DOI:
10.1039/C8RA06716C
(Paper)
RSC Adv., 2018,
8, 32659-32663
Cobalt(III)-catalyzed site-selective C–H amidation of pyridones and isoquinolones†
Received
10th August 2018
, Accepted 12th September 2018
First published on 21st September 2018
Abstract
In this study, Cp*Co(III)-catalyzed site-selective amidation of pyridones and isoquinolones using oxazolones as the amidation reagent is reported. This approach features mild conditions, high efficiency and good functional tolerance. Furthermore, gram-scale preparation and preliminary mechanism experiments were carried out. It provides a straightforward approach for the direct modification of pyridone derivatives.
Introduction
The pyridone motif is the cornerstone of a myriad of natural products and serves as useful building blocks in medicinal chemistry.1 Therefore, the development of efficient synthetic methodologies for the modification of pyridone derivatives has received intensive attention. Traditionally, transition metal-catalyzed cross-coupling of halogenated pyridones represents a practical and reliable strategy. On the contrary, recent advances in transition metal-promoted C–H functionalization2 allow the direct modification of heterocycles without prefunctionalization, featuring environmental friendliness, step- and atom-economy. Compared to the site-selective C–H functionalization at relatively electron-rich C5- and C3-positions of pyridones,3 access to the more electron-deficient C6 position remains undeveloped. Nakao and Hiyama reported C6-selective alkenylation and alkylation of 2-pyridone in the presence of nickel and aluminum catalysts.4 Recently, Miura and co-workers developed copper-mediated site-selective C–H heteroarylation at C6 position ingeniously with the aid of pyridine-based directing group.5 Based on the same pyridine-directed strategy, several research groups have reported transition-metal-catalyzed C6-selective C–H functionalization of pyridones.6 A majority of the reported methodologies demonstrated the formation of C–C bonds, while very few for the construction of C–N bonds. Li developed an elegant work of rhodium-catalyzed amination/annulation of pyridones with anthranils7 (Scheme 1a).6a Very recently, Samanta and co-workers reported C–H amidation of pyridones with various azides8 in the presence of iridium catalyst (Scheme 1b).6m
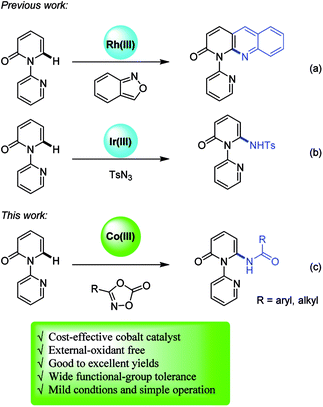 |
| Scheme 1 C6-selective amination or amidation of pyridones. | |
In the context of C–H activation, most of the developed methodologies employ the noble second- and third-row transition metals, such as rhodium and iridium in abovementioned progress. Recently, earth-abundant and environmental-friendly first row transition metals have been employed in C–H functionalization.9 Among the 3d metals, Cp*Co(III) is emerging as a robust and versatile catalyst due to its higher Lewis acidity and good selectivity.10 Since pioneering work by Matsunaga and Kanai,11 many chemists, in particular, Glorius,12 Ackermann,13 Ellman,14 Chang,15 Sundararaju,16 Cheng,17 and others,18 have demonstrated the unique reactivity of Cp*Co(III) catalyst. In continuation of our recent studies on C–H functionalization of pyridones,6c,6g we proposed the construction of C–N bond at C6 position of pyridones via Cp*Co(III)-catalyzed C–H activation. Herein, we report the Cp*Co(III)-catalyzed, site-selective C–H amidation of pyridones under mild conditions by the action of oxazolone19 as user-friendly amidating reagents7,8,20 (Scheme 1c).
Results and discussion
We commenced our studies by examining the reaction parameters of the coupling of 2-pyridone 1a with oxazolone 2a in the presence of [Cp*Co(CO)I2]. To our delight, the desired amidated product 3a was achieved in 94% yield (Table 1, entry 1). The optimization of solvents revealed that dichloromethane was the most effective solvent, affording product 3a in 98% yield, while no desired product could be obtained in protonic solvents (entries 2–6). The efficiency of the reaction was also significantly affected by Ag salt and no desired product was obtained in the absence of Ag salt (entries 7 and 8). Further screening of base indicated that base is crucial for this reaction and KOAc proved to be the best (entries 9–11). By contrast, the reaction gave no conversion when [Cp*Co(CO)I2] was omitted (entry 13).
Table 1 Optimization of reaction conditionsa

|
Entry |
Ag salt |
Additive |
Solvent |
Yieldb (%) |
Reaction conditions: 1a (0.2 mmol), 2a (0.6 mmol), [Cp*Co(CO)I2] (10 mol%), Ag salt (20 mol%) and base (30 mol%) in solvent (2.0 mL) under air at 90 °C for 12 h. Isolated yield. [Cp*Co(MeCN)3](SbF6)2 instead of [Cp*Co(CO)I2]. No catalyst. |
1 |
AgSbF6 |
KOAc |
DCE |
94 |
2 |
AgSbF6 |
KOAc |
DCM |
98 |
3 |
AgSbF6 |
KOAc |
CHCl3 |
Trace |
4 |
AgSbF6 |
KOAc |
TFE |
0 |
5 |
AgSbF6 |
KOAc |
MeOH |
0 |
6 |
AgSbF6 |
KOAc |
Dioxane |
39 |
7 |
AgNTf2 |
KOAc |
DCM |
10 |
8 |
— |
KOAc |
DCM |
0 |
9 |
AgSbF6 |
KOPiv |
DCM |
82 |
10 |
AgSbF6 |
NaOAc |
DCM |
96 |
11 |
AgSbF6 |
— |
DCM |
Trace |
12c |
— |
KOAc |
DCM |
90 |
13d |
AgSbF6 |
KOAc |
DCM |
0 |
With the optimized conditions identified, we investigated the scope and limitation of pyridones (Table 2). Satisfyingly, both electron-donating and -withdrawing groups at C3 position of pyridones were well tolerated, affording the desired amidated products in good to excellent yields (3b–3g). It was worth mentioning that halogen-substituted pyridones were also compatible in this catalytic system, guaranteeing further transformation (3d–3f). Similarly, in the cases of the C4-substituted pyridones, both electron-donating and -withdrawing groups were accomplished smoothly, giving the desired products in excellent yields (3h–3j). However, 5-methyl substituted 1k afforded no desired product, while 5-fluoro substituted 1l gave the desired product 3l in 86% yield, probably due to steric factors. Meanwhile, 4-pyridone could be amidated monoselectively at the C2 position (3m). Isoquinolinones with electron-donating and -withdrawing substitutions were also compatible in this transformation, yielding the corresponding products in high yields (3n–3q). Importantly, the site-selective C–H amidation was carried out on a gram scale without any additives to yield 3a in 93% yield (Scheme 2).
Table 2 Scope of pyridonesa,b
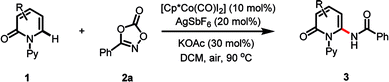
|
Reaction conditions: 1 (0.2 mmol), 2a (0.6 mmol), [Cp*Co(CO)I2] (10 mol%), AgSbF6 (20 mol%) and KOAc (30 mol%) in DCM (2.0 mL) under air at 90 °C for 12 h. Isolated yield. At 120 °C. |
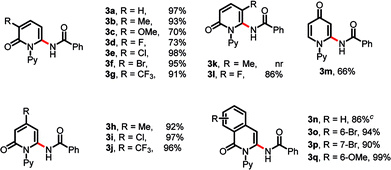 |
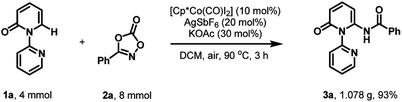 |
| Scheme 2 Gram-scale amidation of pyridone. | |
Next, we evaluated the scope of oxazolones (Table 3). Generally, amidation of 2-pyridone 1a with various substituted oxazolones proceeded efficiently to afford the desired products in good to excellent yields. Both electron-donating and -withdrawing substituents on the phenyl ring underwent the reaction smoothly (4a–4m). Additionally, the substituent of oxazolones is not limited to phenyl ring, 3-(thiophen-2-yl)-1,4,2-dioxazol-5-one also coupled to afford the products 4l in excellent yield. Gratifyingly, aliphatic substituent was also compatible in this reaction, producing the desired product in 94% yield (4m).
Table 3 Scope of oxazolonesa,b

|
Reaction conditions: 1a (0.2 mmol), 2 (0.6 mmol), [Cp*Co(CO)I2] (10 mol%), AgSbF6 (20 mol%) and KOAc (30 mol%) in DCM (2.0 mL) under air at 90 °C for 12 h. Isolated yield. At 120 °C. |
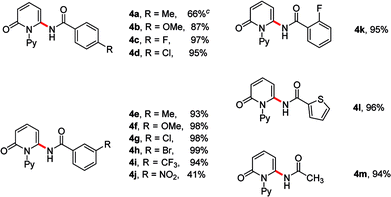 |
A series of control experiments were conducted to investigate the preliminary mechanism (Scheme 3). First, to gain insight into the C–H cleavage step, the hydrogen–deuterium (H/D) exchange experiments were carried out. No deuterium exchange with CD3OD was observed, indicating that the cobalt-mediated C–H bond cleavage is irreversible. On the other hand, by employing [D1]-1a as the substrate, the kinetic isotope effect (KIE) was tested and low level of primary kinetic isotope effects both in parallel and competition experiments were observed, implying that the C–H bond cleavage was not the rate-determining step in the transformation.21 Moreover, to probe the electronic preference, intermolecular competition experiments were carried out and the results indicated that the electron-rich substrate 1b reacted at a much higher rate.
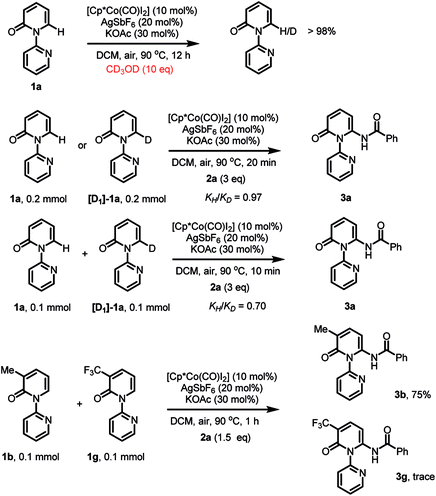 |
| Scheme 3 Control experiments. | |
Based on the preliminary results and literature precedents,13f,18a,f,g,h a plausible amidation mechanism is proposed (Scheme 4). First, a cationic Cp*Co(III) species, which was generated by the aid of Ag salt, undergoes electrophilic C–H bond cleavage of 2-pyridone irreversibly to form intermediate A, which is subsequently coordinated by 2a with the release of CO2. Next, migratory insertion of intermediate B affords the intermediate C. Finally, protodemetalation of intermediate C gives the desired product and regenerates the active catalyst.
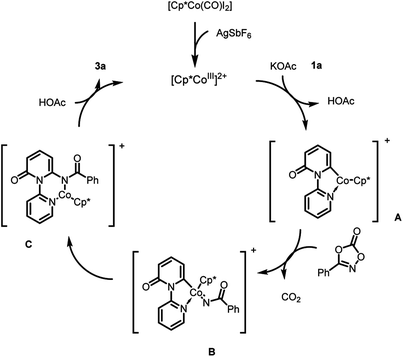 |
| Scheme 4 Proposed mechanism. | |
Conclusions
In conclusion, we have developed a method of Cp*Co(III)-catalyzed site-selective amidation of pyridones using oxazolones as amidation reagent under mild conditions. It provides a straightforward approach for the direct modification of pyridone derivatives, which are identified as privileged scaffolds with wide potential bioactivity in pharmaceuticals. Therefore, this efficient strategy will be of importance to medicinal chemists.
Conflicts of interest
There are no conflicts to declare.
Acknowledgements
We gratefully acknowledge financial support from the National Natural Science Foundation of China (81620108027, 21632008 and 81602975), the Major Project of Chinese National Programs for Fundamental Research and Development (2015CB910304).
Notes and references
-
(a) A. G. Amr and M. M. Abdulla, Bioorg. Med. Chem., 2006, 14, 4341 CrossRef PubMed;
(b) S. Hibi, K. Ueno, S. Nagato, K. Kawano, K. Ito, Y. Norimine, O. Takenaka, T. Hanada and M. Yonaga, J. Med. Chem., 2012, 55, 10584 CrossRef PubMed;
(c) G. Manfroni, F. Meschini, M. L. Barreca, P. Leyssen, A. Samuele, N. Iraci, S. Sabatini, S. Massari, G. Maga, J. Neyts and V. Cecchetti, Bioorg. Med. Chem., 2012, 20, 866 CrossRef PubMed;
(d) L. A. Hasvold, W. Wang, S. L. Gwaltney, T. W. Rockway, L. T. J. Nelson, R. A. Mantei, S. A. Fakhoury, G. M. Sullivan, Q. Li, N.-H. Lin, L. Wang, H. Zhang, J. Cohen, W.-Z. Gu, K. Marsh, J. Bauch, S. Rosenberg and H. L. Sham, Bioorg. Med. Chem. Lett., 2003, 13, 4001 CrossRef PubMed;
(e) S. Heeb, M. P. Fletcher, S. R. Chhabra, S. P. Diggle, P. Williams and M. Camara, FEMS Microbiol. Rev., 2011, 35, 247 CrossRef PubMed;
(f) C. Bengtsson and F. Almqvist, J. Org. Chem., 2010, 75, 972 CrossRef PubMed.
- Selected reviews and accounts:
(a) D. Alberico, M. E. Scott and M. Lautens, Chem. Rev., 2007, 107, 174 CrossRef PubMed;
(b) J. WencelDelord, T. Dröge, F. Liu and F. Glorius, Chem. Soc. Rev., 2011, 40, 4740 RSC;
(c) R. Giri, B.-F. Shi, K. M. Engle, N. Maugel and J.-Q. Yu, Chem. Soc. Rev., 2009, 38, 3242 RSC;
(d) L. Ackermann, Acc. Chem. Res., 2014, 47, 281 CrossRef PubMed;
(e) O. Daugulis, H.-Q. Do and D. Shabashov, Acc. Chem. Res., 2009, 42, 1074 CrossRef PubMed;
(f) X.-X. Guo, D.-W. Gu, Z. Wu and W. Zhang, Chem. Rev., 2015, 115, 1622 CrossRef PubMed;
(g) F. Hu, Y. Xia, C. Ma, Y. Zhang and J. Wang, Chem. Commun., 2015, 51, 7986 RSC;
(h) J. Wencel-Delord and F. Glorius, Nat. Chem., 2013, 5, 369 CrossRef PubMed;
(i) C. Liu, H. Zhang, W. Shi and A. Lei, Chem. Rev., 2011, 111, 1780 CrossRef PubMed;
(j) J. Yamaguchi, A. D. Yamaguchi and K. Itami, Angew. Chem., Int. Ed., 2012, 51, 8960 CrossRef PubMed;
(k) X.-S. Zhang, K. Chen and Z.-J. Shi, Chem. Sci., 2014, 5, 2146 RSC.
-
(a) A. Nakatani, K. Hirano, T. Satoh and M. Miura, Chem.–Eur. J., 2013, 19, 7691 CrossRef PubMed;
(b) A. Nakatani, K. Hirano, T. Satoh and M. Miura, J. Org. Chem., 2014, 79, 1377 CrossRef PubMed;
(c) E. E. Anagnostaki, A. D. Fotiadou, V. Demertzidou and A. L. Zografos, Chem. Commun., 2014, 50, 6879 RSC;
(d) A. Modak, S. Rana and D. Maiti, J. Org. Chem., 2015, 80, 296 CrossRef PubMed;
(e) A. Najib, S. Tabuchi, K. Hirano and M. Miura, Heterocycles, 2016, 92, 1187 CrossRef;
(f) P. Chauhan, M. Ravi, S. Singh, P. Prajapati and P. P. Yadav, RSC Adv., 2016, 6, 109 RSC;
(g) T. Itahara and F. Ouseto, Synthesis, 1984, 1984, 488 CrossRef;
(h) Y. Y. Chen, F. Wang, A. Q. Jia and X. W. Li, Chem. Sci., 2012, 3, 3231 RSC.
-
(a) Y. Nakao, H. Idei, K. S. Kanyiva and T. Hiyama, J. Am. Chem. Soc., 2009, 131, 15996 CrossRef PubMed;
(b) R. Tamura, Y. Yamada, Y. Nakao and T. Hiyama, Angew. Chem., Int. Ed., 2012, 51, 5679 CrossRef PubMed.
- R. Odani, K. Hirano, T. Satoh and M. Miura, Angew. Chem., Int. Ed., 2014, 53, 10784 CrossRef PubMed.
-
(a) S. Yu, Y. Li, X. Zhou, H. Wang, L. Kong and X. Li, Org. Lett., 2016, 18, 2812 CrossRef PubMed;
(b) Y. Li, F. Xie and X. Li, J. Org. Chem., 2016, 81, 715 CrossRef PubMed;
(c) P. Peng, J. Wang, H. Jiang and H. Liu, Org. Lett., 2016, 18, 5376 CrossRef PubMed;
(d) W. Miura, K. Hirano and M. Miura, Org. Lett., 2016, 18, 3742 CrossRef PubMed;
(e) T. Li, Z. Wang, K. Xu, W. Liu, X. Zhang, W. Mao, Y. Guo, X. Ge and F. Pan, Org. Lett., 2016, 18, 1064 CrossRef PubMed;
(f) K. Takamatsu, K. Hirano and M. Miura, Angew. Chem., Int. Ed., 2017, 56, 5353 CrossRef PubMed;
(g) P. Peng, J. Wang, C. Li, W. Zhu, H. Jiang and H. Liu, RSC Adv., 2016, 6, 57441 RSC;
(h) W. Miura, K. Hirano and M. Miura, J. Org. Chem., 2017, 82, 5337 CrossRef PubMed;
(i) J. Ni, H. Zhao and A. Zhang, Org. Lett., 2017, 19, 3159 CrossRef PubMed;
(j) S. Y. Chen, X. L. Han, J. Q. Wu, Q. Li, Y. Chen and H. Wang, Angew. Chem., Int. Ed., 2017, 56, 9939 CrossRef PubMed;
(k) D. Das, P. Poddar, S. Maity and R. Samanta, J. Org. Chem., 2017, 82, 3612 CrossRef PubMed;
(l) J. Xia, Z. Huang, X. Zhou, X. Yang, F. Wang and X. Li, Org. Lett., 2018, 20, 740 CrossRef PubMed;
(m) D. Das and R. Samanta, Adv. Synth. Catal., 2018, 360, 379 CrossRef.
-
(a) H. Jin, L. Huang, J. Xie, M. Rudolph, F. Rominger and A. S. K. Hashmi, Angew. Chem., Int. Ed., 2016, 55, 794 CrossRef PubMed;
(b) J. S. Baum, M. E. Condon and D. A. Shook, J. Org. Chem., 1987, 52, 2983 CrossRef.
-
(a) H. Chen and M. P. Huestis, ChemCatChem, 2015, 7, 743 CrossRef;
(b) W. Li and L. Dong, Adv. Synth. Catal., 2018, 360, 1104 CrossRef;
(c) J. Ryu, K. Shin, S. H. Park, J. Y. Kim and S. Chang, Angew. Chem., Int. Ed., 2012, 51, 9904 CrossRef PubMed.
- Selected reviews and accounts:
(a) M. Moselage, J. Li and L. Ackermann, ACS Catal., 2015, 6, 498 CrossRef;
(b) R. Shang, L. Ilies and E. Nakamura, Chem. Rev., 2017, 117, 9086 CrossRef PubMed;
(c) W. Liu and L. Ackermann, ACS Catal., 2016, 6, 3743 CrossRef;
(d) L. C. M. Castro and N. Chatani, Chem. Lett., 2015, 44, 410 CrossRef;
(e) W. Liu and Y. Bi, Chin. J. Org. Chem., 2012, 32, 1041 CrossRef.
-
(a) T. Yoshino and S. Matsunaga, Adv. Synth. Catal., 2017, 359, 1245 CrossRef;
(b) D. Wei, X. Zhu, J. Niu and M. Song, ChemCatChem, 2016, 8, 1242 CrossRef.
- T. Yoshino, H. Ikemoto, S. Matsunaga and M. Kanai, Angew. Chem., Int. Ed., 2013, 52, 2207 CrossRef PubMed.
-
(a) D. Zhao, J. H. Kim, L. Stegemann, C. A. Strassert and F. Glorius, Angew. Chem., Int. Ed., 2015, 54, 4508 CrossRef PubMed;
(b) T. Gensch, S. VasquezCespedes, D.-G. Yu and F. Glorius, Org. Lett., 2015, 17, 3714 CrossRef PubMed;
(c) D.-G. Yu, T. Gensch, F. de Azambuja, S. Vasquez-Céspedes and F. Glorius, J. Am. Chem. Soc., 2014, 136, 17722 CrossRef PubMed;
(d) A. Lerchen, T. Knecht, M. Koy, C. G. Daniliuc and F. Glorius, Chem.–Eur. J., 2017, 23, 12149 CrossRef PubMed.
-
(a) J. Li and L. Ackermann, Angew. Chem., Int. Ed., 2015, 54, 8551 CrossRef PubMed;
(b) J. Li and L. Ackermann, Angew. Chem., Int. Ed., 2015, 54, 3635 CrossRef PubMed;
(c) M. Moselage, N. Sauermann, J. Koeller, W. Liu, D. Gelman and L. Ackermann, Synlett, 2015, 1596 Search PubMed;
(d) H. Wang, J. Koeller, W. Liu and L. Ackermann, Chem.–Eur. J., 2015, 21, 15525 CrossRef PubMed;
(e) N. Sauermann, M. J. Gonzalez and L. Ackermann, Org. Lett., 2015, 17, 5316 CrossRef PubMed;
(f) R. Mei, J. Loup and L. Ackermann, ACS Catal., 2016, 6, 793 CrossRef;
(g) D. Zell, M. Bursch, V. Müller, S. Grimme and L. Ackermann, Angew. Chem., Int. Ed., 2017, 56, 10378 CrossRef PubMed.
-
(a) J. R. Hummel and J. A. Ellman, Org. Lett., 2015, 17, 2400 CrossRef PubMed;
(b) J. R. Hummel and J. A. Ellman, J. Am. Chem. Soc., 2015, 137, 490 CrossRef PubMed.
-
(a) A. B. Pawar and S. Chang, Org. Lett., 2015, 17, 660 CrossRef PubMed;
(b) P. Patel and S. Chang, ACS Catal., 2015, 5, 853 CrossRef;
(c) J. Park and S. Chang, Angew. Chem., Int. Ed., 2015, 54, 14103 CrossRef PubMed.
-
(a) M. Sen, B. Emayavaramban, N. Barsu, J. Richard Premkumar and B. Sundararaju, ACS Catal., 2016, 6, 2792 CrossRef;
(b) N. Barsu, M. Sen, J. R. Premkumar and B. Sundararaju, Chem. Commun., 2016, 52, 1338 RSC;
(c) M. Sen, D. Kalsi and B. Sundararaju, Chem.–Eur. J., 2015, 21, 15529 CrossRef PubMed;
(d) N. Barsu, M. A. Rahman, M. Sen and B. Sundararaju, Chem.–Eur. J., 2016, 22, 9135 CrossRef PubMed.
-
(a) S. Prakash, K. Muralirajan and C. H. Cheng, Angew. Chem., Int. Ed., 2016, 55, 1844 CrossRef PubMed;
(b) K. Muralirajan, R. Kuppusamy, S. Prakash and C. H. Cheng, Adv. Synth. Catal., 2016, 358, 774 CrossRef.
-
(a) Y. Liang, Y.-F. Liang, C. Tang, Y. Yuan and N. Jiao, Chem.–Eur. J., 2015, 21, 16395 CrossRef PubMed;
(b) Z.-Z. Zhang, B. Liu, C.-Y. Wang and B.-F. Shi, Org. Lett., 2015, 17, 4094 CrossRef PubMed;
(c) X.-G. Liu, S.-S. Zhang, C.-Y. Jiang, J.-Q. Wu, Q. Li and H. Wang, Org. Lett., 2015, 17, 5404 CrossRef PubMed;
(d) L. Kong, S. Yu, X. Zhou and X. Li, Org. Lett., 2016, 18, 588 CrossRef PubMed;
(e) Y. Liang and N. Jiao, Angew. Chem., Int. Ed., 2016, 55, 4035 CrossRef PubMed;
(f) L. Kong, S. Yu, G. Tang, H. Wang, X. Zhou and X. Li, Org. Lett., 2016, 18, 3802 CrossRef PubMed;
(g) F. Wang, H. Wang, Q. Wang, S. Yu and X. Li, Org. Lett., 2016, 18, 1306 CrossRef PubMed;
(h) F. Wang, L. Jin, L. Kong and X. Li, Org. Lett., 2017, 19, 1812 CrossRef PubMed.
-
(a) Y. Park, K. T. Park, J. G. Kim and S. Chang, J. Am. Chem. Soc., 2015, 137, 4534 CrossRef PubMed;
(b) V. Bizet and C. Bolm, Eur. J. Org. Chem., 2015, 2015, 2854 CrossRef;
(c) Y. Park, S. Jee, J. G. Kim and S. Chang, Org. Process Res. Dev., 2015, 19, 1024 CrossRef;
(d) V. Bizet, L. Buglioni and C. Bolm, Angew. Chem., Int. Ed., 2014, 53, 5639 CrossRef PubMed;
(e) L. Li and G. Wang, Tetrahedron, 2018, 74, 4188 CrossRef;
(f) P. W. Tan, A. M. Mak, M. B. Sullivan, D. J. Dixon and J. Seayad, Angew. Chem., Int. Ed., 2017, 56, 16550 CrossRef PubMed;
(g) C. L. Zhong, B. Y. Tang, P. Yin, Y. Chen and L. He, J. Org. Chem., 2012, 77, 4271 CrossRef PubMed;
(h) E. Eibler, J. Käsbauer, H. Pohl and J. Sauer, Tetrahedron Lett., 1987, 28, 1097 CrossRef;
(i) W. J. Middleton, J. Org. Chem., 1983, 48, 3845 CrossRef;
(j) J. Sauer and K. K. Mayer, Tetrahedron Lett., 1968, 9, 319 CrossRef.
-
(a) P. Patel and S. Chang, ACS Catal., 2015, 5, 853 CrossRef;
(b) Y. Wu, Z. Chen, Y. Yang, W. Zhu and B. Zhou, J. Am. Chem. Soc., 2018, 140, 42 CrossRef PubMed;
(c) A. D. Sadiq, X. Chen, N. Yan and J. Sperry, ChemSusChem, 2018, 11, 532 CrossRef PubMed;
(d) M. J. Hülsey, H. Yang and N. Yan, ACS Sustainable Chem. Eng., 2018, 6, 5694 CrossRef.
- E. M. Simmons and J. F. Hartwig, Angew. Chem., Int. Ed., 2012, 51, 3066 CrossRef PubMed.
Footnote |
† Electronic supplementary information (ESI) available: Data for new compounds and experimental procedures. See DOI: 10.1039/c8ra06716c |
|
This journal is © The Royal Society of Chemistry 2018 |
Click here to see how this site uses Cookies. View our privacy policy here.