DOI:
10.1039/C8RA05936E
(Paper)
RSC Adv., 2018,
8, 36233-36238
Production of cellulase by Trichoderma reesei from pretreated straw and furfural residues
Received
12th July 2018
, Accepted 7th October 2018
First published on 25th October 2018
Abstract
In this study, furfural residues were used as a substrate for cellulase production by the fungi Trichoderma reesei. The results indicated that a low pH and the presence of lignin in the furfural residues have an obvious impact on cellulase production by T. reesei. After pH adjustment, furfural residues could be used for cellulase production by T. reesei, with a higher filter paper activity (FPA) and a higher activity of CMCase compared to that yielded from furfural residues with pH unadjusted. After being washed with 1.6% (w/v) H2O2, all of the lignin in the furfural residues was removed, and an FPA of 7.1 FPU ml−1 and a CMCase activity of 3.4 IU ml−1 were obtained in 115 h, while pretreated straw could yield an FPA of 8.0 FPU ml−1 and a CMCase activity of 2.7 IU ml−1 in 160 h. Moreover, after being treated with H2O2, furfural residues could be used as an inducer in the production of cellulases. With the treated furfural residues added into the medium at the beginning of cultivation, T. reesei gave the maximum FPA (8.4 FPU ml−1) and CMCase activity (4.8 IU ml−1) at 142 h from pretreated straw, which is relatively high for cellulase production compared to that from most other agricultural wastes reported.
1. Introduction
Cellulases hydrolyze β-1,4 glycosidic linkages in cellulose, which are among the most prevalent and stable bonds in nature.1 Cellulase is an enzyme system capable of degrading crystalline cellulose, and consists of several cellobiohydrolases, endoglucanases and β-glucosidases.2 The synergistic action of all three kinds of enzyme component is required for the hydrolysis of cellulose and the entire cellulase system acts to convert cellulose into glucose.3 Cellulases are used in various biotechnological processes, such as in the textile industry, and the food and feed industry.4 Furthermore, this complex enzyme is important for biochemical technology designed to convert lignocellulosic biomass to chemicals and fuels such as ethanol.5–7
Most commercial cellulases are obtained from filamentous fungi such as Trichoderma, Penicillium, and Aspergillus. Among them the ascomycete Trichoderma reesei is the main industrial source of cellulases and hemicellulases, and its high production levels of cellulases are nowadays industrially exploited in the pulp, food and textile industries.3 The industrial strains of T. reesei are today capable of producing more than 100.0 g l−1 of cellulases,8 but it is still necessary to reduce the costs and maximize the efficiency of the produced enzyme mixtures even further and intensive research efforts in this area are ongoing.9
The cost of cellulase is a significant factor in the economics of biomass to ethanol conversion technology. Thus, it has a direct impact on the cost of ethanol production, and therefore needs to be minimized. Reducing the cost of the growth medium and substrate is one of the critical factors for producing highly active cellulase inexpensively.10 Lignocellulosic biomass is an abundant resource that can be used for the production of cellulase. The lignocellulosic material pretreated rice straw was selected as a carbon source for cellulase production in our previous study, while it was recently found that furfural residue is a more economic material for cellulase production. Furfural residue is an industrial waste product which is produced during the manufacture of furfural from corncob, but the environmentally friendly disposal and the utilization of furfural residues are significant challenges to the furfural industries. Furfural residue is a new carbon resource which contains 83.2% glucose, 5.6% arabinose, and 5.9% mannose, and it is said that the process of furfural production could decrease the degree of polymerization of cellulose, thus, the furfural residue is able to be easily hydrolyzed by cellulase.11 Moreover, in the furfural production process, hemicelluloses are hydrolyzed to produce furfural under acid catalysis. Hemicelluloses in biomass have been cited as barriers to cellulase activity,12 but the furfural residues are almost free of hemicelluloses,13,14 and hence, furfural residues are a potential alternative source for cellulase production. In China, about 2.3 million tons of furfural residues are produced every year, but only small amounts are effectively used. As a raw material, the low cost of furfural residues means that they have the potential to reduce the cost of cellulase production.13 Studies on the utilization of furfural residues have shown its potential as a source of bioethanol,15 but there are few reports on the use of furfural residues as a substrate for cellulase production, though enzymatic hydrolysis of furfural residues is an energy saving and environmentally friendly method.29–31 Thus, the aim of this study was to examine the potential utilization of furfural residues as substrates in cellulase production by T. reesei.
2. Materials and methods
2.1. Strains and materials
The cellulase producer used in this study was T. reesei. Furfural residue was collected from Shanxi, China and stored for later use in a freezer. The pretreated straw was kindly supplied by Baimai Green Biological Energy CO., LTD. (Huaian, China). The mineral medium for cellulase production contains 1.6 g l−1 of corn steep liquor, 0.2 g l−1 of CoCl2·6H2O, 1.4 g l−1 of (NH4)2SO4, 2.0 g l−1 of KH2PO4, 0.3 g l−1 of MgSO4 and 0.3 g l−1 of urea and was supplemented with various carbon sources as shown in Table 1.
Table 1 The culture medium and carbon resources used in this study
Medium |
Carbon resource |
PS |
Pretreated straw |
F1 |
Furfural residues (pH adjusted to 5–6) |
F2 |
Furfural residues (pH 4–5, unadjusted) |
F3 |
Furfural residues (treated with 1.0% (w/v) H2O2 and water, pH 5–6) |
F4 |
Furfural residues (treated with 1.6% (w/v) H2O2 and water, pH 5–6) |
PF1 |
Pretreated straw with furfural residue added at 0 h (pH 5–6) |
PF2 |
Pretreated straw with furfural residue added at 72 h (pH 5–6) |
PF3 |
Pretreated straw with furfural residue added at 120 h (pH 5–6) |
2.2. Cellulase production
An inoculum of 1 × 107 spores was added to 3.0 ml of culture medium in a test tube and grown at 30 °C under shaking (200 rpm) for 24 hours. Then all of the preculture was inoculated into 100.0 ml of induction medium in a 500 ml flask. The culture for cellulase production was grown under shaking (200 rpm) at 30 °C. The composition of the cellulase producing medium was the mineral medium supplemented with 30.0 g l−1 of carbon sources. 10.0 g l−1 Tween-80 was added to the culture medium at the beginning of the cultivation. The culture was centrifuged at 10
000g and 4 °C for 10 min and the cell-free culture supernatants were assayed to study the concentration of extracellular proteins and the enzyme production pattern over different phases of growth. All experiments were performed in triplicate.
2.3. Analytical methods
The total concentration of extracellular protein was assayed by the Lowry method,16 and bovine serum albumin was used as the standard protein. The filter paper activities (FPA) of the cellulase preparations were evaluated according to the method described by Ghose et al.17 After incubating at 50 °C for 1 h, 3.0 ml of dinitrosalicylic acid (DNS) was added to the mixture. The released glucose was measured with spectrophotometry, and the activity of the filter paper hydrolysis was evaluated based on the release of glucose, termed FPU that is defined here as the amount of enzyme which catalyzes the formation of 1 μmol of glucose per minute from the hydrolysis of filter paper under assay conditions. CMCase activity is measured by determining the amount of reducing sugars released after enzyme reaction with 20.0 g l−1 of CMC at pH 4.8 and 50 °C for 30 min,18 after which 3.0 ml of DNS was added to the mixture. One unit (IU) of CMCase is defined as the amount of enzyme that liberates 1.0 μmol of glucose per minute under assay conditions. The 3,5-dinitrosalicylic acid (DNS) assay was also used to assay the concentration of produced reducing sugars in the medium, using a glucose standard curve prepared at six different concentrations.
3. Result and discussion
3.1. Effects of pH of furfural residues on cellulase production
It has been reported that the pH of the medium has an obvious influence on cellulase production.20–22 The pH of the furfural residues is about 3–4 and a low pH might be an obstacle to cellulase production by T. reesei from furfural residues. Therefore in this study, the pH of the furfural residues was adjusted to 5–6 (F1) and this was then used for cellulase production by T. reesei. The strains of T. reesei were grown on media F1, PS and F2 respectively, with samples taken at regular intervals, and then the cellulase activity and extracellular proteins of each culture was determined. The results in Fig. 1 show that the maximum FPA and CMCase activity produced by T. reesei on medium PS reached 8.1 FPU ml−1 and 2.3 IU ml−1 after 160 h and 139 h of cultivation respectively, and the maximum FPA and CMCase activity produced by T. reesei on medium F1 reached 5.4 FPU ml−1 and 1.9 IU ml−1 after 115 h and 139 h of cultivation respectively, while T. reesei gave much lower FPA and CMCase activity on medium F2 (Fig. 1a and b). Similar time courses were observed for the extracellular protein production of T. reesei on media PS and F1, while the amount of protein produced by T. reesei on medium F2 is much lower than that on media PS and F1 (Fig. 1c). These results indicated that the low pH of the furfural residue had a negative effect on the secretion of extracellular protein as well as on the cellulase production, and that a pH of 5–6 is suitable for cellulase production by T. reesei from furfural residues. It has been reported that Aspergillus niger and T. viride gave a maximum cellulase production at pH 4.5 and pH 5.5 respectively,23 and also that pH 5–6 is favored by most fungi,17,23 which is consistent with our results.
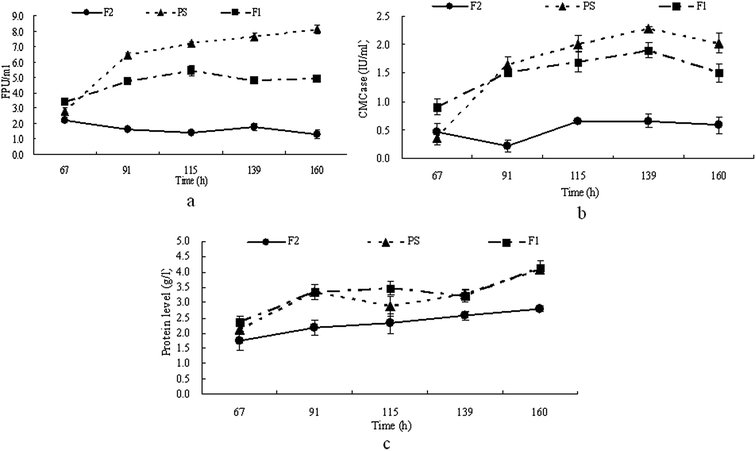 |
| Fig. 1 Time course of FPA (a), CMCase (b) and extracellular protein production (c) on PS, F1 and F2 by T. reesei. | |
3.2. Effects of hemicellulose in furfural residues on cellulase production
Hemicellulose hinders microbial attack, but it was reported that washing with H2O2 could remove most of the hemicellulose thus enhancing microbial utilization.13 Therefore in this study furfural residues were treated with 1.0% (w/v) H2O2 and then washed to neutral with water (used as carbon resource in medium F3) or treated with 1.6% (w/v) H2O2 and then washed to neutral with water (used as carbon resource in medium F4). T. reesei strains were then grown in culture media PS, F3 and F4 respectively. The cellulase activity and extracellular proteins in the medium were determined at regular intervals, and the time courses of the production of extracellular proteins and cellulase activities are shown in Fig. 2. Clearly, as shown in Fig. 2a, the FPA produced by T. reesei on media PS, F3 and F4 were not statistically different during the first 115 h of the culture. The maximum activities (6.4 FPU ml−1 and 7.1 FPU ml−1) of FPA were observed at 115 h in F3 and F4 respectively, while the maximum activity of FPA on medium PS (8.0 FPU ml−1) was not observed until 160 h of cultivation. T. reesei showed the highest activities of CMCase on F4 (3.4 U ml−1) followed by that of on F3 (3.2 U ml−1) at 139 h, while the CMCase in medium PS was much lower and reached its highest activity (2.7 U ml−1) at 160 h (Fig. 2b). In media F3 and F4, the protein level increased during the first 139 hours of cultivation, reached the maximum level at 139 h and then decreased at the end of cultivation. However, in medium PS, the protein level kept increasing until the end of the cultivation (Fig. 1c), corresponding to the production of cellulase in medium PS.
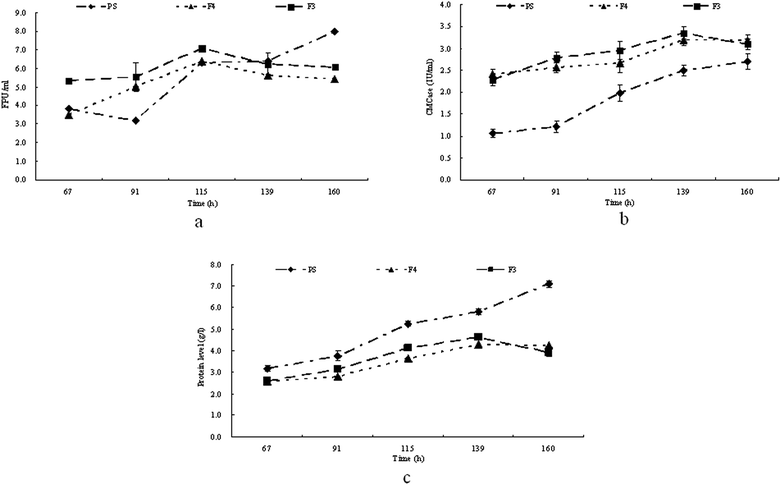 |
| Fig. 2 Time course of FPA (a), CMCase (b) and extracellular protein production (c) on PS, F3 and F4 by T. reesei. | |
In fact, the use of furfural residue for enzyme production has two advantages: the low cost and the ease of its hydrolysis. The furfural residue is made up of a matrix of cellulose and lignin with a small amount of hemicellulose. It was shown in Fig. 2a and b that when T. reesei was grown on media F3 and F4, the strains both gave a lower FPA but a higher CMCase activity within a shortened cultivation time than that of the T. reesei grown on PS. One possible explanation is that the polymerization in the furfural residue is lower than that of in the pretreated straw for the removal of hemicellulose by H2O2. Hemicellulose causes hindrance in microbial attack, thus removing the hemicellulose in the furfural residue with H2O2 is required to enhance microbial utilization. The composition of the furfural residue after being washed with 1.0% H2O2 was 64.1% cellulose and 32.4% lignin, while after being washed with 1.6% H2O2, the composition of the furfural residue was 85.3% cellulose and 11.9% lignin.13 The increase in cellulose was predominantly attributed to the decrease in lignin and makes the furfural residue more accessible to cellulase.13 It has been reported that the culture time of the highest cellulase activity depends upon the substrate,24 and the data in Fig. 2a and b showed that T. reesei produced lower FPA but higher CMCase on media F3 and F4 than on PS, and gave the highest cellulase activities in a shorter culture time, which indicated that the furfural residues were an ideal material for cellulase production by T. reesei.
3.3. Cellulase production from pretreated straw supplemented with furfural residue
The results above showed that with pretreated straw, T. reesei could have a higher activity of FPA, while with furfural residues T. reesei gave a higher activity of CMCase and produced FPA and CMCase activity within a shortened culture time. Therefore T. reesei strains were grown on mineral media containing 30.0 g l−1 of pretreated straw with 10.0 g l−1 of furfural residue added, which were treated with 1.6% H2O2 (furfural residue was added at the 0th hour (PF1), 72nd hour (PF2) and 120th hour (PF3) of the cultivation respectively). The results in Fig. 3a show that when grown on media PF1 and PF2, T. reesei gave the maximum FPA of 8.4 FPU ml−1 and 7.3 FPU ml−1 respectively, which were both observed at 142 h of cultivation. When T. reesei strains were grown on medium PF3, the maximum FPA (7.7 FPU ml−1) was observed at the end of the cultivation (184 h). At the same time, a similar pattern was shown concerning the production of CMCase (Fig. 3b). It is shown in Fig. 3c that the patterns of extracellular protein production were similar in medium PF1, PF2, and PF3. These results indicated that supplementation of the furfural residue in pretreated straw at the beginning of the cultivation could clearly induce the production of cellulase as well as shorten the culture time.
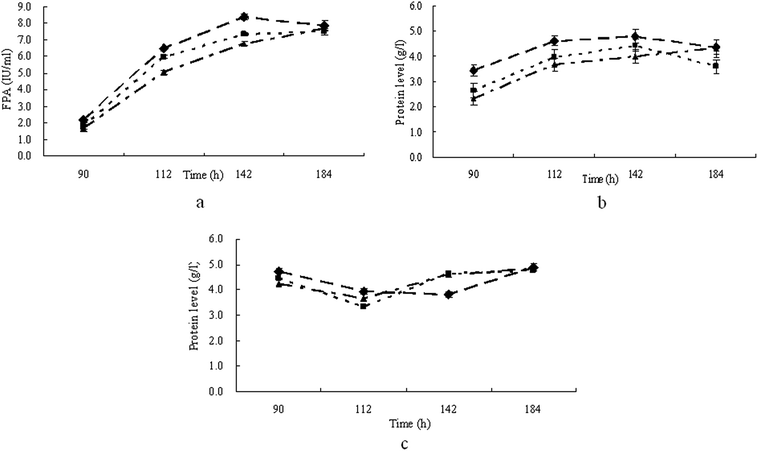 |
| Fig. 3 Time course of FPA (a), CMCase (b) and extracellular protein production (c) by T. reesei when cultivated on medium PF1 (◆), PF2 (■) and PF3 (▲) respectively. | |
The highest cellulase production value (based on filter paper activity) achieved in this study was 8.4 PFU ml−1 and was obtained when T. reesei strains were grown on medium PF1. The cellulase production by T. reesei with different substrates has been compared overall. It has been found that 0.7 FPU ml−1 was produced from steam-treated willow by T. reesei RUT C30 within 120 h,25 while when HAc was added as an inducer to the medium, an enhancement of cellulase production was observed, resulting in a cellulase activity of 1.6 FPU ml−1.26 2.1 FPU ml−1 and 1.3 FPU ml−1 were respectively obtained from Solka Floc and lactose by T. reesei RUT C30 in 120 h. 1.7 FPU ml−1 of cellulase was produced from dairy manure in a culture time of 144 h.19 With acid-swollen cellulose, T. reesei RUT C30 and T. reesei RUT NG14 could both produce 15.0 FPU ml−1 of cellulase.27 For rice straw sticks, pretreatment with alkali led to the highest enzyme activity in the culture medium (1.1 FPU ml−1) within a 144 h culture. It is said that the pretreatment of the rice straw with alkali might cause the removal of lignin in the cellulose, such that the rest of the cellulose components could effectively induce the synthesis of enzymes by the fungal cells.28 For furfural residue, treatment with H2O2 could cause enormous changes of the components with all of the hemicellulose and most of the lignin being removed from the furfural residue.13 This removal of lignin could induce high cellulase activity produced by T. reesei in a shortened culture time. Therefore in this study, supplementation with furfural residue makes cellulase production by T. reesei from low cost pretreated straw more economic.
4. Conclusion
Low pH and the presence of lignin in furfural residues have a negative impact on cellulase production by T. reesei. After pH adjustment or the removal of lignin with H2O2, furfural residues can be used for cellulase production by T. reesei. When grown on a medium containing pretreated straw supplemented with furfural residues, a high activity of cellulase was produced by T. reesei in a shortened culture time. T. reesei gave the maximum FPA (8.4 FPU ml−1) and CMCase activity (4.8 IU ml−1) at 142 h from pretreated straw, which is relatively high for cellulase production compared to from most other agricultural wastes reported.
Conflicts of interest
There are no conflicts to declare.
Acknowledgements
This work was supported by Jiangsu Key Laboratory for Biomass-based Energy and Enzyme Technology (Huaiyin Normal University) and was supported by the National Science Foundation of China (31200023) and National Natural Science Foundation (BS2012SW001) of Shan Dong Province and the J14LE58 and J17KA122, J18KZ008 Project of Shandong Province Higher Educational Science and Technology Program from Shandong Provincial Education Department.
References
- C. P. Kubicek, M. Mikus, A. Schuster, M. Schmoll and B. Seiboth, Metabolic engineering strategies for the improvement of cellulase production by Hypocrea jecorina, Biotechnol. Biofuels, 2009, 2, 19 CrossRef PubMed
. - C. P. Kubicek, The Cellulase Proteins of Trichoderma veesei: structure, multiplicity, mode of action and regulation of formation, Adv. Biochem. Eng./Biotechnol., 1992, 45, 1–22 CrossRef CAS
. - H. Q. Liu, Y. Feng, D. Q. Zhao and J. X. Jiang, Evaluation of cellulases produced from four fungi cultured on furfural residues and microcrystalline cellulose, Biodegradation, 2012, 23, 465–472 CrossRef CAS PubMed
. - D. Tisch, C. P. Kubicek and M. Schmoll, The phosducin-like protein PhLP1 impacts regulation of glycoside hydrolases and light response in Trichoderma reesei, BMC Genomics, 2011, 12, 613 CrossRef CAS PubMed
. - M. G. Adsul, M. S. Singhvi, S. A. Gaikaiwari and D. V. Gokhale, Development of biocatalysts for production of commodity chemicals from lignocellulosic biomass, Bioresour. Technol., 2011, 102, 4304–4312 CrossRef CAS PubMed
. - D. B. Wilson, Cellulases and biofuels, Curr. Opin. Biotechnol., 2009, 20, 295–299 CrossRef CAS PubMed
. - D. B. Wilson, Processive and nonprocessive cellulases for biofuel production-lessons from bacterial genomes and structural analysis, Appl. Microbiol. Biotechnol., 2012, 93, 497–502 CrossRef CAS PubMed
. - J. R. Cherry and A. L. Fidantsef, Directed evolution of industrial enzymes: an update, Curr. Opin. Biotechnol., 2003, 14(4), 438–443 CrossRef CAS PubMed
. - G. Stephanopoulos, Challenges in engineering microbes for biofuels production, Science, 2007, 315(5813), 801–804 CrossRef CAS PubMed
. - G. P. Philippidis, Cellulase Production technology, in Enzymatic Conversion of Biomass for Fuel Production, ed. M. E. Himmel, et al., ACS symposium series, 1994, p. 566 Search PubMed
. - L. Zhang, T. Li, L. Wang and S. Z. Li, Enzymatic hydrolysis of corncob residues of furfural manufacture and optimum conditions for cellulose conversion, Trans. Chin. Soc. Agric. Eng., 2009, 25, 226–230 CAS
. - B. Yang and C. E. Wyman, Effect of xylan and lignin removal by batch and flowthrough pretreatment on the enzymatic digestibility of corn stover cellulose, Biotechnol. Bioeng., 2004, 86(1), 88–98 CrossRef CAS PubMed
. - Y. Xing, L. X. Bu, K. Wang and J. X. Jiang, Pretreatment of furfural residues with alkaline peroxide to improve cellulose hydrolysis characterization of isolated lignin, Cellul. Chem. Technol., 2012, 46, 249–260 CAS
. - S. N. Sun, X. F. Cao, F. Xu, G. L. Jones and M. Baird, Alkaline and organosolv lignins from furfural residue : structural features and antioxidant activity, BioResources, 2014, 9, 772–785 CAS
. - S. Zhang, Study on components of furfural residue from corncob, J. Northeast For. Univ., 1991, 2(1), 98–107 Search PubMed
. - O. H. Lowry, N. J. Rosebrough, A. L. Farr and R. J. Randall, Protein measurement with the folin phenol reagent, J. Biol. Chem., 1951, 193, 265–275 CAS
. - T. K. Ghose, Measurement of cellulase activities, Pure Appl. Chem., 1987, 59, 257–268 CAS
. - M. Mandels, R. Andreotti and C. Roche, Measurement of saccharifying cellulase, Biotechnol. Bioeng. Symp., 1976, 21–33 CAS
. - G. L. Miller, Use of dinitrosalicylic acid reagent for determination of reducing sugar, Anal. Chem., 1959, 31, 426–428 CrossRef CAS
. - Z. Wen, W. Liao and S. Chen, Production of cellulase by Trichoderma reesei from dairy manure, Bioresour. Technol., 2005, 96, 491–499 CrossRef CAS PubMed
. - Y. j. Lee, B. K. Kim, B. H. Lee, K. I. Jo, N. K. Lee, C. H. Chung, Y. C. Lee and J. W. Lee, Purification and characterization of cellulase produced by Bacillus amyoliquefaciens DL-3 utilizing rice hull, Bioresour. Technol., 2008, 99, 378–386 CrossRef CAS PubMed
. - M. Sohail, R. Siddiqi, A. Ahmad and S. A. Khan, Cellulase production from Aspergillus niger MS82: effect of temperature and pH, New Biotechnol., 2009, 25(6), 437–441 CrossRef CAS PubMed
. - A. Das, S. Bhattacharya, K. S. Roopa and S. S. Yashoda, Microbial utilization of agronomic wastes for cellulase production by Aspergillus niger and Trichoderma viride using solid-state fermentation, Dyn. Biochem. Process Biotechnol. Mol. Biol., 2011, 5(2), 18–22 Search PubMed
. - T. V. Ojumu, B. O. Salomon, E. Betiku, S. K. Layokun and B. Amigun, Cellulase Production by Aspergillus flavus Linn isolate NSPR 101 fermented in sawdust, bagasse and corncob, Afr. J. Biotechnol., 2003, 2(6), 150–152 CrossRef CAS
. - K. Reczey, Z. Szengyel, R. Eklund and G. Zacchi, Cellulase production by T. reesei, Bioresour. Technol., 1996, 57, 25–30 CrossRef CAS
. - Z. Szengyel and G. Zacchi, Effect of acetic acid and furfural on cellulase production of Trichoderma reesei RUT C30, Appl. Biochem. Biotechnol., 2000, 89, 31–42 CrossRef CAS PubMed
. - B. S. Montenecourt and D. E. Eveleigh, Selective screening methods for the isolation of high yielding cellulase mutants of Trichoderma reesei, Adv. Chem. Ser., 1979, 181, 289–301 CrossRef
. - W. C. Sun, C. H. Cheng and W. C. Lee, Protein expression and enzymatic activity of cellulases produced by Trichoderma reesei Rut C-30 on rice straw, Process Biochem., 2008, 43, 1083–1087 CrossRef CAS
. - H. Q. Liu, Y. Feng, D. Q. Zhao and J. X. Jiang, Evaluation of cellulases produced from four fungi cultured on furfural residues and microcrystalline cellulose, Biodegradation, 2012, 23(3), 465–472 CrossRef CAS PubMed
. - H. Q. Liu, Y. Feng, J. X. Jiang and L. W. Zhu, Enzymatic Hydrolysis of Furfural Residues Using Crude Trichoderma cellulases, Adv. Mater. Res., 2011, 236–238, 452–455 CAS
. - H. Yu, X. Li, W. Zhang, D. Sun and J. Jiang, Hydrophilic pretreatment of furfural residues to improve enzymatic hydrolysis, Cellulose, 2015, 22(3), 1675–1686 CrossRef CAS
.
|
This journal is © The Royal Society of Chemistry 2018 |
Click here to see how this site uses Cookies. View our privacy policy here.