DOI:
10.1039/C8RA06780E
(Paper)
RSC Adv., 2018,
8, 30374-30378
Silver-catalyzed direct benzylation of acetanilide: a highly efficient approach to unsymmetrical triarylmethanes†
Received
13th August 2018
, Accepted 13th August 2018
First published on 28th August 2018
Abstract
A highly efficient silver-catalyzed dibenzylation of acetanilides by employing the benzhydrol derivatives as the coupling partners to yield triphenylmethane derivatives has been developed. Various functional groups were tolerated, leading to the corresponding products in moderate to good yields. Preliminary experimental results indicated that the silver ions activate the arene rings and the strong acid TfOH provides the carbocations.
Introduction
Triarylmethane derivatives are a class of important substructures which can be usually found in organic dyes,1 fluorescent compounds,2 supramolecular structures, and pharmaceutically-related compounds.3–5 In the last decade, several routes have been explored in the synthesis of the triarylmethane scaffold.6,7 For example, the transition metal-catalyzed cross-coupling reaction has been developed to provide a direct approach to the synthesis of the triarylmethanes, which greatly enriched the synthetic methods to prepare these structures (Scheme 1a).8–14 However, the application of this method has been limited due to the requirement for the pre-functionalization during the synthesis of suitable coupling partners. Reduction of triarylmethanol derivatives offers another approach to obtain the triarylmethanes, which usually involved a multistep process (Scheme 1b).15,16 The Friedel–Crafts reaction is one of the most simple and widely employed routes for the preparation of triarylmethanes (Scheme 1c).17–20 The group of Mccubbin reported a pentafluorophenylboronic acid catalyzed method for the synthesis of triarylmethanes from benzhydrol with electronic rich arenes.21 Aoyama and co-workers developed a simple and efficient approach for alkylation of aromatics from alcohols with NaHSO4/SiO2 NaHSO4/SiO2 as the catalyst.22 Nevertheless, this approach is limited by the substrate scope because electronic donating group-substituted arenes were required to realize the transformation. To the best of our knowledge, there are still no examples of coupling of diphenylmethanol and acetanilide.23 In this perspective, the development of a new catalyst system to address the aforementioned limitation for Friedel–Crafts reactions is highly imperative to construct the triarylmethanes. Herein, we report a silver-catalyzed direct benzylation of acetanilides with benzhydrol derivatives as the coupling partners under mild conditions (Scheme 1d). A wide variety of functional groups are well tolerated, yielding the corresponding benzylated products in moderate to good yields. Preliminary experimental results showed that the silver ions activate the arene rings and trifluoromethanesulfonic acid (TfOH) provides the carbocations.
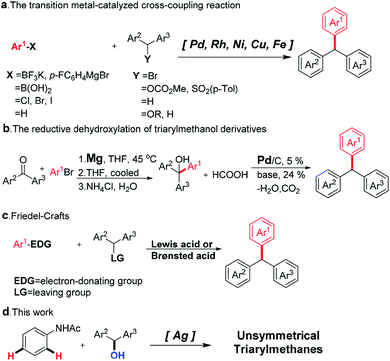 |
| Scheme 1 Approaches to the synthesis of triphenylmethanes. | |
The skeleton of the aniline derivative was usually found in the triphenylmethane-based bioactive compounds, such as pararosaniline, leucomalachite green, malachite green, and leucocrystal violet.1 Therefore, a highly efficient strategy for the construction the aniline-containing triphenylmethanes is highly desired.
Results and discussion
We envisioned that the aniline-containing triphenylmethane derivatives can be synthesized by treating the anilides with the benzhydrol derivatives via a transition metal catalyst. At the outset of our study, we treated acetanilide 1a with benzhydrol 2a in the presence of iron(III) chloride (20 mol%) in dichloroethane at 120 °C to investigate whether the diphenyl-methanation could be performed. We were pleased to find that the diphenylmethylated product 3aa was generated in 5% yield, along with the rest of the starting material 1a being recovered. Encouraged by this result, we next screened various Lewis acids such as InBr3, Fe(OTf)3, Zn(OTf)2, Al(OTf)3, Sc(OTf)3, and AgOTf to improve the efficiency of the reaction (Table 1). To our great delight, a dramatically improved yield (76%) of 3aa was obtained when AgOTf was used as the catalyst. Next, we tried to decrease the catalyst loading to 5 mol%; the product 3aa was obtained in 60% yield. A satisfactory yield of 3aa could also be achieved with 10 mol% AgOTf. In addition, several other solvents including toluene, CH3CN, t-amyl-OH, and 1,4-dioxane were investigated, but none of them gave better yields than that of DCE. The control experiment showed that the catalyst is indispensable for this silver-promoted dibenzylation-reaction (see ESI†).
Table 1 Optimization of reaction conditionsa
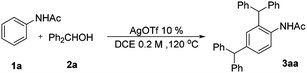
|
Entry |
Catalyst |
Solvent |
Yield (%)b |
Reaction conditions: 1a (0.1 mmol), 2a (0.2 mmol), cat, solvent (1 mL), 120 °C, 8 h. Yields were based on LC-MS analysis using acetyl benzene as an internal standard. |
1 |
FeCl3 20% |
DCE |
5 |
2 |
InBr3 20% |
DCE |
Trace |
3 |
Fe(OTf)3 20% |
DCE |
64 |
4 |
Zn(OTf)2 20% |
DCE |
5 |
5 |
Al(OTf)3 20% |
DCE |
0 |
6 |
Sc(OTf)3 20% |
DCE |
0 |
7 |
AgOTf 20% |
DCE |
76 |
8 |
AgOTf 10% |
DCE |
78 |
9 |
AgOTf 5% |
DCE |
60 |
10 |
AgOTf 10% |
Toluene |
6 |
11 |
AgOTf 10% |
CH3CN |
0 |
12 |
AgOTf 10% |
t-Amyl-OH |
0 |
13 |
AgOTf 10% |
1,4-Dioxane |
5 |
With the optimized conditions in hand, we next explored the substrate scope to investigate the functional group tolerance of this silver trifluoromethanesulfonate-catalyzed dibenzylation reaction (Scheme 2). Initially, a series of ortho-substituted acetanilides (1b–1f) performed well under the standard conditions, which afforded the corresponding para-diphenylmethylated products in moderate yields. It is worth noting that all the ortho-substituted acetanilides with electron withdrawing groups yielded para-dibenzylated products, whereas those with electronic donating groups (1m, 1o) afforded both the para- and ortho- position both diphenylmethylated products. This might be due to the fact that the electronic donating functional groups greatly activate the arene ring, which could trap the diphenylmethane carbon ion very quickly.
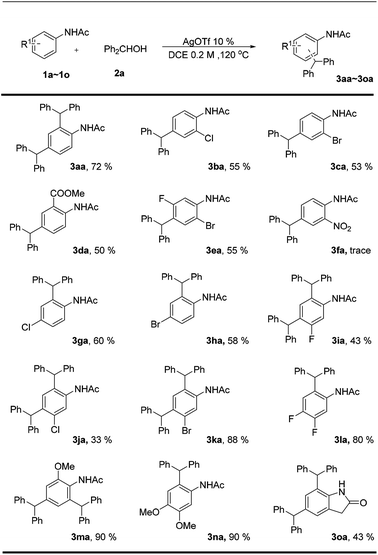 |
| Scheme 2 Scope of acetanilidea,b. aReaction conditions: 1a–1o (0.1 mmol), 2a (0.2 mmol), AgOTf 10%, DCE (1 mL), 120 °C, 8 h. bYields of isolated products. | |
Next, we investigated the para-substituted acetanilides (1g, 1h, and 1l); the ortho-diphenylmethylated products were obtained in good yields, which indicated that the electronic effects greatly affect the reactivity of the acetanilides. Interestingly, all the meta-substituted substrates provide both the ortho- and para-diphenylmethylated products (3ia–3ka) in good to moderate yields. The X-ray crystal structures of 3ca, 3ha, 3jb, and 3la are shown in Fig. 1.
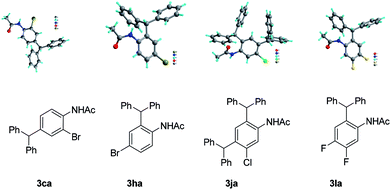 |
| Fig. 1 X-ray crystal structures of 3ca, 3ha, 3jb and 3la. | |
The bromide substituted arenes can be further transformed into various other functional groups by well-known cross-coupling reactions.24 Therefore, 2-bromoacetanilide was employed to investigate the scope of benzhydrol and the results were listed in Scheme 3. The results clearly show that the functional groups, such as Me, F, Cl, Br, CF3, and Ph were all fully tolerated in this reaction. All the dibenzylation took place selectively at the para-position. Even though the exact reason is unclear, we speculated that the steric effect is the major reason for the para-selectivity.
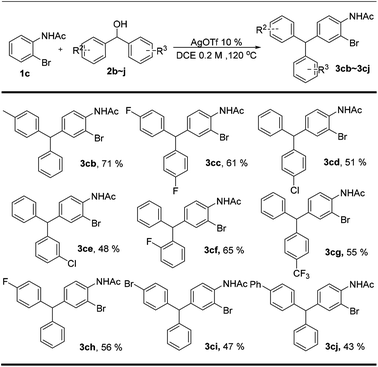 |
| Scheme 3 Scope of benzhydrola,b. aReaction conditions: 1c (0.1 mmol), 2b–2j (0.2 mmol), AgOTf 10%, DCE (1 mL), 120 °C, 8 h. bYields of isolated products. | |
To further demonstrate the synthetic utility of the developed synthetic protocol, gram-scale reactions were performed. To our delight, all the scale up reactions provided the corresponding products (3aa, 3ha) in good yields (Scheme 4).
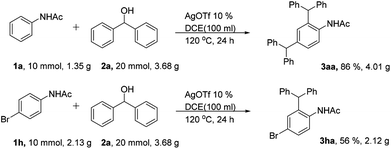 |
| Scheme 4 Gram-scale reactions. | |
In order to propose the reaction mechanism of this silver-catalyzed selective dibenzylation reaction, several experiments were further performed. TfOH was used instead of AgOTf, which afforded 35% 3aa (Scheme 5a); this result indicated that silver has a significant role in the realization of the dibenzylation reaction. However, when silver acetate was used as the catalyst, no dibenzylated product was obtained and the starting material 1a was completely recovered (Scheme 5b). Interestingly, when silver acetate (10 mol%) and TfOH (10 mol%) were used to instead of AgOTf, a good yield of 3aa was achieved (Scheme 5c). Moreover, when we added a proton scavenger Et3N (10 mol%) to the reaction, the reaction was well inhibited (scheme 5d). This result might indicate that the TfOH would generate the diphenyl-methane carbocation (complex II), and the silver ions might coordinate with oxygen to activate the acetanilides (complex I). To further support this point, N-methylacetanilide was subjected to the standard reaction conditions to investigate the occurrence of dibenzylation (Scheme 5e). Impressively, there was no dibenzylated product and only the starting material was recovered, which further validated our proposal. Next, the deuteration experiment was performed by directly adding D2O into the reaction (Scheme 5f). The result from this experiment may indicate that the silver only acted as a Lewis acid in this reaction.
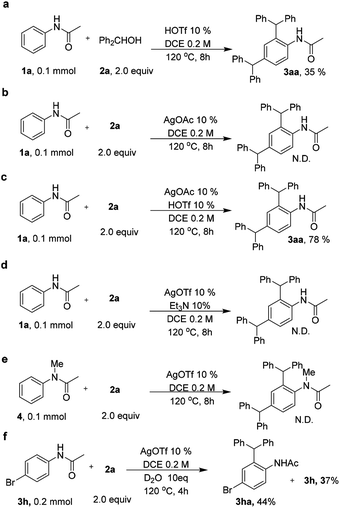 |
| Scheme 5 Preliminary mechanistic study. | |
Based on these results and previous reports6,7 a plausible reaction mechanism was proposed in Scheme 6. The carbocation I might be formed by combination with silver ions under acidic conditions. Meanwhile, the strong Lewis acid TfOH would enable the formation of the carbocation (complex II), which was further trapped by the complex I, affording the dibenzylated product.
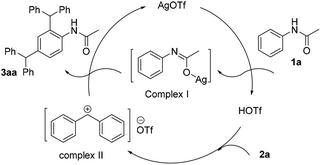 |
| Scheme 6 Proposed reaction mechanism. | |
Conclusions
In conclusion, we have developed a highly efficient approach for the synthesis of triphenylmethane derivatives through the direct combination of acetanilides and diphenylmethanol derivatives using AgOTf as the catalyst. A wide variety of functional groups are well tolerated, leading to the corresponding benzylated products in moderate to good yields. Preliminary experimental results indicated that the silver ions activate the arene rings and TfOH provides the carbocations. Further applications and mechanistic studies are now underway in our lab.
Conflicts of interest
There are no conflicts to declare.
Acknowledgements
We gratefully acknowledge financial support from the Natural Science Foundation of China (Nos. 21772139 and 21572149). Project of Scientific and Technologic Infrastructure of Suzhou (SZS201708) and the PAPD Project are also gratefully acknowledged.
Notes and references
- Reviews:
(a) D. F. Duxbury, Chem. Rev., 1993, 93, 381–433 CrossRef;
(b) M. S. Shchepinov and V. A. Korshun, Chem. Soc. Rev., 2003, 32, 170–180 RSC;
(c) V. Nair, S. Thomas, S. C. Mathew and K. G. Abhilash, Tetrahedron, 2006, 62, 6731–6747 CrossRef.
-
(a) C. D. Mason and F. F. Nord, J. Org. Chem., 1951, 16, 722–727 CrossRef;
(b) V. V. Ghaisas, B. J. Kane and F. F. Nord, J. Org. Chem., 1958, 23, 560–565 CrossRef;
(c) M. Irie, J. Am. Chem. Soc., 1983, 105, 2078–2079 CrossRef;
(d) R. Muthyala, A. R. Katritzky and X. F. Lan, Dyes Pigm., 1994, 25, 303–324 CrossRef.
-
(a) R. A. Al-Qawasmeh, Y. Lee, M. Y. Cao, X. Gu, A. Vassilakos, J. A. Wright and A. Young, Bioorg. Med. Chem., 2004, 14, 347–350 CrossRef;
(b) G. Panda, J. K. Mishra, V. Chaturvedi, A. K. Srivastave, R. Srivastava and B. S. Srivastava, Bioorg. Med. Chem., 2004, 12, 5269–5276 CrossRef PubMed.
-
(a) R. S. Dothager, K. S. Putt, B. J. Allen, B. J. Leslie, V. Nesterenko and P. J. Hergenrother, J. Am. Chem. Soc., 2005, 127, 8686–8696 CrossRef PubMed;
(b) Shagufta, A. K. Srivastava, R. Sharma, R. Mishra, A. K. Balapure, P. S. R. Murthy and G. Panda, Bioorg. Med. Chem., 2006, 14, 1497–1505 CrossRef PubMed.
-
(a) M. K. Parai, G. Panda, V. Chaturvedi, Y. K. Manju and S. Sinha, Bioorg. Med. Chem. Lett., 2008, 18, 289–292 CrossRef PubMed;
(b) P. R. alchaudhuri, V. Nesterenko and P. J. Hergenrother, J. Am. Chem. Soc., 2008, 130, 10274–10281 CrossRef PubMed.
- Reviews:
(a) M. Shiri, M. A. Zolfigol, H. G. Kruger and Z. Tanbakouchian, Chem. Rev., 2010, 110, 2250–2293 CrossRef PubMed;
(b) M. Nambo and C. M. Crudden, ACS Catal., 2015, 5, 4734–4742 CrossRef.
-
(a) M. Barbero, S. Cadamuro, S. Dughera, C. Magistris and P. Venturello, Org. Biomol. Chem., 2011, 9, 8393–8399 RSC;
(b) F. L. Sun, X. J. Zheng, Q. Gu, Q. L. He and S. L. You, Eur. J. Org. Chem., 2010, 47–50 CrossRef;
(c) S. Dhiman and S. S. V. Ramasastry, Org. Biomol. Chem., 2013, 11, 8030–8035 RSC;
(d) P. Arde and R. V. Anand, RSC Adv., 2016, 6, 77111–77115 RSC.
-
(a) G. A. Molander and M. D. Elia, J. Org. Chem., 2006, 71, 9198–9202 CrossRef PubMed;
(b) A. López-Pérez, J. Adrio and J. C. Carretero, Org. Lett., 2009, 11, 5514–5517 CrossRef PubMed.
- J. Y. Yu and R. Kuwano, Org. Lett., 2008, 10, 973–976 CrossRef PubMed.
-
(a) L. L. Cao, X. N. Li, F. Y. Meng and G. F. Jiang, Tetrahedron Lett., 2012, 53, 3873–3875 CrossRef;
(b) T. Niwa, H. Yorimitsu and K. Oshima, Org. Lett., 2007, 9, 2373–2375 CrossRef PubMed;
(c) G. Song, Y. Su, X. Gong, K. Han and X. Li, Org. Lett., 2011, 13, 1968–1971 CrossRef PubMed;
(d) S. Tabuchi, K. Hirano, T. Satoh and M. Miura, J. Org. Chem., 2014, 79, 5401–5411 CrossRef PubMed.
- X. Zhao, G. Wu, Y. Zhang and J. Wang, J. Am. Chem. Soc., 2011, 133, 3296–3299 CrossRef PubMed.
- J. Zhang, A. Bellomo, A. D. Creamer, S. D. Dreher and P. J. Walsh, J. Am. Chem. Soc., 2012, 134, 13765–13772 CrossRef PubMed.
- M. Tobisu, A. Yasutome, H. Kinuta, K. Nakamura and N. Chatani, Org. Lett., 2014, 16, 5572–5575 CrossRef PubMed.
-
(a) B. F. Shi, N. Maugel, Y. H. Zhang and J. Q. Yu, Angew. Chem., Int. Ed., 2008, 47, 4882–4886 CrossRef PubMed;
(b) X. Shen, N. N. Gu, Y. Liu and B. Dai, Chin. J. Chem., 2016, 34, 1033–1038 CrossRef.
- G. Cravotto, A. Procopio, M. Oliverio, L. Orioa and D. Carnaroglioa, Green Chem., 2011, 13, 2806–2809 RSC.
- S. Sawadjoon, A. Lundstedt and J. S. M. Samec, ACS Catal., 2013, 3, 635–642 CrossRef.
-
(a) J. Esquivias, R. G. Arrayas and J. C. Carretero, Angew. Chem., Int. Ed., 2006, 45, 629 CrossRef PubMed;
(b) V. Nair, N. Vidya and K. G. Abhilash, Synthesis, 2006, 3647 CrossRef.
-
(a) S. H. Lin and X. Y. Lu, J. Org. Chem., 2007, 72, 9757 CrossRef PubMed;
(b) S. Podder, J. Choudhury, U. K. Roy and S. Roy, J. Org. Chem., 2007, 72, 3100 CrossRef PubMed.
-
(a) I. Alonso, J. Esquivias, R. G. Arrayas and J. C. Carretero, J. Org. Chem., 2008, 73, 6401 CrossRef PubMed;
(b) G. J. Li, E. J. Wang, Y. Chen, Y. H. Liu and P. G. Wang, Tetrahedron, 2008, 64, 9033 CrossRef;
(c) C. R. Liu, M. B. Li, C. F. Yang and S. K. Tian, Chem. Commun., 2008, 1249 RSC.
- S. Gao, X. Xu, H. Yao and A. J. Lin, Eur. J. Org. Chem., 2016, 3006–3012 CrossRef.
- J. A. McCubbin and O. V. Krokhin, Tetrahedron Lett., 2010, 51, 2447–2449 CrossRef.
- Y. Sato, T. Aoyama, T. Takido and M. Kodomari, Tetrahedron, 2012, 68, 7077–7081 CrossRef.
-
(a) R. Sanz, A. Martínez, D. Miguel, J. M. Álvarez-Gutiérrez and F. Rodríguez, Adv. Synth. Catal., 2006, 348, 1841–1845 CrossRef;
(b) J. L. Bras and J. Muzart, Tetrahedron, 2007, 63, 7942–7948 CrossRef;
(c) H. B. Sun, B. Li, S. Chen, J. Li and R. Hua, Tetrahedron, 2007, 63, 10185–10188 CrossRef;
(d) J. A. McCubbin and O. V. Krokhin, Tetrahedron Lett., 2010, 51, 2447–2449 CrossRef;
(e) J. A. McCubbin and O. V. Krokhin, Tetrahedron Lett., 2010, 51, 2447–2449 CrossRef.
- Reviews:
(a) N. Miyaura and A. Suzuki, Chem. Rev., 1995, 95, 2457–2483 CrossRef;
(b) C. Liu, H. Zhang, W. Shi and A. Lei, Chem. Rev., 2011, 111, 1780–1824 CrossRef PubMed;
(c) J. Pouliot, F. Grenier, J. T. Blaskovits, S. Beaupré and M. Leclerc, Chem. Rev., 2016, 116, 14225–14274 CrossRef PubMed;
(d) P. R. Castillo and S. L. Buchwald, Chem. Rev., 2016, 116, 12564–12649 CrossRef PubMed;
(e) H. Yi, G. Zhang, H. Wang, Z. Huang, J. Wang, A. K. Singh and A. W. Lei, Chem. Rev., 2017, 117, 9016–9085 CrossRef PubMed;
(f) R. J. Procter, J. J. Dunsford, P. J. Rushworth, D. G. Hulcoop, R. A. Layfield and M. J. Ingleson, Chem.–Eur. J., 2017, 23, 15889–15893 CrossRef PubMed;
(g) Y. Xia, D. Qiu and J. B. Wang, Chem. Rev., 2017, 117, 13810–13889 CrossRef PubMed;
(h) T. Komiyama, Y. Minami, Y. Furuya and T. Hiyama, Angew. Chem., Int. Ed., 2018, 57, 1987–1990 CrossRef PubMed.
Footnote |
† Electronic supplementary information (ESI) available: CCDC 1842259–1842262. For ESI and crystallographic data in CIF or other electronic format see DOI: 10.1039/c8ra06780e |
|
This journal is © The Royal Society of Chemistry 2018 |
Click here to see how this site uses Cookies. View our privacy policy here.