DOI:
10.1039/C8RA06215C
(Paper)
RSC Adv., 2018,
8, 32597-32600
KI-catalyzed oxidative cyclization of α-keto acids and 2-hydrazinopyridines: efficient one-pot synthesis of 1,2,4-triazolo[4,3-a]pyridines†
Received
23rd July 2018
, Accepted 15th September 2018
First published on 21st September 2018
Abstract
A one-pot approach to substituted 1,2,4-triazolo[4,3-a]pyridines has been developed that is based on a KI-catalyzed oxidative cyclization of α-keto acids and 2-hydrazinopyridines. This transition-metal-free procedure was highly efficient and shows good economical and environmental advantages.
Introduction
Triazolo pyridines represent an important class of nitrogen containing fused-ring heterocycles, which exist as core scaffolds in many natural products and bioactive molecules.1 Among them, 1,2,4-triazolo[4,3-a]pyridines display versatile biological activities and possess attractive applications in the fields of pharmaceutical and pesticide chemistry.2 For example, functionalized 1,2,4-triazolo[4,3-a]pyridines have been investigated as human 11β-hydroxysteroid dehydrogenase-type 1 (ref. 2a) (Fig. 1a) and P38α mitogen-activated (MAP) kinase inhibitors2b (Fig. 1b), as well as an effective antimalarial agent2c (Fig. 1c). Moreover, 1,2,4-triazolo[4,3-a]pyridines also have found wide applications in the fields of coordination chemistry and material chemistry.3 As a consequence, the development of effective and practical methods for the construction of substituted 1,2,4-triazolo[4,3-a]pyridines has attracted considerable interests. Generally, classic methods for the synthesis of 1,2,4-triazolo[4,3-a]pyridines including cyclodehydration of acylated 2-hydrazinopyridines4 and oxidative cyclization of 2-pyridylhydrazones.5 In recent years, some elegant one-pot, two-steps synthesis of 1,2,4-triazolo[4,3-a]pyridines were developed.6 Chang and co-workers reported condensation of aldehydes and 2-hydrazinopyridine in EtOH and followed by iodine-induced oxidative cyclization.6a Very recently, Reddy developed a I2/DMSO system to oxidize α-aryl methyl ketones to give aldehydes, sequential cyclization with 2-hydrazinopyridines.6b However, a really one-pot synthesis of 1,2,4-triazolo[4,3-a]pyridines is still seldom reported.7 Thus, the development of practical one-pot synthesis of 1,2,4-triazolo[4,3-a]pyridines is highly desirable.
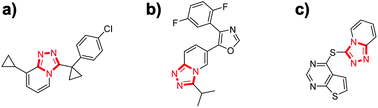 |
| Fig. 1 Selected examples of functional 1,2,4-triazolo[4,3-a]pyridines. (a) Inhibitor of 11β-HSD-1, (b) inhibitor of P38a MAP kinase, (c) antimalarial agent. | |
Results and discussion
Due to the unique variable-valent and environment friendly natures of iodides, a variety of useful iodide-containing catalytic systems such as XI/TBHP (X = I, Na, K, nBu4N…), I2/H2O2, ArI/mCPBA and NaI/tBuOCl have been developed rapidly in recent years.8 In this respect, XI/TBHP systems have found wide applications in the preparation of functionalized heterocyclic compounds.9 Wang and co-workers developed a NIS/TBHP-mediated intermolecular oxidative amination providing substituted quinazolines in high yields.9a Kalita and co-workers reported an I2/TBHP-induced synthesis of 4,3-fused 1,2,4-triazoles via azomethine imine 1,3-dipolar cycloaddition with aromatic N-heterocycles.9b Although iodine-promoted procedures have been developed for the synthesis of 1,2,4-triazolo[4,3-a]pyridines, excess amount of iodine as well as two-steps are required, which highly increased the cost of the method. As part of our studies on transition-metal-free oxidative cyclization reactions,10 we previously found hydrazides hardly tolerated under oxidative conditions, especially at high reaction temperatures. Aiming at these problems, we surmise that a quick transformation of 2-hydrazinopyridines with active carbonyl compounds may inhibit the production of the oxidative byproducts, and sequentially realize the construction of 1,2,4-triazolo[4,3-a]pyridines. Herein, we demonstrate an efficient oxidative cyclization of α-keto acids and 2-hydrazinopyridines via KI/TBHP system which afforded good yields of substituted 1,2,4-triazolo[4,3-a]pyridines in one-pot.
In the initial study, a screen of the active carbonyl compounds was preceded. A one-pot synthesis of 1,2,4-triazolo[4,3-a]pyridines requires the condensation reaction rate k1 much larger than the oxidative cyclization reaction rate k2 and the functional group easy to be removed. Therefore, some electron-withdrawing-groups were tested in the reaction such as –CO2H, –COMe, –CHO, –COPh, –CN and –CO2Me, which enhanced the electrophilicity of the α-carbonyl group and could be removed by C–C bond cleavage under oxidative conditions (Fig. 2).11 After several trials, we were delight to find that the desired 1,2,4-triazolo[4,3-a]pyridine 4a could be obtained in 82% yield via oxidative cyclization of α-keto acid 1a and 2-hydrazinopyridine 2a in the presence of 20 mol% of KI, 2 equiv. of TBHP and 2 equiv. of Na2CO3 in 1,4-dioxane at 130 °C for 12 h.
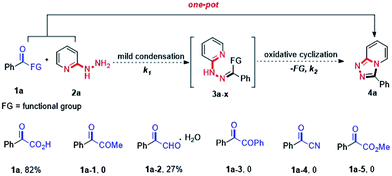 |
| Fig. 2 Screened active carbonyl compounds. | |
Around the optimized conditions, the reaction parameters were varied and the results were summarized in Table 1. Further investigation of iodides revealed that NaI, TBAI and I2 were less reactive than KI (entries 2–4). Subsequently, changing the oxidants into others such as H2O2, DTBP and K2S2O8 would decrease the outcomes of the reaction (entries 5–7). Next, the influence of the bases was studied. The product yields were highly improved when Na2CO3 and K2CO3 were employed as bases (entries 8, 9). Other bases such as TEA showed little poor performance (entry 10).
Table 1 Optimization of the reaction conditionsa

|
Entry |
Variation from standard conditions |
Yieldb |
Reaction conditions: 1a (0.5 mmol), 2a (0.5 mmol), KI (20 mol%), TBHP (70% aqueous solution, 1 mmol) and Na2CO3 (1 mmol) in 1,4-dioxane (2 mL) at 130 °C for 12 h. Isolated yield. |
1 |
None |
82 |
2 |
NaI, instead of KI |
68 |
3 |
TBAI, instead of KI |
56 |
4 |
I2, instead of KI |
55 |
5 |
H2O2, instead of TBHP |
37 |
6 |
DTBP, instead of TBHP |
0 |
7 |
K2S2O8, instead of TBHP |
0 |
8 |
K2CO3, instead of Na2CO3 |
63 |
9 |
Without Na2CO3 |
41 |
10 |
TEA, instead of Na2CO3 |
18 |
To demonstrate the generality of this one-pot 1,2,4-triazolo[4,3-a]pyridine synthesis reaction, we then set out to explore the substrate scope. As shown in Scheme 1, a wide range of aryl α-keto acids were employed to react with 2-hydrazinopyridine 2a under the optimized conditions. Aryl α-keto acids with electron-donating groups (–Me, –OMe) on the aryl ring proceeded smoothly to afford the desired products 4 in moderate to good yields (4b–4i, 40–83%). However, ortho-methyl substituted substrate gave a comparatively low yield of 4d; this might be due to the steric hindrance of ortho-methyl group. Halogen substituted (4-F, 3-Cl, 4-Cl, 4-Br) aryl α-keto acids were well-tolerated in this tandem reaction (4j–4m), which could be further derivatized in classic cross-coupling reactions. Other electron-withdrawing groups such as 4-CF3 and 4-Ph were also compatible under the typical conditions, to produce the desired product in 42% and 71% yield (4o, 4x), respectively. Moreover, the ring-fused and heterocyclic substrates also reacted smoothly to deliver the corresponding products (4p–4r) in moderate yields. However, the system was not applicable for the cyclization of 2-oxopropanoic acid with 2a. This could be attributed to a weaker electrophilicity of C2-carbonyl of 2-oxopropanoic acid in compared with the aryl α-keto acids. Next, an array of 2-hydrazinopyridine derivatives were investigated. To our satisfaction, the developed method was successfully applied to different Me–, Cl–, Br– and CF3– substituted 2-hydrazinopyridines, providing the desired products in 35–74% yields (4t–4x). It was noted that 6-Me substituted 2-hydrazinopyridines gave a lower yield of product, probably due to the steric hindrance. However, steric effects had a little impact on the reaction 2-Cl and 2-Br substituted 2-hydrazinopyridines, which gave yields similar to the para-substituted ones. Furthermore, 2-hydrazinoquinoline derivatives were also compatible with the optimized conditions, affording the corresponding products 4y and 4z in 62% and 70% yield, respectively. Additionally, the model reaction can be easily performed on 10 mmol scale, producing 1.40 g (72% yield) of 4a.
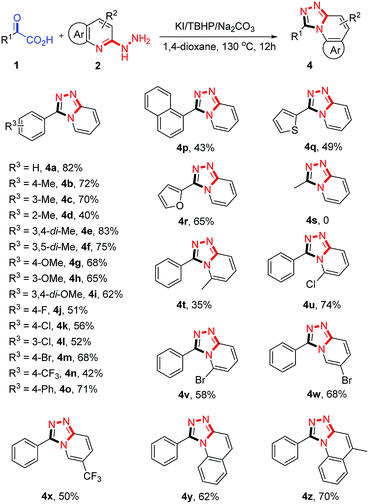 |
| Scheme 1 Substrate scope. | |
To gain further insight into the reaction mechanism, some control experiments were carried out. Addition of 2 equiv. of TEMPO into the model reaction, which is a known radical scavenger, a slightly decreased yield of product 4a was obtained (Scheme 2a). The results suggested that the reaction might follow a hypervalent iodine-catalytic mechanism than a radical pathway. When the reaction was preceded for 2 min, the condensation product 3a was isolated in 48% yield. As expected, further reaction of 3a under standard conditions furnished the desired product 4a in 91% yield. These observations identified that 3a was a key intermediate of the reaction.
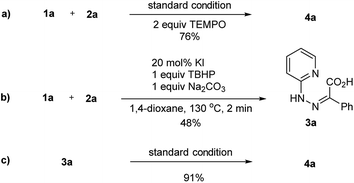 |
| Scheme 2 Mechanistic investigation. | |
Based on the above experiments and previous studies,8,9,12 a plausible mechanism is proposed in Scheme 3. Hypoiodate A is initially generated from oxidation of iodide by tBuO2H.9b,12 Then, oxidation of the in situ generated intermediate 3a by A forms B, which could be further transferred to C through intermolecular nucleophilic cyclization.9 Finally, decarboxylation aromatization of C gives 4a and completed the catalytic circle of iodide. Bases may affect the key steps B to C, thus, enhancing the reaction outcomes.8a,e
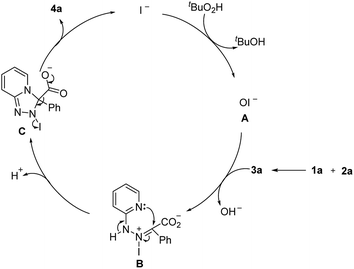 |
| Scheme 3 Proposed mechanism. | |
Conclusions
In summary, we have developed a one-pot method for the synthesis of 1,2,4-triazolo[4,3-a]pyridines using KI as the catalyst and tBuO2H as the terminal oxidant. This transition-metal-free methodology shows good economical and environmental advantages. Furthermore, the mildness of this approach also makes it appealing for further application in organic synthesis.
Conflicts of interest
There are no conflicts to declare.
Acknowledgements
This work was supported by the China Postdoctoral Science Foundation (2017M613183), the National Undergraduate Training Programs for Innovation and Entrepreneurship (201610721010), the Shaanxi Provincial Key Laboratory Project (13JS006, 16JS009), and Baoji University of Arts and Sciences (ZK15046, 2017dc107).
Notes and references
-
(a) M. S. Krieger, R. N. Yoder and R. Gibson, J. Agric. Food Chem., 2000, 48, 3710 CrossRef PubMed;
(b) M. K. Khera, I. A. Cliffe, T. Mathur and O. Prakash, Bioorg. Med. Chem. Lett., 2011, 21, 2887 CrossRef PubMed;
(c) P. Liscio, A. Carotti, S. Asciutti, T. Karlberg, D. Bellocchi, L. Llacuna, A. Macchiarulo, S. A. Aaronson, H. Schüler, R. Pellicciari and E. Camaioni, J. Med. Chem., 2014, 57, 2807 CrossRef PubMed;
(d) F. J. R. Rombouts, G. Tresadern, P. Buijnsters, X. Langlois, F. Tovar, T. B. Steinbrecher, G. Vanhoof, M. Somers, J.-I. Andrés and A. A. Trabanco, ACS Med. Chem. Lett., 2015, 6, 282 CrossRef PubMed;
(e) C. C. Chrovian, A. Soyode-Johnson, H. Ao, G. M. Bacani, N. I. Carruthers, B. Lord, L. Nguyen, J. C. Rech, Q. Wang, A. Bhattacharya and M. A. Letavic, ACS Chem. Neurosci., 2016, 7, 490 CrossRef PubMed.
-
(a) J. Li, L. J. Kennedy, H. Wang, J. J. Li, S. J. Walker, Z. Hong, S. P. O'Connor, A. Nayeem, D. M. Camac, P. E. Morin, S. Sheriff, M. Wang, T. Harper, R. Golla, R. Seethala, T. Harrity, R. P. Ponticiello, N. N. Morgan, J. R. Taylor, R. Zebo, D. A. Gordon and J. A. Robl, ACS Med. Chem. Lett., 2014, 5, 803 CrossRef PubMed;
(b) A. S. Kalgutkar, H. L. Hatch, F. Kosea, H. T. Nguyen, E. F. Choo, K. F. McClure, T. J. Taylor, K. R. Henne, A. V. Kuperman, M. A. Dombroski and M. A. Letavic, Biopharm. Drug Dispos., 2006, 27, 371 CrossRef PubMed;
(c) C. D. Edlin, G. Morgans, S. Winks, S. Duffy, V. M. Avery, S. Wittlin, D. Waterson, J. Burrows and J. Bryans, ACS Med. Chem. Lett., 2012, 3, 570 CrossRef PubMed;
(d) X.-H. Liu, X.-Y. Xu, C.-X. Tan, J.-Q. Weng, J.-H. Xin and J. Chen, Pest Manage. Sci., 2015, 71, 292 CrossRef PubMed;
(e) M. Siu, R. Pastor, W. Liu, K. Barrett, M. Berry, W. S. Blair, C. Chang, J. Z. Chen, C. Eigenbrot, N. Ghilardi, P. Gibbons, H. He, C. A. Hurley, J. R. Kenny, S. C. Khojasteh, H. Le, L. Lee, J. P. Lyssikatos, S. Magnuson, R. Pulk, V. Tsui, M. Ultsch, Y. Xiao, B.-Y. Zhu and D. Sampath, Bioorg. Med. Chem. Lett., 2013, 23, 5014 CrossRef PubMed.
-
(a) D. Kaase, C. Gotzmann, S. Rein, Y. Lan, S. Kacprzak and J. Klingele, Inorg. Chem., 2014, 53, 5546 CrossRef PubMed;
(b) J. Wu, Q. You, J. Lan, Q. Guo, X. Li, Y. Xue and J. You, Org. Biomol. Chem., 2015, 13, 5372 RSC.
-
(a) G. S. Kumar, C. Kurumurthy, P. S. Rao, B. Veeraswamy, P. S. Rao and B. Narsaiah, J. Heterocycl. Chem., 2015, 52, 75 CrossRef;
(b) A. Reichelt, J. R. Falsey, R. M. Rzasa, O. R. Thiel, M. M. Achmatowicz, R. D. Larsen and D. Zhang, Org. Lett., 2010, 12, 792 CrossRef PubMed;
(c) X.-H. Liu, Z.-H. Sun, M.-Y. Yang, C. Xia Tan, J.-Q. Weng, Y.-G. Zhang and Y. Ma, Chem. Biol. Drug Des., 2014, 84, 342 CrossRef PubMed.
-
(a) T. Swamy, P. Raviteja, G. Srikanth, B. V. S. Reddy and V. Ravinder, Tetrahedron Lett., 2016, 57, 5596 CrossRef;
(b) M. Ciesielski, D. Pufky and M. Döring, Tetrahedron, 2005, 61, 5942 CrossRef.
-
(a) E. Li, Z. Hu, L. Song, W. Yu and J. Chang, Chem.–Eur. J., 2016, 22, 11022 CrossRef PubMed;
(b) L. M. Reddy, V. V. Reddy, P. Sai Prathima, Ch. K. Reddy and B. V. S. S. Reddy, Adv. Synth. Catal., 2018, 360, 3069 CrossRef;
(c) K. S. Vadagaonkar, K. Murugan, A. C. Chaskar and P. M. Bhate, RSC Adv., 2014, 4, 34056 RSC;
(d) K. D. Baucom, S. C. Jones and S. W. Roberts, Org. Lett., 2016, 18, 560 CrossRef PubMed.
-
(a) M. A. Schmidt and X. Qian, Tetrahedron Lett., 2013, 54, 5721 CrossRef;
(b) Z. Ye, M. Ding, Y. Wu, Y. Li, W. Hua and F. Zhang, Green Chem., 2018, 20, 1732 RSC.
-
(a) M. Uyanik and K. Ishihara, ChemCatChem, 2012, 4, 177 CrossRef;
(b) R. D. Richardson and T. Wirth, Angew. Chem., Int. Ed., 2006, 45, 4402 CrossRef PubMed;
(c) S. Minakata, I. Sasaki and T. Ide, Angew. Chem., Int. Ed., 2010, 49, 1309 CrossRef PubMed;
(d) M. Uyanik, H. Okamoto, T. Yasui and K. Ishihara, Science, 2010, 328, 1376 CrossRef PubMed;
(e) D. Liu and A. Lei, Chem.–Asian J., 2015, 10, 806 CrossRef PubMed;
(f) D. Yang, K. Yan, W. Wei, J. Zhao, M. Zhang, X. Sheng, G. Li, S. Lu and H. Wang, J. Org. Chem., 2015, 80, 6083 CrossRef PubMed;
(g) K. Yan, D. Yang, P. Sun, W. Wei, Y. Liu, G. Li, S. Lu and H. Wang, Tetrahedron Lett., 2015, 56, 4792 CrossRef.
-
(a) Y. Yan, Y. Zhang, C. Feng, Z. Zha and Z. Wang, Angew. Chem., Int. Ed., 2012, 51, 8077 CrossRef PubMed;
(b) S. B. Inturi, B. Kalita and A. J. Ahamed, Org. Biomol. Chem., 2016, 14, 11061 RSC;
(c) D. Zhao, T. Wang, Q. Shen and J.-X. Li, Chem. Commun., 2014, 50, 4302 RSC;
(d) Y. Yan, B. Niu, K. Xu, J. Yu, H. Zhi and Y. Liu, Adv. Synth. Catal., 2016, 358, 212 CrossRef.
-
(a) P. Gao, J. Wang, Z. Bai, H. Cheng, J. Xiao, M. Lai, D. Yang and M. Fan, Tetrahedron Lett., 2016, 57, 4616 CrossRef;
(b) P. Gao, J. Wang, Z.-J. Bai, L. Shen, Y.-Y. Yan, D.-S. Yang, M.-J. Fan and Z.-H. Guan, Org. Lett., 2016, 18, 6074 CrossRef PubMed;
(c) P. Gao, J. Wang, Z. Bai, M.-J. Fan, D.-S. Yang and Z.-H. Guan, Org. Lett., 2018, 20, 3627 CrossRef PubMed;
(d) P. Gao, M. Fan, Z. Bai and Y. Wei, Chin. J. Chem., 2015, 33, 479 CrossRef.
-
(a) X. Huang, X. Li, M. Zou, S. Song, C. Tang, Y. Yuan and N. Jiao, J. Am. Chem. Soc., 2014, 136, 14858 CrossRef PubMed;
(b) Q. Gao, S. Liu, X. Wu, J. Zhang and A. Wu, Org. Lett., 2015, 17, 2960 CrossRef PubMed;
(c) W. Zhou, W. Fan, Q. Jiang, Y.-F. Liang and N. Jiao, Org. Lett., 2015, 17, 2542 CrossRef PubMed;
(d) Z. Qi, S. Yu and X. Li, Org. Lett., 2016, 18, 700 CrossRef PubMed;
(e) H. Wang, L.-N. Guo and X.-H. Duan, Chem. Commun., 2014, 50, 7382 RSC;
(f) K. Yan, D. Yang, W. Wei, J. Zhao, Y. Shuai, L. Tian and H. Wang, Org. Biomol. Chem., 2015, 13, 7323 RSC;
(g) K. Yan, D. Yang, W. Wei, F. Wang, Y. Shuai, Q. Li and H. Wang, J. Org. Chem., 2015, 80, 1550 CrossRef PubMed;
(h) N. Zhang, D. Yang, W. Wei, L. Yuan, F. Nie, L. Tian and H. Wang, J. Org. Chem., 2015, 80, 3258 CrossRef PubMed.
-
(a) Y. Tian, C. Sun, R. X. Tan and Z.-Q. Liu, Green Chem., 2018, 20, 588 RSC;
(b) X. Wu, Q. Gao, S. Liu and A. Wu, Org. Lett., 2014, 16, 2888 CrossRef PubMed;
(c) G. Majji, S. Guin, A. Gogoi, S. K. Rout and B. K. Patel, Chem. Commun., 2013, 49, 3031 RSC;
(d) U. Kloeckner, N. M. Weckenmann and B. J. Nachtsheim, Synlett, 2012, 2012, 97 CrossRef.
Footnote |
† Electronic supplementary information (ESI) available: Detailed procedures and NMR data. See DOI: 10.1039/c8ra06215c |
|
This journal is © The Royal Society of Chemistry 2018 |
Click here to see how this site uses Cookies. View our privacy policy here.