DOI:
10.1039/C8RA06619A
(Paper)
RSC Adv., 2018,
8, 30076-30079
Highly reactive 2-deoxy-2-iodo-D-allo and D-gulo pyranosyl sulfoxide donors ensure β-stereoselective glycosylations with steroidal aglycones†
Received
6th August 2018
, Accepted 11th August 2018
First published on 24th August 2018
Abstract
The preparation of well-defined D-xylo and D-ribo glycosides represents a synthetic challenge due to the limited configurational availability of starting materials and the laborious synthesis of homogeneous 2-deoxy-β-glycosidic linkages, in particular that of the sugar-steroid motif, which represents the “stereoselective determining step” of the overall synthesis. Herein we describe the use of 2-deoxy-2-iodo-glycopyranosyl sulfoxides accessible from widely available D-xylose and D-ribose monosaccharides as privileged glycosyl donors that permit activation at very low temperature. This ensures a precise kinetic control for a complete 1,2-trans stereoselective glycosylation of particularly challenging steroidal aglycones.
Introduction
2-Deoxy- and 2,6-dideoxy-β-glycosides are common architectures present in many biologically active ingredients such as antibiotics, appetite suppressants, and nucleosides.1 These deoxyglycosides, and especially cardiac glycosides (e.g., cardenolides N-1 from Nerium oleander or those from chrysomelid beetles,3 and helveticoside4), are usually composed of uncommon glycosyl moieties including D-ribo and D-xylo-configured pyranoses (Fig. 1). While these glycoconjugates, with the general structure [sugar]n–aglycone, are nicely produced in nature, most of the chemical glycosylation approaches5 for their preparation are mainly focused on the sugar–sugar motif but are still inefficient for the β-stereoselective synthesis of the sugar-steroid portion. In addition, the β-stereoselectivity is typically better for the construction of the sugar–sugar motif (up to only β) compared to that of the sugar-steroid fragment (up to 9
:
1 β/α) and thus, the latter glycosylation step represents the overall “stereoselective determining step” in 2-deoxy and 2,6-dideoxyglycoconjugates featuring such particular configurations.5,6 Our group has developed an indirect7 synthetic approach for the stereoselective synthesis of 2-deoxy- and 2,6-dideoxy-2-iodoglycosides that utilizes 2-deoxy-2-iodo-1-thioglycoside donors, being particularly effective for the production of β-D-allo and β-D-gulo pyranosides.2,8–10 The resulting configuration is predefined by the starting furanose and thus, D-ribo and D-xylo structures serve as configurational templates for D-allo and D-gulo pyranosides, respectively. Our findings determined the key β-selective glycosylation step is kinetically-controlled and the presence of iodine favours the stereoselective formation of a 1,2-trans glycoside via the least energetic transition state upon preferential nucleophilic attack to the oxonium intermediate 3H4. This is consistent with the Felkin–Anh–Eisenstein 1,2-induction model with stabilizing hyperconjugative interactions between
and σC–OR (Scheme 1). According to most current models,11 the stereoselectivity is determined by the interplay between (a) the ground-state conformational preferences of oxocarbenium intermediates (4H3 vs. 3H4) in which electronegative substituents such as I and OBn prefer a pseudo-axial disposition due to stabilizing electrostatic and/or hyperconjugative interactions (e.g., between σC–I and
) and (b) the relative reactivity of each conformer under the SN1 paradigm, according to a Curtin–Hammett kinetics scenario. In this context, while glycosylations of 2-deoxy-2-iodo-1-thioglycosides with sugar acceptors proceed at ca. −40 °C and provided reasonably good selectivities (up to 16
:
1 β/α), we observed a reduction to 8
:
1 β/α with steroidal aglycones (Scheme 1).8 We reasoned that prior oxidation of the 1-thiophenyl donor to a glycosyl sulfoxide (SPh → S(O)Ph) would enhance its reactivity enabling activation at lower temperatures.12,13 Hence, iodine control will perform better at lower temperatures restoring the kinetic control in challenging glycosylations as those using steroidal aglycones, favouring the selective formation of β-glycosides.
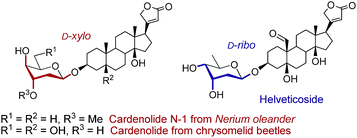 |
| Fig. 1 Naturally occurring 2-deoxy and 2,6-dideoxy-β-glycosides with “rare” D-xylo and D-ribo configurations. | |
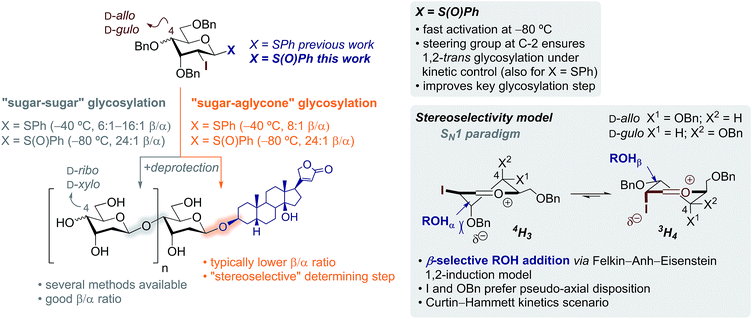 |
| Scheme 1 Scope and limitations of the stereoselective synthesis of β-steroidal glycosides of D-ribo and D-xylo configurations – using sulfoxides to improve key “sugar–aglycone” glycosylation step. | |
Results and discussion
Preliminary oxidation studies of 1 with mCPBA (in CHCl3)13 and Selectfluor™ (in CH3CN)14 revealed the high reactivity of the resulting sulfoxide 2, which evaded isolation due to decomposition. The best protocol used mCPBA as the sole oxidant in CH2Cl2 from −80 °C to −50 °C, followed by neutralization of the residual benzoic acid with NaHCO3, removal of the precipitate, and conducting the following glycosylation in sequence (Table 1). Thus, dichloromethane perfectly combines chemical inertness towards oxidants, good oxidation rate of sulfides using peroxy acids at the low temperatures necessitated to avoid decomposition,15 and good β-selective properties in the subsequent glycosylation reaction with sulfoxides (up to 3
:
1 β/α ratio with Bn as protecting groups).13 To verify the formation of 2, oxidation was monitored by 1H NMR in CD2Cl2 (Scheme 2). The signal peak at 5.10 ppm corresponding to the H-1 proton of the predominant 1β-anomer was gradually converted to two new doublets at 5.14 and 5.02 ppm, tentatively assigned to 2β(S) and 2β(R), respectively with a 88
:
12 dr. Although the signal of 2β(S) was gradually shifted upfield upon warming from −70 to −15 °C, the Δδ of ca. 0.2 ppm between the two stereoisomers was in accordance with previously reported diastereomeric sulfoxides.16
Table 1 Glycosylation scope (SPh vs. S(O)Ph)a
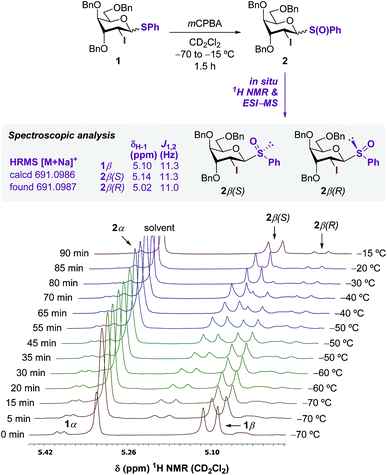 |
| Scheme 2 VT-NMR monitoring of the oxidation of 1. | |
The identity of 2 was further confirmed by high-resolution mass spectrometry analysis (HRMS). Next, glycosylation was explored comparing the selectivities obtained for the activation of 1 and 2 (Table 1). Standard glycosylation using 1-thiophenyl donor 1 resulted in excellent β-stereoselectivities with primary 4-nitro-benzyl alcohol 3a (up to 30
:
1 β/α) and secondary methyl glucoside alcohol 3b (16
:
1 β/α) (entries 1 and 3).8 In contrast, employing cholesterol 3c as representative steroidal acceptor substantially decreased the selectivity to 8
:
1 β/α ratio and the thermodynamically more stable α-anomer could not be separated from its β-counterpart. Alternatively, oxidation of 1 followed by activation using the Tf2O/DTBMP system at −80 °C afforded the corresponding glycosides in very short reaction times and good yields (up to 80%). Glycosylation with primary benzylic 3a and secondary sugar acceptors 3b slightly improved the selectivity up to 40
:
1 β/α (entries 2 and 4). To our delight, glycosylations using cholesterol 3c reached comparable levels of stereocontrol (up to 21
:
1 β/α) only when sulfoxide was used as the glycosyl donor (entries 5 and 6). Thus, merging the excellent stereodirecting group properties of I8,9 and the lower reaction temperature enabled by the reactive sulfoxide ensured excellent kinetic control with challenging steroidal aglycones.
Finally, the unique combination of oxidation/glycosylation sequence of this strategy was utilized for the synthesis of 2-deoxy-2-iodo-β-pyranosides 7 and 8 with high stereoselectivities (>22
:
1 β/α) and good yields (up to 64%) using the steroidal acceptor digitoxigenin 6 and D-gulo- and D-allo-1-thiopyranosides 1 and 5a as glycosyl donors (Scheme 3). The stereochemistry of the C-4 substituent had little effect on the selectivity although the slight improvement in the D-allo configuration may be explained by the less entropically disfavored β-transition state resulting from the stabilizing pseudoaxial positioning of OBn in the 3H4 conformer (Scheme 1).11,17 Elaboration of 7 and 8 under conventional deiodination and debenzylation conditions2 afforded final 2-deoxy cardiac glycosides 9 and 10 in excellent yields (up to 95%).
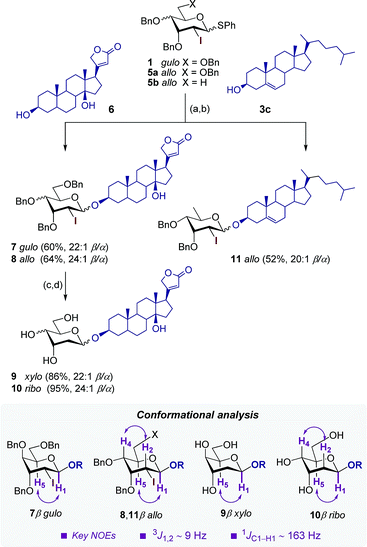 |
| Scheme 3 Synthesis of 2-deoxy- and 2,6-dideoxy-2-iodo-β-pyranosides 7, 8 and 11 and deprotection steps to digitoxigenyl 2-deoxy-β-D-xylo and D-ribo cardiac glycosides 9 and 10. Reagents and conditions: (a) mCPBA, 4 Å MS, CH2Cl2 from −80 °C to −40 °C, 30 min; (b) 3,6, DTBMP, 4 Å MS, Tf2O, −80 °C, 30 min; (c) Bu3SnH, Et3B, toluene, rt, 1 h; (d) H2 (1 atm), 10% Pd/C, 1 : 1 EtOAc/MeOH, 0 °C, 1–3 h. | |
Likewise, cholesterol 3c was subjected to the same oxidation/glycosylation sequence with the more challenging 2,6-dideoxy glycosyl donor 5b to afford 2,6-dideoxy-2-iodo-D-allo derivative 11 with good stereoselectivity (20
:
1 β/α)2,9d and moderate overall yield (52%). Final products 9 and 10 as well as their precursors 7, 8 and 11 adopted a 4C1 conformation as determined by NOE experiments and the analysis of diagnostic coupling constants (3J1,2 ∼ 9 Hz and 1JC1–H1 ∼ 163 Hz).
Conclusions
In conclusion, the present work upgrades the previous reported methodology (using 1-thioglycosides) for the stereoselective synthesis of 2-deoxy-β-glycosides with D-ribo and D-xylo configurations, improving the overall β-control using challenging steroidal aglycones. The enhanced reactivity of glycosyl sulfoxides and the presence of an equatorial steering iodine permitted the precise formation of complex 2-deoxy-β-glycosides after removal of the temporary directing element. We expect that the present protocol will find broad application in the chemical synthesis of steroidal glycosides for the medicinal research field.
Conflicts of interest
There are no conflicts to declare.
Acknowledgements
We thank the Spanish Government-MINECO and the national agency of investigation-AEI (CTQ2017-89750-R and CTQ2017-90088-R), the European Regional Development Fund, and the Universitat Rovira i Virgili (Martí Franquès Research Fellowship Programme to J. M. and D. C.) for financial support. We also thank Arnau R. Rubio for preliminary experiments. O. B. is a Ramón y Cajal Fellow (RYC-2015-17705).
Notes and references
-
(a) B. Heasley, Chem.–Eur. J., 2012, 18, 3092 CrossRef PubMed;
(b) J. Zhang, H. Shi, Y. Ma and B. Yu, Chem. Commun., 2012, 48, 8679 RSC;
(c) R. M. De Lederkremer and C. Marino, Adv. Carbohydr. Chem. Biochem., 2008, 61, 143 CrossRef;
(d) H. P. Albrecht, in Naturally Occurring Glycosides, ed. R. Ikan, Wiley, Chichester, 1999 Search PubMed;
(e) A. C. Weymouth-Wilson, Nat. Prod. Rep., 1997, 14, 99 RSC;
(f) L. D. Nord, N. K. Dalley, P. A. McKernan and R. K. Robins, J. Med. Chem., 1987, 30, 1044 CrossRef PubMed;
(g) A. Kirschning, A. F.-W. Bechthold and J. Rohr, Top. Curr. Chem., 1997, 188, 1 CrossRef.
- J. Mestre, M. I. Matheu, Y. Díaz, S. Castillón and O. Boutureira, J. Org. Chem., 2017, 82, 3327 CrossRef PubMed.
- D. Daloze, F. Broeders, J.-C. Braekman, J. Araujo and J. M. Pasteels, Biochem. Syst. Ecol., 1995, 23, 113 CrossRef.
- T. Nakamura, Y. Goda, S. Sakai, K. Kondo, H. Akiyama and M. Toyoda, Phytochemistry, 1998, 49, 2097 CrossRef PubMed.
-
(a) C. S. Bennett and M. C. Galan, Chem. Rev., 2018 DOI:10.1021/acs.chemrev.7b00731;
(b) J. Zeng, Y. Xu, H. Wang, L. Meng and Q. Wan, Sci. China: Chem., 2017, 60, 1162 CrossRef;
(c) S. Medina and M. C. Galan, in Carbohydrate Chemistry, Royal Society of Chemistry, Cambridge, 2016, 41, p. 59 Search PubMed;
(d) A. Borovika and P. Nagorny, J. Carbohydr. Chem., 2012, 31, 255 CrossRef;
(e) D. Hou and T. L. Lowary, Carbohydr. Res., 2009, 344, 1911 CrossRef PubMed;
(f) C. H. Marzabadi and R. W. Franck, Tetrahedron, 2000, 56, 8385 CrossRef.
-
(a) J. Zeng, G. Sun, R. Wang, S. Zhang, S. Teng, Z. Liao, L. Menga and Q. Wan, Org. Chem. Front., 2017, 4, 2450 RSC;
(b) K. N. Baryal, S. Adhikari and J. Zhu, J. Org. Chem., 2013, 78, 12469 CrossRef PubMed;
(c) Y. Ma, Z. Li, H. Shi, J. Zhang and B. Yu, J. Org. Chem., 2011, 76, 9748 CrossRef PubMed;
(d) H. Tanaka, A. Yoshizawa and T. Takahashi, Angew. Chem., Int. Ed., 2007, 46, 2505 CrossRef PubMed;
(e) M. Zhou and G. A. O'Doherty, J. Org. Chem., 2007, 72, 2485 CrossRef PubMed;
(f) K. Toshima, Carbohydr. Res., 2006, 341, 1282 CrossRef PubMed;
(g) M. Zhou and G. A. O'Doherty, Org. Lett., 2006, 8, 4339 CrossRef PubMed;
(h) F. E. McDonald and K. S. Reddy, Angew. Chem., Int. Ed., 2001, 40, 3653 CrossRef;
(i) F. E. McDonald, K. S. Reddy and Y. Díaz, J. Am. Chem. Soc., 2000, 122, 4304 CrossRef;
(j) K. Wiesner, T. Y. R. Tsai and H. Jin, Helv. Chim. Acta, 1985, 68, 300 CrossRef.
-
(a) S. K. Battina and S. Kashyap, Tetrahedron Lett., 2016, 57, 811 CrossRef;
(b) H. Wang, J. Tao, X. Cai, W. Chen, Y. Zhao, Y. Xu, W. Yao, J. Zeng and Q. Wan, Chem.–Eur. J., 2014, 20, 17319 CrossRef PubMed;
(c) M. De Castro and C. H. Marzabadi, Tetrahedron, 2010, 66, 3395 CrossRef;
(d) T. B. Durham and W. R. Roush, Org. Lett., 2003, 5, 1871 CrossRef PubMed;
(e) P. Y. Chong and W. R. Roush, Org. Lett., 2002, 4, 4523 CrossRef PubMed.
- M. A. Rodríguez, O. Boutureira, X. Arnés, Y. Díaz, M. I. Matheu and S. Castillón, J. Org. Chem., 2005, 70, 10297 CrossRef PubMed.
-
(a) A. Kövér, O. Boutureira, M. I. Matheu, Y. Díaz and S. Castillón, J. Org. Chem., 2014, 79, 3060 CrossRef PubMed;
(b) O. Boutureira, M. I. Matheu, Y. Díaz and S. Castillón, RSC Adv., 2014, 4, 19794 RSC;
(c) I. Cobo, M. I. Matheu, S. Castillón, O. Boutureira and B. G. Davis, Org. Lett., 2012, 14, 1728 CrossRef PubMed;
(d) M. A. Rodríguez, O. Boutureira, M. I. Matheu, Y. Díaz and S. Castillón, Eur. J. Org. Chem., 2007, 2470 CrossRef;
(e) M. A. Rodríguez, O. Boutureira, M. I. Matheu, Y. Díaz, S. Castillón and P. H. Seeberger, J. Org. Chem., 2007, 72, 8998 CrossRef PubMed;
(f) O. Boutureira, M. A. Rodríguez, M. I. Matheu, Y. Díaz and S. Castillón, Org. Lett., 2006, 8, 673 CrossRef PubMed.
-
(a) O. Boutureira, M. A. Rodríguez, Y. Díaz, M. I. Matheu and S. Castillón, Carbohydr. Res., 2010, 345, 1041 CrossRef PubMed;
(b) O. Boutureira, M. A. Rodríguez, D. Benito, M. I. Matheu, Y. Díaz and S. Castillón, Eur. J. Org. Chem., 2007, 3564 CrossRef;
(c) O. Boutureira, M. I. Matheu, Y. Díaz and S. Castillón, Carbohydr. Res., 2007, 342, 736 CrossRef PubMed.
-
(a) A. Martin, A. Arda, J. Désiré, A. Martin-Mingot, N. Probst, P. Sinaÿ, J. Jiménez-Barbero, S. Thibaudeau and Y. Blériot, Nat. Chem., 2016, 8, 186 CrossRef PubMed;
(b) M. T. C. Walvoort, J. Dinkelaar, L. J. van den Bos, G. Lodder, H. S. Overkleeft, J. D. C. Codée and G. A. van der Marel, Carbohydr. Res., 2010, 345, 1252 CrossRef PubMed;
(c) M. Heuckendorff, C. M. Pedersen and M. Bols, Chem.–Eur. J., 2010, 16, 13982 CrossRef PubMed;
(d) D. Hou, H. A. Taha and T. L. Lowary, J. Am. Chem. Soc., 2009, 131, 12937 CrossRef PubMed;
(e) M. G. Beaver, S. B. Billings and K. A. Woerpel, J. Am. Chem. Soc., 2008, 130, 2082 CrossRef PubMed;
(f) D. M. Smith and K. A. Woerpel, Org. Biomol. Chem., 2006, 4, 1195 RSC;
(g) F. Bravo, A. Viso, E. Alcázar, P. Molas, C. Bo and S. Castillón, J. Org. Chem., 2003, 68, 686 CrossRef PubMed.
-
(a) B. Yang, W. Yang, S. Ramadan and X. Huang, Eur. J. Org. Chem., 2018, 1075 CrossRef PubMed;
(b) W. Yang, B. Yang, S. Ramadan and X. Huang, Beilstein J. Org. Chem., 2017, 13, 2094 CrossRef PubMed;
(c) M. A. Fascione, R. Brabham and W. B. Turnbull, Chem.–Eur. J., 2016, 22, 3916 CrossRef PubMed;
(d) M. C. Aversa, A. Barattucci and P. Bonaccorsi, Tetrahedron, 2008, 64, 7659 CrossRef.
- D. Kahne, S. Walker, Y. Cheng and D. Van Engen, J. Am. Chem. Soc., 1989, 111, 6881 CrossRef.
- S. P. Vincent, M. D. Burkart, C.-Y. Tsai, Z. Zhang and C.-H. Wong, J. Org. Chem., 1999, 64, 5264 CrossRef.
- R. Curci, R. A. DiPrete, J. O. Edwards and G. Modena, J. Org. Chem., 1970, 35, 740 CrossRef.
-
(a) T. Taniguchi, M. Asahata, A. Nasu, Y. Shichibu, K. Konishi and K. Monde, Chirality, 2016, 28, 534 CrossRef PubMed;
(b) J. F. Moya-López, E. Elhalem, R. Recio, E. Álvarez, I. Fernández and N. Khiar, Org. Biomol. Chem., 2015, 13, 1904 RSC.
-
(a) N. Aiguabella, M. C. Holland and R. Gilmour, Org. Biomol. Chem., 2016, 14, 5534 RSC;
(b) N. Santschi and R. Gilmour, Eur. J. Org. Chem., 2015, 6983 CrossRef;
(c) E. Durantie, C. Bucher and R. Gilmour, Chem.–Eur. J., 2012, 18, 8208 CrossRef PubMed;
(d) C. Bucher and R. Gilmour, Angew. Chem., Int. Ed., 2010, 49, 8724 CrossRef PubMed;
(e) J. A. C. Romero, S. A. Tabacco and K. A. Woerpel, J. Am. Chem. Soc., 2000, 122, 168 CrossRef;
(f) R. J. Woods, C. W. Andrews and J. P. Bowen, J. Am. Chem. Soc., 1992, 114, 850 CrossRef;
(g) R. J. Woods, C. W. Andrews and J. P. Bowen, J. Am. Chem. Soc., 1992, 114, 859 CrossRef.
Footnote |
† Electronic supplementary information (ESI) available. See DOI: 10.1039/c8ra06619a |
|
This journal is © The Royal Society of Chemistry 2018 |