |
4.49 |
1 |
4.49 |
52.61 |
0.0002 |
** |
B |
0.49 |
1 |
0.49 |
5.75 |
0.0476 |
* |
C |
0.96 |
1 |
0.96 |
11.25 |
0.0122 |
** |
AB |
0.16 |
1 |
0.16 |
1.92 |
0.2080 |
|
AC |
0.61 |
1 |
0.61 |
7.14 |
0.0319 |
* |
BC |
0.042 |
1 |
0.042 |
0.49 |
0.5053 |
|
A2 |
8.23 |
1 |
8.23 |
96.53 |
<0.0001 |
** |
B2 |
4.43 |
1 |
4.43 |
51.94 |
0.0002 |
** |
C2 |
0.68 |
1 |
0.68 |
8.02 |
0.0253 |
* |
Residual |
0.60 |
7 |
0.085 |
|
|
|
Lack of fit |
0.42 |
3 |
0.14 |
3.09 |
0.1519 |
|
Pure error |
0.18 |
4 |
0.045 |
|
|
|
Cor total |
21.90 |
16 |
|
|
|
|
As the graphical representations of a regression equation, 3D response surface and 2D contour plots were made to predict the relationships between the independent variables and the dependent variables.28 As shown in Fig. 2, the extraction yield of s-SDF was investigated when two varieties kept in experimental range and other variety fixed at zero. For the content of cellulase and hydrolysis time (Fig. 2a and b), the contour plot was circular, which indicated the mutual interactions between the content of cellulase and hydrolysis time was insignificant. The similar trend (Fig. 2e and f) was found for hydrolysis temperature and hydrolysis time. The elliptical contour plot shown in Fig. 2c and d indicated the mutual interactions between the content of cellulase and hydrolysis temperature was significant. By solving the regression equation and analyzing the response surface contour plots, the optimal extraction conditions were obtained as follows: the content of cellulase 5.0%, hydrolysis time 120 min, hydrolysis temperature 50 °C. The maximum predicted extraction yield of s-SDF was 8.80%. To validate the reliability of the model equation, the verification experiment was carried out under optimal extraction conditions. As a result, an actual extraction yield of 8.76 ± 0.06% was obtained, which was not significant different from the predicated value, indicating that the model was adequate for the prediction of s-SDF extraction.
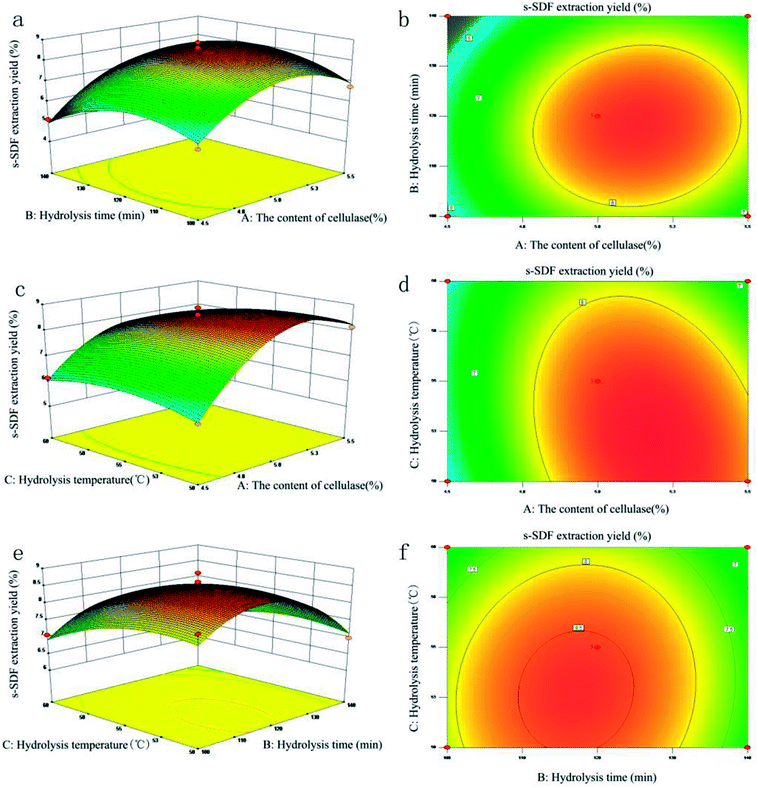 |
| Fig. 2 Respose surfaces plots showing the mutual effects of the content of cellulase (a and b), hydrolysis time (c and d) and hydrolysis temperature (e and f) on the extraction yield of s-SDF. | |
3.4 Functional characteristics of SDF
3.4.1 Binding capacities of sodium cholate, cholesterol and oil. Dietary fiber is an important component of our diet, although it is not digested by endogenous enzymes within the human intestinal tract. Especially the non-starch polysaccharides and lignin of the plant origin, may lower cholesterol and fat contents. DF is capable of binding bile acids in the small intestine and binding cholesterol in both the stomach and small intestine.29 Therefore, the binding capacities of DF for sodium cholate, cholesterol and oil were commonly considered as the important parameters to evaluate its adsorption capacity for lipophilic components.4In this study, the absorption properties of SDF obtained by four extraction methods were investigated. The extraction yield and binding capacities of fiber samples (s-SDF, u-SDF, a-SDF and c-SDF) to sodium cholate, cholesterol (at pH 2.0 and pH 7.0) and oil were listed in Table 9. The results shown that the extraction yield of s-SDF was 8.76%, which is lower than the extraction yield of a-SDF (12.19%) and c-SDF (15.08%), but higher than that of u-SDF (8.12%). The binding capacities of a-SDF and s-SDF to sodium cholate was significantly higher than those of u-SDF and c-SDF. The binding capacities to cholesterol was dramatically influenced by the pH value of reaction system. The CBC of four kinds of dietary fiber at pH 7.0 was better than those at pH 2.0, and s-SDF exhibited obvious binding capacities for cholesterol in vitro, which indicated that the CBC in small intestine was higher than that in stomach, and s-SDF exhibited better healthy effect to lower the concentration of cholesterol in the small intestine.
Table 9 Comparison of extraction yield and absorption capacities of s-SDF, u-SDF, a-SDF and c-SDF
|
s-SDF |
u-SDF |
a-SDF |
c-SDF |
s-SDF, soluble dietary fiber prepared from pineapple pomace by shear homogenization-assisted cellulase extraction method; u-SDF, ultrasonic-assisted cellulase extraction method; a-SDF, acid extraction method; c-SDF, cellulase-assisted alkali extraction method; data are presented as the mean values ± SDs (n = 3). Different letters within the same line are significantly different at the level of p < 0.05, and the same letters within the same line are insignificantly different at the level of p > 0.05. *Is significantly different at the level of p < 0.05 (comparing pH 2.0 and pH 7.0). |
SDF extraction yield (%) |
8.76 ± 0.06b |
8.12 ± 0.04b |
12.19 ± 0.04a |
15.08 ± 0.13a |
SCBC (mg g−1) pH 7.0 |
9.29 ± 0.77b |
6.28 ± 0.82c |
10.82 ± 0.31a |
2.45 ± 0.29d |
CBC (mg g−1) |
pH 2.0 |
14.07 ± 0.55ab |
14.56 ± 0.33a |
13.51 ± 0.16bc |
13.16 ± 0.26c |
pH 7.0 |
15.23 ± 0.83a |
15.49 ± 0.23a |
13.86 ± 0.09b |
14.86 ± 0.19ab |
NIAC (mg g−1) |
pH 2.0 |
4.27 ± 0.05b* |
4.43 ± 0.04a |
4.34 ± 0.08ab |
4.14 ± 0.02c |
pH 7.0 |
2.44 ± 0.24b |
2.10 ± 0.16bc |
3.24 ± 0.12a |
1.93 ± 0.11c |
OAC (g g−1) |
1.11 ± 0.09b |
1.03 ± 0.02b |
0.86 ± 0.02c |
1.34 ± 0.12a |
GAC (mmol g−1) |
19.12 ± 0.06c |
19.34 ± 0.04ab |
19.45 ± 0.04a |
19.32 ± 0.06b |
The ability of SDF to retain oil is important for food applications, such as preventing fat loss upon cooking, improving the texture and sensory quality of foods and removing excess fat from the human body.30 As shown in Table 9, the c-SDF had higher binding capacity for peanut oil (1.34 ± 0.12 g g−1) compared with s-SDF (1.11 ± 0.09 g g−1), u-SDF (1.03 ± 0.02 g g−1) and a-SDF (0.86 ± 0.02 g g−1). A comparison of the OAC of s-SDF with the OACs of SDF from other sources indicated that the OAC of s-SDF was similar to the papaya skin (1.15 ± 0.09 g g−1) but lower than those of orange peel (1.76 ± 0.32 g g−1) and rice bran (1.85 ± 0.15 g g−1),22,31 which may be due to the different sources and extraction methods of the above SDF.
3.4.2 Absorption capacity of nitrite ion. Nitrite is a compound with relatively high toxicity, which is commonly used for the curing of meat for a desirable color and prevention of bacterial growth. Consumption of cured meat products and vegetables containing natural nitrate could increase content of nitrites in human digestive system, which react with degradation products of amino acids to form the carcinogenic important trait. DF contributes to the prevention of human toxication by absorption over dose of nitrites.2 The NIAC of s-SDF, u-SDF, a-SDF and c-SDF were showed that the condition in the stomach was higher than that in intestine, and the NIAC of s-SDF was higher than c-SDF, but lower than u-SDF and a-SDF.
3.4.3 Absorption of glucose. The viscous and gel forming properties of DF slows down gastric emptying and macronutrient adsorption from the gut which marks reduction in postprandial glucose responses.12,32 The influence of four kinds of SDF on the postprandial glucose level was analyzed by in vitro glucose adsorption assay. The results shown that s-SDF, u-SDF, a-SDF and c-SDF can absorb 19.12, 19.34, 19.45 and 19.32 mmol g−1 of glucose at the concentration of 100 mmol L−1, respectively, which indicated that SDF from different extraction methods absorbs glucose effectively and thus can play a potential role in reducing postprandial glucose.
3.4.4 Antioxidant activities. Several methods have been developed to estimate the antioxidant capacity of different plant materials, these methods including measure the ability of antioxidants to scavenge specific radicals, to inhibit lipid peroxidation or to chelate metal ions. Usually, more than one method should be used to gain a more complete picture of the antioxidant capacity of active compounds, as illustrated by the data in Fig. 3 that show the antioxidant activity of s-SDF using each of the DPPH˙, ABTS+, hydroxyl radicals, superoxide anion radicals and reducing power assays.
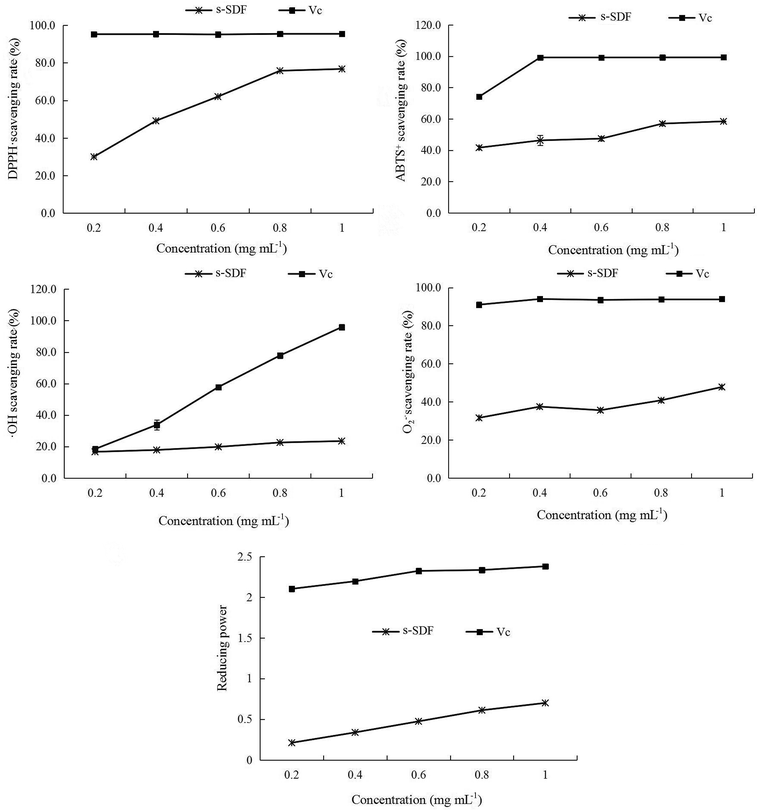 |
| Fig. 3 The scavenging capacity of s-SDF from pineapple pomace against DPPH˙, ABTS+, hydroxyl radicals, superoxide anion free radicals and reducing power. | |
The DPPH radical does not requires any special preparation and is considered a simple and very fast method for determining antioxidant activity. Results in Fig. 3 demonstrated that the s-SDF exhibited outstanding DPPH scavenging ability, with a dose-dependent manner. The scavenging rates at the concentration of 1.0 mg mL−1 was 76.72%. The ABTS assay is based on the ability of antioxidants to scavenge the long-life cation ABTS+. With the increases of s-SDF concentration, the scavenging ability to ABTS+ radical was gradually increased. The scavenging rates was 58.40% at 1.0 mg mL−1. The scavenging ability of s-SDF to hydroxyl radicals was insignificantly improved with the concentration increased from 0.2 mg mL−1 to 1.0 mg mL−1, which indicated that s-SDF had a moderate scavenging ratio for hydroxyl radicals, the scavenging rate was only 23.47% when the concentration was 1.0 mg mL−1. Superoxide anions radical is considered as the initial free radicals formed from the mitochondrial electron transport system. Fig. 3 shown that the s-SDF had a certain ability to remove superoxide anions. With the increase of s-SDF concentration, the scavenging ability to superoxide radical was gradually improved. At the concentration of 1.0 mg mL−1, the scavenging rates reached 47.79%, but lower than the scavenging rate of Vc. Meanwhile, the s-SDF presented stronger reducing power, reaching 0.7 at 1.0 mg mL−1.
3.5 Discussion
Greater consumption of fruits, vegetables and cereals, which contain relatively high levels of dietary fiber, contributes to the decrease of the risk of chronic diseases caused by hyperlipidemia or hyperglycemia. In recent years, attention was focused on the fibers extracted from fruit peels, seeds, and cores, a much more cheap and available resource, while those from pineapple pomace have not been well investigated. Nowadays, chemical, enzymatic, and enzyme-assisted chemical methods have been used to extract SDF from different food sources. Differences of extraction conditions could affect the structure and composition of SDF, and further influence the physicochemical properties.33,34 Studies have shown that acid or alkali extraction can destroy the glycosidic bond of DF, resulting in almost loss of 100% SDF, 30–40% hemicellulose, and 10–20% cellulose.35 Some scholars have also used α-amylase, cellulase, glucosidase, neutral protease, or papain to extract DF from wheat bran, sweet potatoes, potatoes, bean dregs, cereal bran, and oats. However, these methods usually use a variety of enzymes for continuous extraction, the operation process is cumbersome, and the enzyme utilization rate is low.36,37 Shear homogenization technology is mainly used for agitating and crushing some fluid materials. During the high-speed relative rotation of the spindle and rotor, the material could withstand tens of thousands of shears, tears, and mixing per minute under huge centrifugal force to achieve a dispersed and homogeneous effect. To date, shear homogenization-assisted extraction has been used to extract peptides from corn gluten, which has mild extraction conditions, high yield, continuous production and easily achieves industrialization compared with traditional extraction methods. However, there was no report on its application in the extraction of SDF. In this study, the pineapple pomace was decomposed by shear homogenization-assisted enzymatic hydrolysis to prepare soluble dietary fiber, and the optimum extraction conditions were determined by PB design and RSM. The extraction yield of s-SDF from pineapple pomace reached to 8.76%.
The changes in the particle size of dietary fiber may affect its structure, porosity, and specific surface area, and then affected its physical and functional properties. Reducing the particle size of the dietary fiber from lime does not change the absorption capacity of glucose, but could significantly increase the glucose arrest and bile acid block indices.38 The absorption capacity analysis in vitro indicated that s-SDF exhibited higher CBC than u-SDF, a-SDF and c-SDF. In addition, the OAC and SCBC of s-SDF were higher than those of u-SDF and c-SDF, which might be partly mechanism for their hypolipidemic effect. Meanwhile, s-SDF could reduce the diffusion speed of bile acids, cholesterol, glucose molecules and nitrite or trap part of them due to its high viscosity and network structure. In addition, s-SDF generally showed more promising capacities for binding cholesterol, fat and sodium cholate, and the cholesterol binding capacity in small intestine was higher than that in stomach. Their binding capacities to fat and cholesterol could disturb the absorption of lipids in the digestive system, while binding of bile acids could promote the conversion of cholesterol to bile acids in liver, which could reduce the levels of total cholesterol and LDL cholesterol as a result.39 After entering the stomach and intestines, dietary fiber absorbs the moisture and oil in the food through its swellability and its water and oil-holding capacity, thus expanding the volume, enhancing the satiety of the human body, and effectively reducing the intake of food, fat, and calories. The oil-holding capacity of s-SDF was lower than that in orange peel and rice bran, indicating that pineapple pomace s-SDF has a certain space for development in reducing the absorption of oil in the human intestine.
The antioxidant manifestations of dietary fiber are mainly free radical scavenging, inhibition of lipid peroxidation, increase in body antioxidant enzyme activity, and adsorption of chelated metal ions. Studies have reported that citrus peel dietary fiber could remove DPPH˙ and nitrite, and the clearance rate was not affected by irradiation treatment.38 The effect of drying temperature on the antioxidant properties shown that orange dietary fiber has the strongest antioxidant capacity at 60 °C, and drying at 80–90 °C or 30–40 °C for a long time could reduce its antioxidant capacities.38 However, the antioxidant activity analysis of pineapple pomace dietary fiber obtained by shear homogenization-assisted enzymatic method in vitro has not been reported. This study has found that s-SDF showed scavenging ability to DPPH˙, ABTS+, ·OH, and O2−˙ in a concentration dependent manner. At a mass concentration of 1.0 mg mL−1, the scavenging rates of DPPH˙, ABTS+, ·OH, and O2−˙ reached 76.72%, 58.40%, 47.79% and 23.47%, respectively, indicating that the prepared s-SDF has strong antioxidant activity.
In this study, we compared the properties of s-SDF with those of u-SDF, a-SDF and c-SDF. The extraction yield of s-SDF was slightly lower than that of a-SDF and c-SDF, but higher than u-SDF. Meanwhile, the adsorption ability of s-SDF to cholesterol and sodium cholate was improved compared with u-SDF and c-SDF, and possessed higher antioxidant activities. Cellulase-assisted alkali extraction method (c-SDF) and acid extraction method (a-SDF) have been widely used to extract SDF from different materials due to high extraction yield, but they have some drawbacks such as great solvent consumption, high requirements of temperature, and introduction of a large number of ions during the reaction process. Shear homogenization-assisted extraction method (s-SDF) is a new and promising biological extraction method, which resulting in low energy consumption, low environment pollution and high product safety. This study provides a theoretical basis for the application of shear homogenization-assisted extraction method for the preparation of pineapple pomace dietary fiber in health care products.
4 Conclusion
RSM were used to optimize the extraction conditions of s-SDF based on PB design, and the optimal extraction conditions were obtained as follows: the content of cellulase 5.0%, hydrolysis time 120 min, hydrolysis temperature 50 °C, cutting speed 9000 rpm, cutting time 20 min, pH value 4.5, and raw material to water ratio 1
:
45 g mL−1. The extraction yield of s-SDF under these conditions was 8.76 ± 0.06%, the slight difference between the theoretical (8.80%) and actual extraction yield indicated the optimization process was feasible. The in vitro absorption capacity experiments revealed that s-SDF had a higher binding capacity for lipophilic components especially for sodium cholate, cholesterol, and oil. The scavenging activities to DPPH˙, ABTS+, hydroxyl radicals, superoxide anion radicals and reducing power assays indicated that s-SDF has stronger antioxidant activities. Which implies the potential applications of s-SDF as an ingredient in formulated foods. Further investigations on the potential hypolipidemic ability of s-SDF using animal-feeding experiments in vitro are awaited.
Conflicts of interest
There are no conflicts to declare.
Acknowledgements
The study was financially supported by a grant-in-aid for Scientific Research from the Public Welfare Industry (Agriculture) of China (201503142-13 and 201503142-14).
References
- L. Sepúlveda, A. Romaní, C. N. Aguilar and J. Teixeira, Valorization of pineapple waste for the extraction of bioactive compounds and glycosides using autohydrolysis, Innovative Food Sci. Emerging Technol., 2018, 47, 38–45 CrossRef.
- X. Luo, Q. Wang, B. Zheng, L. Lin and B. Chen, Hydration properties and binding capacities of dietary fibers from bamboo shoot shell and its hypolipidemic effects in mice, Food Chem. Toxicol., 2017, 109, 1003–1009 CrossRef CAS PubMed.
- W. Zhang, G. Zeng, Y. Pan, W. Chen, W. Huang and Y. Li, Properties of soluble dietary fiber-polysaccharide from papaya peel obtained through alkaline or ultrasound-assisted alkaline extraction, Carbohydr. Polym., 2017, 172, 102–112 CrossRef CAS PubMed.
- Y. Zhu, J. Chu, Z. Lu, F. Lv, X. Bie and H. Zhao, Physicochemical and functional properties of dietary fiber from foxtail millet (Setaria italica) bran, J. Cereal Sci., 2018, 79, 456–461 CrossRef CAS.
- H. Chen, C. Zhao, J. Li, S. Hussain, S. Yan and Q. Wang, Effects of extrusion on structural and physicochemical properties of soluble dietary fiber from nodes of lotus root, LWT–Food Sci. Technol., 2018, 93, 204–211 CrossRef CAS.
- Y. Zheng, Y. Li, J. Xu, G. Gao and F. Niu, Adsorption activity of coconut (Cocos nucifera L.) cake dietary fibers: effect of acidic treatment, cellulase hydrolysis, particle size and pH, RSC Adv., 2018, 8, 2844–2850 RSC.
- J. I. Mann JHC, Possible implications for health of the different definitions of dietary fibre, Nutr., Metab. Cardiovasc. Dis., 2009, 19(3), 226–229 CrossRef PubMed.
- L. MJAM, Position of the american dietetic association: health implications of dietary fiber, J. Am. Diet. Assoc., 2008, 108, 1716–1731 CrossRef.
- J. F. Ayala-Zavala, V. Vegavega, C. Rosasdomínguez, H. Palafoxcarlos and J. A. Villarodriguez, Agro-industrial potential of exotic fruit byproducts as a source of food additives, Food Res. Int., 2011, 44, 1866–1874 CrossRef CAS.
- M. Viudamartos, M. C. Lópezmarcos and J. Fernándezlópez, Role of fiber in cardiovascular diseases: a review, Compr. Rev. Food Sci. Food Saf., 2010, 9, 240–258 CrossRef CAS.
- M. P. Mcrae, Dietary fiber is beneficial for the prevention of cardiovascular disease: an umbrella review of meta-analyses, Journal of Chiropractic Medicine, 2017, 9, 289–299 CrossRef PubMed.
- K. B. Arun, S. Thomas, T. R. Reshmitha, G. C. Akhil and P. Nisha, Dietary fibre and phenolic-rich extracts from Musa paradisiaca inflorescence ameliorates type 2 diabetes and associated cardiovascular risks, J. Funct. Foods, 2017, 31, 198–207 CrossRef CAS.
- X. Luo, Q. Wang, D. Fang, W. Zhuang, C. Chen and Y. Zheng, Modification of insoluble dietary fibers from bamboo shoot shell: structural characterization and functional properties, Int. J. Biol. Macromol., 2018, 120, 1461–1467 CrossRef CAS PubMed.
- L. Wang, H. Xu, F. Yuan, Q. Pan, R. Fan and Y. Gao, Physicochemical characterization of five types of citrus dietary fibers, Biocatal. Agric. Biotechnol., 2015, 4, 250–258 CrossRef.
- M. Mengmei and M. Taihua, Modification of deoiled cumin dietary fiber with laccase and cellulase under high hydrostatic pressure, Carbohydr. Polym., 2016, 136, 87–94 CrossRef PubMed.
- V. Tejada-Ortigoza, L. E. Garcia-Amezquita, S. O. Serna-Saldívar and J. Welti-Chanes, Advances in the functional characterization and extraction processes of dietary fiber, Food Eng. Rev., 2015, 8, 1–21 Search PubMed.
- W. Zhang, G. Zeng and Y. Pan, Properties of soluble dietary fiber-polysaccharide from papaya peel obtained through alkaline or ultrasound-assisted alkaline extraction, Carbohydr. Polym., 2017, 172, 102–112 CrossRef CAS PubMed.
- H. Hu Q. Zhao and J. Xie, Polysaccharides from pineapple pomace: new insight into ultrasonic-cellulase synergistic extraction and hypoglycemic activities, Int. J. Biol. Macromol., 2019, 121, 1213–1226 CrossRef PubMed.
- H. Xu, Q. Jiao, F. Yuan and Y. Gao, In vitro binding capacities and physicochemical properties of soluble fiber prepared by microfluidization pretreatment and cellulase hydrolysis of peach pomace, LWT–Food Sci. Technol., 2015, 63, 677–684 CrossRef CAS.
- Y. Wang, Y. Zhou, Y. Cheng, Z. Jiang, Y. Jin and G. Zhang, Enzymo-chemical preparation, physico-chemical characterization and hypolipidemic activity of granular corn bran dietary fibre, J. Food Sci. Technol., 2013, 52(3), 1718–1723 CrossRef PubMed.
- C. F. Chau, Y. L. Wen and Y. T. Wang, Improvement of the functionality of a potential fruit insoluble fibre by micron technology, Int. J. Food Sci. Technol., 2006, 41, 1054–1060 CrossRef CAS.
- L. Wang, H. Xu, F. Yuan, R. Fan and Y. Gao, Preparation and physicochemical properties of soluble dietary fiber from orange peel assisted by steam explosion and dilute acid soaking, Food Chem., 2015, 185, 90–98 CrossRef CAS PubMed.
- C. SenthilKumara, M. Sivakumarb and K. Ruckmania, Microwave-assisted extraction of polysaccharides from Cyphomandra betacea and its biological activities, Int. J. Biol. Macromol., 2016, 92, 682–693 CrossRef PubMed.
- R. Martínez, P. Torres and M. A. Meneses, Chemical, technological and in vitro antioxidant properties of mango, guava, pineapple and passion fruit dietary fibre concentrate, Food Chem., 2012, 135, 1520–1526 CrossRef PubMed.
- S. Shen, S. Jia, R. Yan, Y. Lin, D. Zhao and P. Han, Effect of culture conditions on the physicochemical properties and antioxidant activities of polysaccharides from Nostoc flagelliforme, Carbohydr. Polym., 2018, 198, 426–433 CrossRef CAS PubMed.
- L. Chen and G. Huang, Extraction, characterization and antioxidant activities of pumpkin polysaccharide, Int. J. Biol. Macromol., 2018, 118, 770–774 CrossRef CAS PubMed.
- X. Liang, Y. Gao, W. Fei, Y. Zou, M. He, L. Yin, Z. Yuan and W. Zhang, Chemical characterization and antioxidant activities of polysaccharides isolated from the stems of Parthenocissus tricuspidata, Int. J. Biol. Macromol., 2018, 119, 70–78 CrossRef CAS PubMed.
- Z. Ye, W. Wei, Q. Yuan, H. Ye, Y. Sun and H. Zhang, Box-Behnken design for extraction optimization, characterization and in vitro antioxidant activity of Cicer arietinum L. hull polysaccharides, Carbohydr. Polym., 2016, 147, 354–364 CrossRef CAS PubMed.
- D. Mudgil and S. Barak, Composition, properties and health benefits of indigestible carbohydrate polymers as dietary fiber: a review, Int. J. Biol. Macromol., 2013, 61, 1–6 CrossRef CAS PubMed.
- B. O. Schneeman, Fiber, inulin and oligofructose: similarities and differences, J. Nutr., 1999, 129, 1424S–1427S CrossRef CAS PubMed.
- G. A. Jacometti, L. R. P. F. Mello, P. H. A. Nascimento, A. C. Sueiro and F. Yamashita, The physicochemical properties of fibrous residues from the agro industry, LWT–Food Sci. Technol., 2015, 62, 138–143 CrossRef CAS.
- D. J. Jenkins, C. W. Kendall, M. Axelsen, L. S. Augustin and V. Vuksan, Viscous and nonviscous fibres, nonabsorbable and low glycaemic index carbohydrates, blood lipids and coronary heart disease, Curr. Opin. Lipidol., 2000, 11, 49–56 CrossRef CAS PubMed.
- N. Emgl and S. Sjm, Modification of physicochemical properties of dietary fibre in carrots by mono- and divalent cations, Food Chem., 2002, 76, 273–280 CrossRef.
- L. Prosky, N. G. Asp, T. F. Schweizer, J. W. Devries and I. Furda, Determination of insoluble, soluble, and total dietary fiber in foods and food products: interlaboratory study, J. - Assoc. Off. Anal. Chem., 1988, 71, 1017–1023 CAS.
- A. S. Meyer, B. P. Dam and H. N. Lærke, Enzymatic solubilization of a pectinaceous dietary fiber fraction from potato pulp: optimization of the fiber extraction process, Biochem. Eng. J., 2009, 43, 106–112 CrossRef CAS.
- W. Quan and H. Guo, The effect of high-speed emulsification pretreatment on the acidic protease
catalyzed hydrolysis of corn gluten meal, Food Sci. Technol., 2007, 33(8), 35–38 CAS.
- C. F. Chau, Y. T. Wang and Y. L. Wen, Different micronization methods significantly improve the functionality of carrot insoluble fibre, Food Chem., 2007, 100, 1402–1408 CrossRef CAS.
- P. Peerajit, N. Chiewchan and S. Devahastin, Effects of pretreatment methods on health-related functional properties of high dietary fibre powder from lime residues, Food Chem., 2012, 4, 1891–1898 CrossRef.
- Y. Niu, Z. Xie, H. Zhang, Y. Sheng and L. Yu, Effects of structural modifications on physicochemical and bile acid-binding properties of psyllium, J. Agric. Food Chem., 2013, 61, 596–601 CrossRef CAS PubMed.
Footnote |
† The two authors contributes equally to this work. |
|
This journal is © The Royal Society of Chemistry 2018 |
Click here to see how this site uses Cookies. View our privacy policy here.