DOI:
10.1039/C8RA04015J
(Paper)
RSC Adv., 2018,
8, 33383-33390
Cadmium tolerance and accumulation characteristics of wetland emergent plants under hydroponic conditions†
Received
11th May 2018
, Accepted 27th August 2018
First published on 27th September 2018
Abstract
For the purpose of screening a potential Cd-hyperaccumulator for Cd-contaminated soil in paddy fields, four kinds of wetland emergent plants (Iris sibirica L., Acorus calamus L., Typha orientalis Presl and Cyperus alternifolius L.) were investigated for their cadmium tolerance and accumulation characteristics under hydroponic conditions. The physiological responses of plants, Cd concentration in tissues, Cd accumulation, bioaccumulation factor (BCF) and translocation factor (TCF) were investigated to evaluate the abilities of wetland emergent plants to absorb and accumulate Cd. In comparison with the other selected emergent plants, Iris sibirica L. has the strongest Cd-tolerance for the absence of Cd toxic symptoms and a Cd concentration as high as 127.3 mg kg−1 in shoots. Due to its large biomass, the Cd accumulation could reach up to 9.4 mg per plant in roots and 5.7 mg per plant in shoots, respectively. Iris sibirica L. possesses the highest TCF, and its BCF for Cd increased with increasing concentration of spiked Cd in the hydroponic solutions. The results indicate that Iris sibirica L. is a potential Cd-hyperaccumulator that may have a strong capacity for extracting Cd from Cd-contaminated paddy soils.
1. Introduction
Heavy metals that exceed concentrations that the natural environment can bear, can disrupt the balance of the ecosystem and pose a health risk to humans.1 Currently, in the world in general and in China specifically, determining how to control and mitigate heavy metal pollution has become an increasingly prominent issue. Once heavy metals enter into the soil environment, the self-cleaning function of the soil alone, in the short term, cannot effectively eliminate their impact on the ecological environment. Cadmium (Cd) is one of the most hazardous and ubiquitous contaminants in soil and water generated from various sources including industrial and municipal wastes, combustion of fossil fuels and use of agro-chemicals.2,3 Cd, with strong mobility, can easily enter the surrounding environment4,5 and accumulate in plants and animals, and ultimately be enriched in human bodies, resulting in damage to human health.6,7 Therefore, it is important and urgent to develop cost-effective methods to decontaminate Cd from soils.
Phytoremediation of soil was developed in the early 1970s to inhibit excessive accumulation of metals in soils on the basis of the theory that use of green plants can effectively remove contaminants from soil or to lower mobility of contaminants.8,9 Because phytoremediation has the benefits of low cost, ease of operation, beneficial landscape effects, environment-friendliness and sustainability, increasing numbers of countries and enterprises are adopting this technology for soil remediation.10 The key to phytoremediation is the selection of the appropriate heavy metal hyperaccumulators. Some terrestrial plants, such as Thlaspi caerulescens, Sedum alfredii Hance, Elsholtzia splendens Nakai and Commelina communis are known as hyperaccumulators for Cd, showing favorable hypertolerant abilities and extracting rates for the remediation of Cd-contaminated soils.2,11,12 However, the slow growth rate, relatively low biomass and regional type of plants often limited the extensive application of these terrestrial hyperaccumulators.13 Moreover, for the large numbers of Cd-contaminated paddy fields in the south of China, the documented terrestrial Cd-hyperaccumulators are not appropriate choice for in situ phytoremediation. Phytoremediation can absorb heavy metals in water for a long time and concentrate heavy metals in plants. Compared with biochar remediation and microbial remediation, phytoremediation is more economical and energy saving, with less influence factors and easier for centralized treatment.31,32
Previous studies also suggested that a variety of wetland emergent plants were particularly useful in removing organic and inorganic pollutants from polluted waters.14 The wetland emergent plants are known for their well-developed root systems, rapid growth rate and high biomass in a reproductive cycle, thereby have demonstrated favorable capabilities for the removal of heavy metals in wastewater and surface water. For example, Phragmites australis, Typha capensis and Spartina maritime habitated in the estuaries with elevated levels of heavy metals reportedly exhibited significantly higher concentrations of metals in their roots for four elements (Cd, Cu, Pb, and Zn).15 Heavy metal cadmium is concentrated by rice and harmful to human body. Cadmium is the most toxic to plants in three heavy metals such as copper, zinc and cadmium. The results of the study showed that plants have certain selective tolerance to these three heavy metals. The results of the study also showed that plant has more obvious accumulation above three heavy metals, and the accumulation contents in sequence were Zn > Cu > Cd. Echinochloa polystachya, as a potential hyperaccumulator in tropical areas, was found to have the capability of extracting Cd from the Cd-contaminated soil or wetlands.16 Solanum nigrum L. and Rorippa globosa (Turcz.) have been recognized as Cd-hyperaccumulators because the Cd accumulations in Solanum nigrum L. and Rorippa globosa were 103.8–107.0 mg kg−1 in their stems and 124.6–150.1 mg kg−1 in their leaves, respectively.2 Although some wetland emergent plants have shown the capability of uptaking heavy metals, systematic studies regarding the cadmium hyperaccumulation in wetland emergent plants are very lacking.
In order to clarify the tolerance and accumulation characteristics of wetland emergent plants to toxic heavy metals, four kinds of wetland emergent plants, which are extensively used for ecological landscape improvement and constructed wetland engineering, were selected and cultivated with hydroponic solution containing Cd2+. The hydroponic culture rather than soil culture was chosen for the cultivation of plants is to avoid the interference from the soil. Cd uptake by plant and Cd adhesion in soil are closely associated with soil texture and microbial conditions, and the heterogeneity of soil often caused the difficulties in quality control of the experimental soils. In contrast, the hydroponic condition allows a precise control on the culture parameters. The objectives of this study were to evaluate the Cd-tolerance ability of the selected four wetland emergent plant species (i.e., Iris sibirica L., Cyperus alternifolius L., Typha orientalis Presl and Acorus calamus L.), and to assess their capabilities for Cd uptake. We hope that the present study not only gives an insight into the Cd-uptake behaviors of the wetland emergent plants, but also provide useful hyperaccumulators for the phytoremediation of Cd-contaminated soils in paddy fields.
2. Materials and methods
2.1. Wetland emergent plants and hydroponic culture
Iris sibirica L., Acorus calamus L., Typha orientalis Presl and Cyperus alternifolius L., four kinds of common emergent plants, were selected for this study. The plants, six months old and with visually similar biomass, were carefully transplanted from a nursery located in the Daxing district, Beijing to the green house for hydroponic culture in September 2015. The plants were washed thoroughly with running tap water to remove remaining sediment and rinsed with deionized water.15 The aforementioned wetland emergent plants were cultured in polyvinyl-chloride buckets (20.0 cm diameter, 30.0 cm length), and each bucket was placed with three plants and 10 L hydroponic solution. Plants were exposed to five different Cd concentrations (0, 5.0, 10.0, 15.0, 25.0 mg L−1). For each kind of plant, experiments were conducted in triplicate for every Cd concentration. Deionized water was added to maintain constant volume of solution every day, and the nutrient solutions were completely replaced every week. The experiment was carried out in a green house located in the Shunyi district, Beijing, lasting for 60 days before harvesting (from 1st September 2015 to 31st October 2015). The temperature range in the green house recorded for the culture period was from 18.0 to 22.0 °C, and the average photoperiod was ∼11/13 h (light/dark). The nutrient solution for culturing plants included 5.0 mmol KNO3, 5.0 mmol Ca(NO3)2·4H2O, 1.0 mmol KH2PO4, 2.0 mmol MgSO4·7H2O, 9.0 mmol MnCl2·4H2O, 0.8 mmol ZnSO4·7H2O, 0.3 mmol CuSO4·5H2O, 4.0 mmol H3BO3, 0.01 mmol H2MoO4·H2O and 0.8 mmol Fe2(SO4)3 citrate as the iron source.17
2.2. Sample collection and preparation
Plant samples after 60 days of hydroponic culture were collected from the same cylinder, washed with deionized water and 0.01 M HCl, and then dissected into roots (including rhizomes and roots sensu stricto), stems, and leaves in the laboratory. Each respective organ was oven dried at 70.0 °C for 72 h to remove the moisture, ground to fine powder using an analytical mill and homogenized to ensure uniform element distribution. After passing through a 160.0 μm diameter sieve, the powder was weighed and ready for subsequent Cd analysis.18
2.3. Analytical methods
To determine the metal concentrations in plant, 0.2 g powder sample was digested in a Teflon digestion vessel. The digestion solution was composed of 7.0 ml 65 wt% nitric acid (HNO3) and 1.0 ml 30 wt% hydrogen peroxide (H2O2).19 The vessels were then capped and fitted into rotor segments and inserted into the microwave digestion system. The samples were radiated for 35 min. Upon cooling, the vessels were uncapped and solutions were transferred into 100 ml volumetric flasks. The analysis of samples was carried out using an Inductively Coupled Plasma Mass-Spectrophotometer (ICP-MS) instrument (Agilent 7500cx). 2 wt% nitric acid was used as a blank.
2.4. Bioaccumulation factor and translocation factor
The capacities of the four plants to accumulate Cd were evaluated based on the Cd uptake per plant, the bioaccumulation factor (BCF), and the translocation factor (TCF). BCF this study is defined as the ratio of total metal concentration in shoots to that in water, which is an indicator of the ability to uptake and transport metals to the shoots;20,21 TCF is defined as the ratio of heavy metal concentration in the shoot to that in the root.22
2.5. Statistical analysis
All values described in this study are means of three replicates. Analysis of variance (ANOVA) was done by using a statistical software, Statistical Product and Service Solutions (SPSS) version 22.0, to determine the significant difference between the mean values. A least significant difference test (LSD test) was used for the comparison of changes at P < 0.05. Also, hierarchical cluster analysis (HCA) was performed on Cd accumulation and TCF with the Ward's method, which uses the squared Euclidean distance as a similarity measure.
3. Results and discussion
3.1. Physiological responses of wetland emergent plants
Plants may show visible Cd toxic symptoms such as necrosis and whitish-brown chlorosis under Cd stress.12 Therefore, the appearance of plants can be used to assess the Cd-induced damage to the growth of wetland emergent plants. After 60 days of exposure, the appearances of the plants are shown in Fig. 1. Iris sibirica L. did not show symptoms such as necrosis and whitish-brown chlorosis under the stress of all Cd concentrations, which suggested that Iris sibirica L. had a strong tolerance to Cd stress. Cyperus alternifolius L. showed slight chlorosis and little necrosis until the Cd concentration was up to 15.0 mg L−1. Typha orientalis Presl appeared serious whitish-brown chlorosis, showing significant Cd toxicity under all the Cd treatments. Acorus calamus L. showed serious Cd toxic symptoms under all Cd treatments. As a hyperaccumulator, the requisite is that the plant has a high tolerance to heavy metal stresses, which enables them to tolerate and evolve resistance to adverse conditions that are toxic to the majority of plants.23 From the results, it can be deduced that Iris sibirica L. has a very strong tolerance to Cd contamination; such hypertolerance is a pivotal property that makes hyperaccumulation possible.
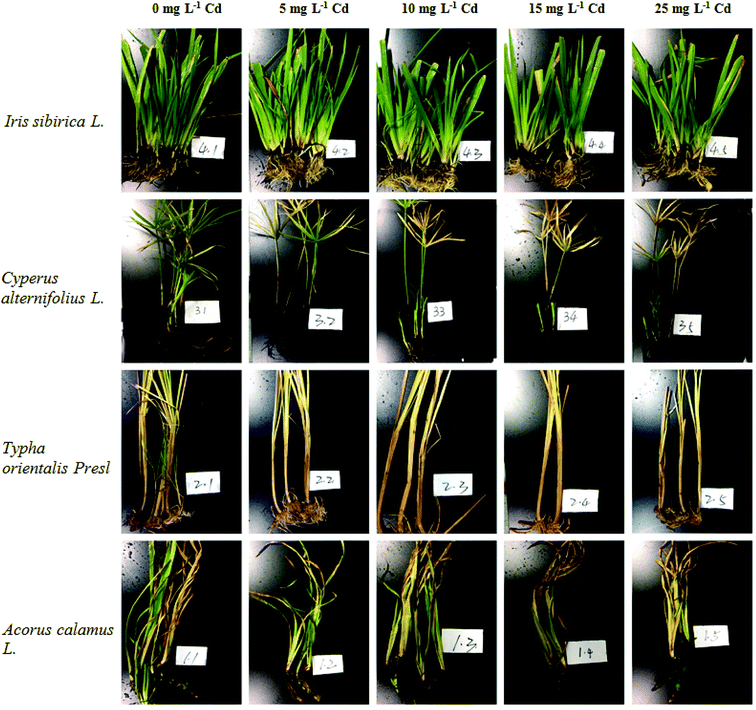 |
| Fig. 1 Growth of wetland emergent plants under the stress of Cd. | |
As for the biomass changes of Iris sibirica L. (Fig. 2), the dry weight increment under Cd treatment was about 20.0 g compared with the control group. Larger biomass could lead to a growth dilution effect on heavy metals and subsequently alleviate the metal phytotoxicity,24 therefore the increased biomass in Iris sibirica L. can further mitigated the toxicity of Cd. Meanwhile, plants with larger biomasses may provide more active absorption sites or metal binding sites.33 The biomass changes of both Cyperus alternifolius L. and Typha orientalis Presl showed same trend: they both reached the largest values at the Cd level of 5.0 mg L−1, and then decreased distinctly. This observation suggested that Cd concentrations higher than 10.0 mg L−1 can inhibit the growth of Cyperus alternifolius L. and Typha orientalis Presl greatly. Relative to the control one, the dry weight change of Acorus calamus L. fell down to negative values when the Cd level exceeded 5.0 mg L−1. It suggested that the treatment of Cd could greatly depress the growth of Acorus calamus L. under Cd stress, and exerted a remarkably deleterious effect on the biomass change of Acorus calamus L. According to the above results, the Cd-tolerance abilities of the emergent plant species tested in this study followed the order: Iris sibirica L. > Cyperus alternifolius L. ≫ Typha orientalis Presl > Acorus calamus L.
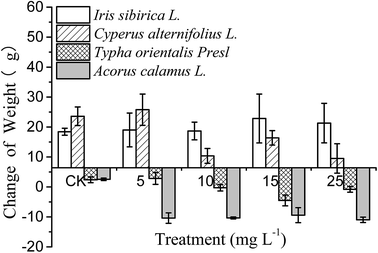 |
| Fig. 2 Changes of total biomass under the stress of Cd. Data are means ± S.D. (n = 3). | |
3.2. Cd concentrations in wetland emergent plant tissues
The amount and distribution of Cd accumulated in the emergent plants under various Cd treatments are depicted in Table S1,† Fig. 3 and 4. The Cd concentrations in all parts of Iris sibirica L. increased with increasing amounts of spiked Cd in the solution (except for the leaf at 15.0 mg L−1 Cd). The highest Cd concentrations in tissues for Iris sibirica L. were found at the 25 mg L−1 Cd treatment: Cd concentrations in root, stem, and leaf were 463.8, 258.4, 56.9 mg kg−1, respectively. The Cd content in the root is much higher than that in the stem or leaf, probably being ascribed to the limited transport of heavy metals from roots to shoots. This phenomenon is in agreement with the earlier reports regarding the absorption of Cd by Baumea juncea, Baumea articulata, Schoenoplectus validus and Juncus subsecundus.17 The distribution of Cd in the tissues of Cyperus alternifolius L. was similar to Iris sibirica L. The Cd concentrations in roots were even higher compared with Iris sibirica L. In the case of Typha orientalis Presl, the Cd concentrations in root, stem and leaf basically showed an ascending trend versus the concentration of spiked Cd and got the peak values at 25.0 mg L−1 Cd. In regard to Acorus calamus L., the concentrations of Cd in root, stem and leaf did not show significant difference when the Cd supply was higher than 10.0 mg L−1, implying that the concentrations of Cd in Acorus calamus L. were almost independent of the exposure levels.
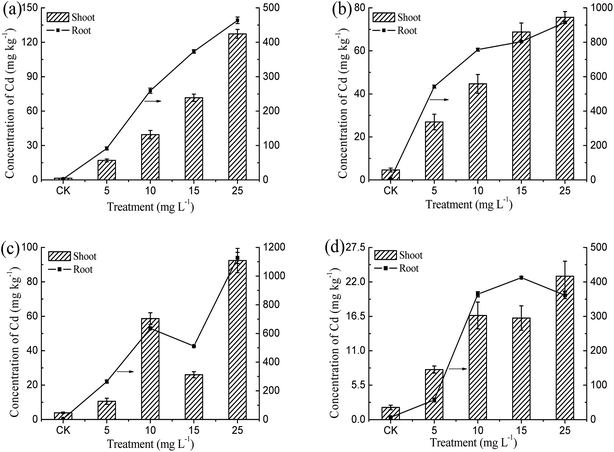 |
| Fig. 3 Concentration of Cd in wetland emergent plants under Cd treatments: (a) Iris sibirica L., (b) Cyperus alternifolius L., (c) Typha orientalis Presl, and (d) Acorus calamus L. Note: shoot Cd concentration is calculated as: (Cstem × Mstem + Cleaf × Mleaf)/(Mstem + Mleaf), where C is the concentration of Cd and M is the biomass of plant. | |
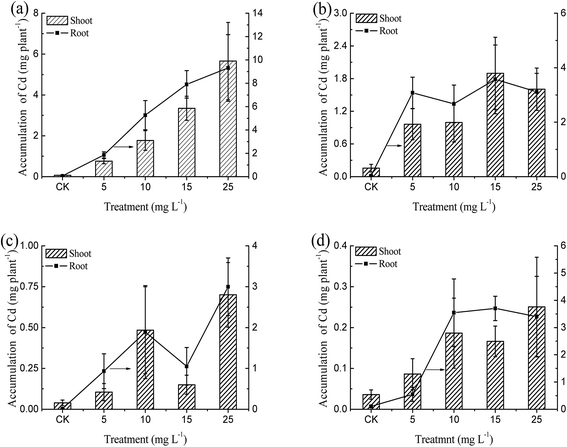 |
| Fig. 4 Accumulation of Cd in wetland emergent plants under Cd treatments: (a) Iris sibirica L., (b) Cyperus alternifolius L., (c) Typha orientalis Presl, and (d) Acorus calamus L. Note: shoot Cd accumulation is (Cstem × Mstem + Cleaf × Mleaf), where C is the concentration of Cd and M is the biomass of plant. | |
The Cd concentrations in the four emergent plants were both in the sequence: root > stem > leaf. The results suggested that plants accumulated a great amount of Cd in their tissues, and a large portion of Cd was stored in the roots and the rest was translocated to the shoots. This is in agreement with previous report that most of Cd accumulated in the roots of sugarcane.25 Similar results were also observed for the uptake of arsenic by winter wheat.26 It appears that Cd(II) metal move predominately into the root apoplast and accumulate near the endodermis. The endodermis acts as a partial barrier to the movement of heavy metals between roots and shoots.25 The barrier effect also explained the nice tolerance of Iris sibirica L. to high concentration of Cd in hydroponic solution.
Generally, the normal level of Cd in most of plants was only 0.1 mg kg−1, and the threshold value that is often used to define a Cd hyperaccumulator is 100.0 mg kg−1 dry weight of shoots.2 A larger amount of heavy metals accumulated in the aboveground parts of a hyperaccumulator was conducive to shifting out metals from environment by harvesting the aboveground parts.27 As shown in Fig. 3, the total Cd concentration in shoots ranged from 1.5 to 127.3 mg kg−1. When the Cd concentration in hydroponic solution went up to 25.0 mg L−1, the Cd concentrations in the shoots of Iris sibirica L., Cyperus alternifolius L., Typha orientalis Presl and Acorus calamus L. reached maxima of 127.3, 75.6, 92.4 and 22.9 mg kg−1, respectively. The concentration of Cd in the shoot of Iris sibirica L. exceeded the critical level of 100.0 mg kg−1, thus Iris sibirica L. can be deemed as a Cd-hyperaccumulator. Meanwhile, Typha orientalis Presl also accumulated a large amount of Cd in the aboveground parts, and the concentration of Cd in shoots could accumulate 92.4 mg kg−1 under 25.0 mg L−1 Cd solution within 60 days. The results indicated that Typha orientalis Presl could be considered as a candidate for remedying Cd-contaminated sites.
The accumulation of Cd in four kinds of emergent plants also maximized under the 25.0 mg L−1 treatment. As shown in Fig. 4, the Cd accumulation in root and shoot of Iris sibirica L. reached 9.4 and 5.6 mg per plant, respectively. Cd accumulation in the root accounted for 62% of the total Cd accumulation. This is similar to the Cd distribution in a terrestrial plant. Tissues of Solanum photeinocarpum extracted 157.0 and 138.0 μg per plant in roots and shoots at 60.0 mg kg−1 Cd in soil: the accumulation in the root took up 53% of the total.28
Although the concentration of Cd in root of Iris sibirica L. was lower than that of Typha orientalis Presl, the overall uptake amount by Iris sibirica L. was far more than other emergent plants due to its large biomass. Correlation analysis showed that the concentrations and the accumulations of Cd in Iris sibirica L. were significantly positively related to the concentrations of Cd in the hydroponic solution. The corresponding regression equations can be expressed as:
|
YCR = 19.311X + 25.676 (R2 = 0.94**)
| (1) |
|
YCS = 5.1651X − 5.4411 (R2 = 0.99**)
| (2) |
|
YAR = 393.26X + 556.34 (R2 = 0.93**)
| (3) |
|
YAS = 230.75X − 225.51 (R2 = 0.99**)
| (4) |
where
YCR and
YCS are Cd concentrations in root and shoot of
Iris sibirica L.;
YAR and
YAS are Cd accumulations in root and shoot of
Iris sibirica L., respectively.
X is the concentration of Cd in hydroponic solution. The significant correlation indicated that the Cd accumulation in
Iris sibirica L. may be further improved under the hydroponic condition higher than 25.0 mg L
−1 Cd.
Hierarchical cluster analysis (HCA) is an unsupervised pattern detection method that partitions all cases into smaller groups or clusters of relatively similar cases that are dissimilar to other groups. The lower the distances between the samples, the more similar they are.29 HCA was performed on data of Cd accumulation, as shown in Fig. 5. The dendrogram shows the similarities and dissimilarities of accumulating ability between emergent plants. The samples of Typha orientalis Presl and Acorus calamus L. formed the first cluster, indicating that the two samples were similar and accumulations of Cd are small. The more similar sample to this cluster was Cyperus alternifolius L., and the more dissimilar sample to the first cluster was Iris sibirica L. Overall, the Cd uptake capabilities of the emergent plants followed the decreasing order: Iris sibirica L. > Typha orientalis Presl > Cyperus alternifolius L. > Acorus calamus L.
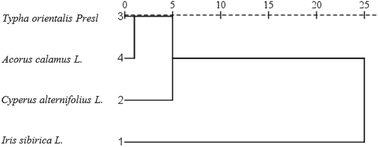 |
| Fig. 5 Hierarchical cluster analysis of the accumulations among emergent plants. | |
3.3. Bioaccumulation factor (BCF) and translocation factor (TCF)
The BCF value is an important index to reflect the capacity of plant to extract heavy metals from the surrounding environments. The BCF can be used to estimate the potential of a plant for phytoremediation,17 In general, plant species exhibiting BCF greater than 1.0 is suitable for the phytoextraction of metals.2 Under the exposure of Cd contamination, most of the BCF values for the four kinds of emergent plants were far more than one or very close to one (Fig. 6a). This observation suggested that the emergent plants used in this experiment all possessed the ability to uptake Cd heavy metal from the hydroponic solution.
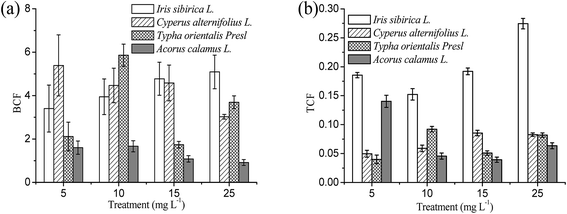 |
| Fig. 6 BCF (a) and TCF (b) for Iris sibirica L., Cyperus alternifolius L., Typha orientalis Presl and Acorus calamus L. under different Cd concentrations (5 mg L−1, 10 mg L−1, 15 mg L−1, 25 mg L−1 Cd). Results are expressed as means ± S.D. (n = 3). | |
In Fig. 6a, it is also observed that the BCF values of Iris sibirica L. are positively correlated with the Cd concentration in hydroponic solution, indicating an increased efficiency of Cd accumulation in upground parts with increasing Cd concentrations in hydroponic solution. This is very different from the reported Cd accumulators. The BCF values of Solanum photeinocarpum, Arthrocnemum macrostachyum and Bidens pilosa were reportedly to decrease with increasing Cd concentrations: the relationship between the BCF values and Cd concentrations was negative and logarithmic linear, indicating a diminishing efficiency of Cd accumulation with increasing Cd concentrations.2,28,30 The increased BCF in this study further confirmed that the Iris sibirica L. has a strong ability to extract Cd from the contaminated matrix. Unlike the Iris sibirica L., the BCF values of Cyperus alternifolius L. decreased with the increasing Cd concentration in hydroponic solution. This is probably due to the lower Cd-tolerance of Cyperus alternifolius L. to increased Cd concentrations. Concerning the BCF values of Typha orientalis Presl, they showed a serious fluctuation with the Cd concentration. A pretty high value in BCFs of Typha orientalis Presl were found at 10.0 mg L−1 Cd, and the BCFs at 5.0, 10.0 mg L−1 are lower than 2. The BCF values of Acorus calamus L. were much lower compared to other emergent plants, and they were not strongly dependent on the hydroponic Cd concentration.
Higher TCF values may suggest that the plant can uptake Cd from soil and store them in the shoots with great efficiency, which indicates that the species have strong ability to transfer Cd from roots to shoots.2 TCFs of all the plant species were all less than one (Fig. 6b), indicating that these plant species were not efficient in translocating Cd metal to shoots. Among all the plants screened, Iris sibirica L. had the highest TCF (0.15–0.27), but did not show any significant changes under all Cd treatments. The higher TCF values among all the emergent plants were Iris sibirica L., this evidenced Iris sibirica L. has stronger ability to translocate the Cd heavy metal from roots to the upground shoots. Susana et al. reported that the TCF values of Arthrocnemum macrostachyum were 0.97 and 1.03 for 0.05 and 0.20 mmol L−1 Cd in hydroponic solution, respectively.30 The TCF values of B. pilosa at the flowering and mature stages were 1.3–7.4 and 1.9–14.4 for Cd extraction from soils, respectively.2 These terrestrial plants showed higher TCF values than Iris sibirica L. However, from a perspective of practical application, the TCF value may not an important criterion for the phytoremediation of wet soil by emergent plants because their roots and shoots can be harvested easily.
4. Conclusion
The present study investigated the cadmium tolerance and accumulation characteristics of four emergent plants. Among all the studied emergent plants, Iris sibirica L. had the greatest tolerance to Cd stresses and the strongest Cd uptake ability under hydroponic culture condition. The concentration of Cd in shoots of Iris sibirica L. was more than 100.0 mg kg−1 under the hydroponic condition of 25.0 mg L−1 Cd, which reached the standard of defining a Cd-hyperaccumulator. Meanwhile, significant correlation was found between Cd accumulation in Iris sibirica L. and the concentrations of Cd in the hydroponic solution, which indicated that the Cd accumulation in Iris sibirica L. may be further improved under the hydroponic condition higher than 25.0 mg L−1 Cd. The BCF of Iris sibirica L. was higher than 1 and increased with increasing Cd concentration in hydroponic solution, which further confirmed the hyperaccumulation characteristics of Iris sibirica L. Although field studies are needed to test the its performance for extracting Cd from real wet soil, the findings of this work have demonstrated the potential of Iris sibirica L. as an engineering Cd-hyperaccumulator.
Conflicts of interest
There are no conflicts to declare.
Acknowledgements
This work was kindly supported by the National Science and Technology Major Project of China (2016ZX05040001), Central Level, Scientific Research Institutes for Basic R&D Special Fund Business (201409030, 2014-GZ-006).
References
- M. Adrees, S. Ali, M. Rizwan, M. Zia-Ur-Rehman, M. Ibrahim, F. Abbas, M. Farid, M. F. Qayyum and M. K. Irshad, Mechanisms of silicon-mediated alleviation of heavy metal toxicity in plants: A review, Ecotoxicol. Environ. Saf., 2015, 119, 186–197 CrossRef CAS PubMed.
- Y. Sun, Q. Zhou, W. Lin and W. Liu, Cadmium tolerance and accumulation characteristics of Bidens pilosa L. as a potential Cd-hyperaccumulator, J. Hazard. Mater., 2009, 161, 808–814 CrossRef CAS PubMed.
- S. C. Wu, K. C. Cheung, Y. M. Luo and M. H. Wong, Effects of inoculation of plant growth-promoting rhizobacteria on metal uptake by Brassica juncea, Environ. Pollut., 2006, 140, 124–135 CrossRef CAS PubMed.
- M. I. Rucandio, M. D. Petit-Domínguez and R. García-Giménez, Biomonitoring of chemical elements in an urban environment using arboreal and bush plant species, Environ. Sci. Pollut. Res., 2011, 18, 51–63 CrossRef CAS PubMed.
- C. Keller, M. Rizwan, J. C. Davidian, O. S. Pokrovsky, N. Bovet, P. Chaurand and J. D. Meunier, Effect of silicon on wheat seedlings (Triticum turgidum L.) grown in hydroponics and exposed to 0 to 30 μM Cu, Planta, 2015, 241, 847–860 CrossRef CAS PubMed.
- S. Ali, A. Chaudhary, M. Rizwan, H. T. Anwar, M. Adrees, M. Farid, M. K. Irshad, T. Hayat and S. A. Anjum, Alleviation of chromium toxicity by glycinebetaine is related to elevated antioxidant enzymes and suppressed chromium uptake and oxidative stress in wheat (Triticum aestivum L.), Environ. Sci. Pollut. Res., 2015, 22, 10669–10678 CrossRef CAS PubMed.
- A. A. Belimov, N. Hontzeas, V. I. Safronova, S. V. Demchinskaya, G. Piluzza, S. Bullitta and B. R. Glick, Cadmium-tolerant plant growth-promoting bacteria associated with the roots of Indian mustard (Brassica juncea L. Czern.), Soil Biol. Biochem., 2005, 37, 241–250 CrossRef CAS.
- Y. Fushiwaki and K. Urano, Adsorption of pesticides and their biodegraded products on clay minerals and soils, J. Health Sci., 2001, 47, 429–432 Search PubMed.
- S. L. Doty, Enhancing phytoremediation through the use of transgenics and endophytes, New Phytol., 2010, 179, 318–333 Search PubMed.
- M. N. V. Prasad, Phytoremediation of Metal-Polluted Ecosystems: Hype for Commercialization, Russ. J. Plant Physiol., 2003, 50, 686–701 CrossRef CAS.
- K. Perronnet, C. Schwartz and J. L. Morel, Distribution of cadmium and zinc in the hyperaccumulator Thlaspi caerulescens grown on multicontaminated soil, Plant Soil, 2003, 249, 19–25 CrossRef CAS.
- X. E. Yang and P. J. Stoffella, Cadmium tolerance and hyperaccumulation in a new Zn-hyperaccumulating plant species (Sedum alfredii Hance), Plant Soil, 2004, 259, 181–189 CrossRef CAS.
- S. D. Ebbs and L. V. Kochian, Toxicity of Zinc and Copper to Brassica Species: Implications for Phytoremediation, J. Environ. Qual., 1997, 26, 776–781 CrossRef CAS.
- J. S. Dunbabin and K. H. Bowmer, Potential use of constructed wetlands for treatment of industrial wastewaters containing metals, Sci. Total Environ., 1992, 111, 151–168 CrossRef CAS.
- D. P. Phillips, H. Lrd and J. B. Adams, Wetland plants as indicators of heavy metal contamination, Mar. Pollut. Bull., 2015, 92, 227–232 CrossRef CAS PubMed.
- M. L. Pompêo, R. Henry and V. Moschini-Carlos, The water level influence on biomass of Echinochloa polystachya (Poaceae) in the Jurumirim Reservoir (São Paulo, Brazil), Braz. J. Biol., 2001, 61, 19 Search PubMed.
- C. Mânzatu, B. Nagy, A. Ceccarini, R. Iannelli, S. Giannarelli and C. Majdik, Laboratory tests for the phytoextraction of heavy metals from polluted harbor sediments using aquatic plants, Mar. Pollut. Bull., 2015, 101, 605–611 CrossRef PubMed.
- P. A. Sainger, R. Dhankhar, M. Sainger, A. Kaushik and R. P. Singh, Assessment of heavy metal tolerance in native plant species from soils contaminated with electroplating effluent, Ecotoxicol. Environ. Saf., 2011, 74, 2284–2291 CrossRef CAS PubMed.
- E. W. I. Hajar, A. Z. B. Sulaiman and A. M. M. Sakinah, Assessment of Heavy Metals Tolerance in Leaves, Stems and Flowers of Stevia Rebaudiana Plant, Procedia Environ. Sci., 2014, 20, 386–393 CrossRef CAS.
- O. Jitar, C. Teodosiu, A. Oros, G. Plavan and M. Nicoara, Bioaccumulation of heavy metals in marine organisms from the Romanian sector of the Black Sea, New Biotechnol., 2015, 32, 369–378 CrossRef CAS PubMed.
- N. Caille, F. J. Zhao and S. P. Mcgrath, Comparison of root absorption, translocation and tolerance of arsenic in the hyperaccumulator Pteris vittata and the nonhyperaccumulator Pteris tremula, New Phytol., 2010, 165, 755–761 CrossRef PubMed.
- Z. Yanqun, L. Yuan, C. Jianjun, C. Haiyan, Q. Li and C. Schvartz, Hyperaccumulation of Pb, Zn and Cd in herbaceous grown on lead-zinc mining area in Yunnan, China, Environ. Int., 2005, 31, 755–762 CrossRef PubMed.
- B. Wang, J. Wang, H. Zhao and H. Zhao, Stress induced plant resistance and enzyme activity varying in cucumber, Colloids Surf., B, 2006, 48, 138–142 CrossRef CAS PubMed.
- S. H. Wei and Q. X. Zhou, Identification of weed species with hyperaccumulative characteristics of heavy metals, Prog. Nat. Sci.: Mater. Int., 2004, 14, 495–503 CrossRef CAS.
- Z. Xin, Y. G. Zhu, Y. B. Zhang, Y. X. Liu, S. C. Liu, J. W. Guo, R. D. Li, S. L. Wu and B. D. Chen, Growth and metal uptake of energy sugarcane (Saccharum spp.) in different metal mine tailings with soil amendments, J. Environ. Sci., 2014, 26, 1080–1089 CrossRef.
- Q. Liu, H. U. Chengxiao, Q. Tan, X. Sun, S. U. Jingjun, Y. Liang, Q. Liu, H. U. Chengxiao, Q. Tan and X. Sun, Effects of As on As uptake, speciation, and nutrient uptake by winter wheat (Triticum aestivum L.) under hydroponic conditions, J. Environ. Sci., 2008, 20, 326–331 CrossRef CAS.
- Y. Sun, Q. Zhou and C. Diao, Effects of cadmium and arsenic on growth and metal accumulation of Cd-hyperaccumulator Solanum nigrum L, Bioresour. Technol., 2008, 99, 1103–1110 CrossRef CAS PubMed.
- X. Zhang, H. Xia, Z. Li, P. Zhuang and B. Gao, Identification of a new potential Cd-hyperaccumulator Solanum photeinocarpum by soil seed bank-metal concentration gradient method, J. Hazard. Mater., 2011, 189, 414–419 CrossRef CAS PubMed.
- F. Zhou, H. Guo, Y. Liu and Y. Jiang, Chemometrics data analysis of marine water quality and source identification in Southern Hong Kong, Mar. Pollut. Bull., 2007, 54, 745–756 CrossRef CAS PubMed.
- S. Redondogómez, E. Mateosnaranjo and L. Andradesmoreno, Accumulation and tolerance characteristics of cadmium in a halophytic Cd-hyperaccumulator, Arthrocnemum macrostachyum, J. Hazard. Mater., 2010, 184, 299–307 CrossRef PubMed.
- X. Li, W. L. Yang, H. He, S. Wu, Q. Zhou, C. Yang, G. Zeng, L. Luo and W. Lou, Responses of microalgae Coelastrella sp. to stress of cupric ions in treatment of anaerobically digested swine wastewater, Bioresour. Technol., 2017, 251, 274 CrossRef PubMed.
- S. Wu, Z. Shen, C. Yang, Y. Zhou, X. Li, G. Zeng, S. Ai and H. He, Effects of C/N ratio and bulking agent on speciation of Zn and Cu and enzymatic activity during pig manure composting, Int. Biodeterior. Biodegrad., 2016, 119, 429–436 CrossRef.
- U. S. Dissanayake, K. U. Tennakoon and N. Priyantha, Potential of two invasive plant species, Lantana camara L. and Wedelia trilobata L., for selective heavy metal uptake, Ceylon J. Sci., Biol. Sci., 2002, 29, 1–11 Search PubMed.
Footnote |
† Electronic supplementary information (ESI) available. See DOI: 10.1039/c8ra04015j |
|
This journal is © The Royal Society of Chemistry 2018 |
Click here to see how this site uses Cookies. View our privacy policy here.