DOI:
10.1039/C8RA03529F
(Paper)
RSC Adv., 2018,
8, 23426-23432
Removal of acenaphthene from water by Triton X-100-facilitated biochar-immobilized Pseudomonas aeruginosa
Received
24th April 2018
, Accepted 21st June 2018
First published on 27th June 2018
Abstract
By adding the nonionic surfactant Triton X-100 and using biochar as an immobilization carrier, a Triton X-100-facilitated biochar-immobilized Pseudomonas aeruginosa (TFBIP) material was prepared using the sorption method and was used to treat acenaphthene in water. The results showed that a low concentration of Triton X-100 simultaneously promoted the sorption capacity of the biochar and the degradation activity of P. aeruginosa, thereby significantly enhancing the removal of acenaphthene from water by the immobilized P. aeruginosa material. Compared with the control without Triton X-100, a low concentration of Triton X-100 significantly increased the acenaphthene removal rate by 20–50%. The optimal conditions for preparing the TFBIP were a loading time of 24 h, the use of a bacterial suspension with a concentration of OD600 = 0.2, and a Triton X-100 concentration of 10 mg L−1. The optimized TFBIP material could efficiently remove acenaphthene from water at temperatures of 10–50 °C, pH values of 4.5–10.5, and NaCl concentrations of up to 0.2 mol L−1. The new TFBIP material can be used for the treatment of wastewater and may also be directly used for the remediation of soils contaminated with organic pollutants.
1 Introduction
Large amounts of industrial wastewater and domestic sewage have caused serious water and soil pollution problems worldwide. How to effectively remove refractory organic pollutants, such as polycyclic aromatic hydrocarbons (PAHs) and polychlorinated biphenyls (PCBs), from wastewater has been a problem of major concern for environmental researchers.1–3 Currently, commonly used treatment methods include physical and chemical adsorption and biodegradation technology. Adsorption/sorption technology yields rapid effects but is not a thorough treatment, while biodegradation of persistent pollutants often requires longer treatment cycles. The use of immobilized microorganism technology to remove organic pollutants is a method that combines the advantages of sorption and degradation.4–6 In immobilized microorganism technology, free microorganisms are immobilized onto a carrier via chemical or physical methods, after which the carrier material allows rapid sorption of organic pollutants, providing a sufficient concentration of substrates to allow the immobilized microorganisms to efficiently remove the pollutants.7,8 The key factors affecting the performance of an immobilized microorganism material include the characteristics of the pollutant-degrading bacteria, the immobilization methods, the loading time, the immobilization carrier and so on. Common immobilization methods include adsorption, embedding, cross-linking and covalent binding method. Among of them, the former two are often used because of the low cost, simple operation, little influence on microbial activity and so on.
An ideal immobilization carrier not only provides the space needed for the attachment and survival of the microorganisms but also plays a role in the sorption of enriched pollutants. Biochar is a stable solid material produced by pyrolysis of biomass under anaerobic or hypoxic conditions and is an efficient and inexpensive sorbent.9 Additionally, the microporous structure and many nutrients on the surface of biochar, such as carbon and nitrogen, can provide favourable conditions for the growth of microorganisms, making biochar a very promising immobilization carrier material. However, current research on biochar has primarily focused on its use in soil improvement10–12 and as a sorbent,13,14 and there have been very few studies on biochar-immobilized microorganisms.15 The development of biochar-immobilized microorganism technology has been limited, primarily because of its insufficient efficiency in processing organic pollutants. How to simultaneously improve the pollutant sorption capacity of the immobilization carrier and the pollutant degradation activity of microorganisms are key problems that need to be solved.
Many studies have shown that a number of surfactants can promote the degradation of organic pollutants by microorganisms by changing the bioavailability of organic pollutants, changing the surface hydrophobicity of microorganisms, or by promoting the activities of key enzymes.3,16–18 In our previous study, we demonstrated that surfactants promoted the sorption of hydrophobic organic pollutants by sorbents such as soil, clay minerals, and biochar.19,20 Therefore, the introduction of a surfactant at suitable concentrations into an immobilized microorganism system is likely to further increase the efficiency of the processing of organic pollutants by immobilized microorganism material.
In this study, using acenaphthene as a representative hydrophobic organic pollutant and using Pseudomonas aeruginosa JXQ as a representative pollutant-degrading bacterium, and by adding the non-ionic surfactant Triton X-100 (octylphenol polyoxyethylene ether) into the biochar-immobilized microorganism system, a surfactant-facilitated biochar-immobilized microorganism material was prepared using the sorption method. The optimal immobilization conditions were determined, and the effects and mechanisms of Triton X-100 on the simultaneous enhancement of sorption and pollutant degradation were investigated to provide a new technical method for rapid and efficient processing of refractory organic pollutants in water.
2 Materials and methods
2.1 Materials and chemicals
Biochar was prepared by carbonization through heating under oxygen-limited conditions.21 Dried rice straw was ground into powders, which was sieved through a 0.1 mm mesh and placed in a quartz beaker. After the powders were tamped, the beaker was capped and placed in a muffle furnace at 500 °C for 4 h. The carbonized product was treated with hydrochloric acid (HCl, 1 mol L−1) for 12 h to remove ash, filtered, washed with ultrapure water until the pH was neutral, and oven-dried. In this way, a straw-derived biochar was obtained and stored in a vacuum dryer until further use. The elemental composition and surface area of the biochar were listed in Table 1.
Table 1 Elemental composition and surface area of the biochar
Sample |
C% |
N% |
H% |
O% |
Ash% |
Surface area/m2 g−1 (N2) |
Biochar |
61.2 |
2.3 |
1.8 |
20.6 |
14.1 |
15.4 |
The strain was isolated from an activated sludge, which was obtained from a pharmaceutical factory in Zhejiang, China. The isolate was 99% similar to Pseudomonas aeruginosa based on its 16S rRNA gene sequence (1147 bp) and was named Pseudomonas aeruginosa JXQ (GenBank accession number: KT329290). This isolate was deposited at China Center for Type Culture Collection, under accession number CCTCC M 2015786. The mineral salt medium (MSM) described by Yu, et al.22 with supplement of 0.35 mg L−1 acenaphthene was used for bacterial isolation, growth and maintenance. For inoculation, the strain was incubated in autoclaved MSM with acenaphthene as the sole carbon source and energy source for a week on THZ-C-1 constant temperature shaker (Taicang Lab-Line Instruments, China) at 30 °C and 150 rpm. After centrifuged at 12
000 × g for 20 min (CF16RXII, Hitachi Koki Co., Ltd., Japan), the cells were washed three times with 50 mmol L−1 phosphate buffer (pH 7.0) and resuspended in MSM to a certain cell density.
The acenaphthene used in this study had a purity greater than 98% and was purchased from Shanghai J&K Chemical Ltd. (Shanghai, China), while the Triton X-100 surfactant (analytical-grade) was purchased from Sinopharm Group Co. Ltd. (China). Other chemicals used in this study were of analytical grade.
2.2 Sorption experiment
To assess sorption kinetics, 0.005 g of biochar was accurately weighed and placed in a 40 mL brown sample tube, to which 40 mL of an acenaphthene solution (3.5 mg L−1) was added. Each group were conducted in triplicate, and a blank control without biochar were included. The samples were placed in a shaker set at 30 °C and 120 rpm and were taken out after 0, 5, 12, 24, or 48 h. Upon filtering the samples with a 0.22 μm hydrophilic polytetrafluoroethylene (PTFE) syringe filter, the acenaphthene concentration in the filtrate was determined by Agilent 1260 high-performance liquid chromatography (USA).
For the batch equilibrium experiment, based on the kinetics experiment, the acenaphthene concentration in the solution was determined after sorption equilibrium was achieved at each of the Triton X-100 concentrations (0, 50, 200, and 300 mg L−1). Taking the equilibrium concentration of acenaphthene as the abscissa and the sorption capacity of acenaphthene by S500 as the ordinate, the equilibrium sorption capacity of biochar was obtained under different conditions according to eqn (1):
|
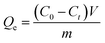 | (1) |
where
C0 is the initial concentration of acenaphthene (mg L
−1);
Ct is the concentration of acenaphthene after equilibrium is achieved (mg L
−1);
V is the volume of solution (L);
m is the mass of biochar (g); and
Qe is the sorption capacity (mg g
−1).
2.3 Degradation experiment
To flasks containing 100 mL of basal mineral salt medium (MSM) with a pH of 7.0 and an acenaphthene concentration of 3.5 mg L−1, seed solution was added such that the initial OD600 was 0.005. These samples were placed in a shaking incubator set at 30 °C and 120 rpm. The concentration of acenaphthene in the solution was determined by sampling at regular intervals, and at the same time, the OD600 value of the culture was also measured. According to the relationship between the bacterial dry weight and OD600, the bacterial dry weight (mg L−1) was then determined.
2.4 Immobilization of P. aeruginosa JXQ by biochar in the presence of Triton X-100
The sorption method was used to immobilize the bacteria to the biochar. P. aeruginosa JXQ was cultured in MSM medium with acenaphthene as the sole carbon source at 30 °C and was centrifuged to separate the solid fraction after culturing for 36 h. The cells were resuspended in MSM, and the resulting bacterial suspension was adjusted to an OD600 of 0.1, 0.2, or 0.5. In these bacterial solutions, Triton X-100 at different concentrations (0–300 mg L−1) was added, and each solution was mixed well. Next, 1% (by the mass ratio) of biochar was added, and the solution was thoroughly mixed at 30 °C to allow the microorganisms to attach to the biochar. After 5–48 h, vacuum filtration was performed to obtain the Triton X-100-facilitated biochar-immobilized bacterial (TFBIP) material. Fig. 1 illustrates the preparation process.
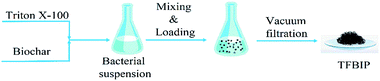 |
| Fig. 1 Preparation of the TFBIP material. | |
2.5 Removal of acenaphthene by biochar-immobilized P. aeruginosa JXQ
To assess the removal of acenaphthene by the biochar-immobilized bacterial materials made using different loading times, different bacterial loading amounts (OD600), or different Triton X-100 concentrations, 0.005 g of each biochar was accurately weighed and placed in a 40 mL brown sample tube, to which 40 mL of acenaphthene solution (3.5 mg L−1) was added. Three parallel biochar types were included in each group, and the samples were placed in a shaker set at 30 °C and 120 rpm. At certain time intervals, samples were removed and filtered with a 0.22 μm hydrophilic PTFE syringe filter. The acenaphthene concentration in the filtrate was then determined by high-performance liquid chromatography.
The oscillator temperature (10–50 °C) and 1 mol L−1 of HCl and NaOH were used to adjust the initial pH value (4.5–10.5) of the acenaphthene solution and to allow different concentrations of NaCl (0.002–1.5 mol L−1) to be assayed. The effects of temperature, pH, and salinity on the treatment of acenaphthene-containing wastewater with the TFBIP material were studied. The removal rate of acenaphthene was calculated according to eqn (2):
|
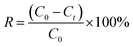 | (2) |
where
C0 is the initial concentration of acenaphthene (mg L
−1);
Ct is the concentration of acenaphthene at time
t (mg L
−1); and
R is the removal rate of acenaphthene (%).
3 Results and discussion
3.1 Utilization of acenaphthene by P. aeruginosa JXQ and the tolerance of Trition X-100
The conclusions: a growth curve of P. aeruginosa JXQ in media containing acenaphthene as the sole carbon source and the degradation of acenaphthene are shown in Fig. 2. Within a few hours after inoculating the medium with a small quantity of bacteria, the concentration of acenaphthene in the medium decreased rapidly, and after 12 h, the bacteria were in log phase growth. After two days of culturing, the concentration of bacteria in the culture increased to OD600 = 0.13 from the initial value of 0.005, while the acenaphthene concentration decreased from an initial concentration of 3.4 mg L−1 to 2.1 mg L−1, a decrease of approximately 38%.
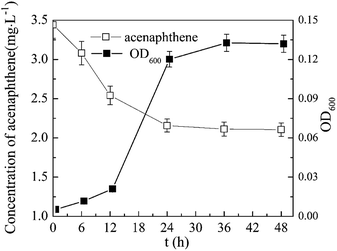 |
| Fig. 2 Degradation of acenaphthene by P. aeruginosa JXQ and its growth curve. | |
P. aeruginosa JXQ could not grow with Triton X-100 as the sole carbon source (data not shown). The tolerance of the strain to Triton X-100 were tested in MSM medium with glucose or acenaphthene as the sole carbon source. In normal MSM with glucose as the carbon source, after 36 h, various concentrations of Triton X-100 (50–500 mg L−1) could all promote the growth of P. aeruginosa JXQ (Fig. 3a). In normal MSM with acenaphthene as the carbon source, the Triton X-100 (50–500 mg L−1) also promoted the growth of the bacteria (Fig. 3b). The result indicates that the bacterium has considerable tolerance to high-concentrations of Triton X-100.
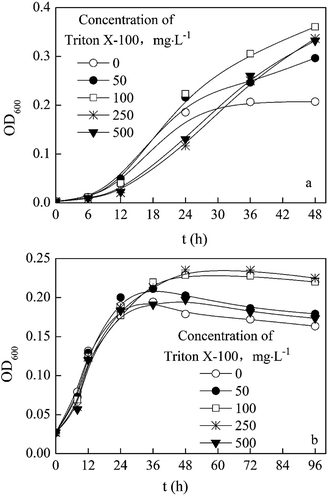 |
| Fig. 3 Effect of Triton X-100 on the utilization of glucose (a) or acenaphthene (b) by P. aeruginosa JXQ. | |
3.2 Optimization of immobilization conditions: bacteria amount and loading time
As described in the methods Section (2.4) and Fig. 1, a biochar-immobilized P. aeruginosa material was prepared by sorption method.
As shown in Fig. 4a, after 48 h treatment, the removal of acenaphthene by biochar without loaded bacteria (control group) was about 63%, whereas the removal of acenaphthene by the immobilized P. aeruginosa JXQ bacterial materials were about 73–77%. The result indicates that the biochar sorption and bacterial degradation both contribute significantly to the removal of acenaphthene. The P. aeruginosa JXQ concentration used during immobilization can affect the actual bacterial loading amount on the immobilized biochar. Using a loading time of 24 h, the biochar-immobilized bacteria have a greater acenaphthene removal efficiency when the concentration of the bacterial suspension has an OD600 = 0.2 than at an OD600 = 0.5 or 0.1. The primary reason for this increased efficiency may be that with an increase in the bacterial concentration, the sorption of bacteria by biochar would gradually approach saturation. When the bacterial density was too high, the limited internal space of the biochar carrier would result in the inhibition of mass transfer for substrates, and the competition of nutrients and dissolved oxygen would cause mutual inhibition among the microorganisms, leading to a decreased processing efficiency of acenaphthene by the immobilized bacterial materials.23 Therefore, under the conditions used in this study, a P. aeruginosa JXQ suspension with an OD600 = 0.2 provided the best immobilization condition.
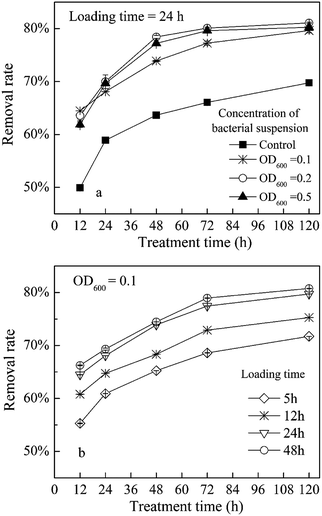 |
| Fig. 4 Acenaphthene removal using biochar-immobilized P. aeruginosa materials prepared with different concentrations of bacterial suspension (a) or loading times (b) (loading time = 24 h). | |
The loading time for preparing the immobilization bacterial materials was also tested. If the loading time is too short, the mechanical strength and stability of the material is inadequate and microorganisms are prone to being unattached to the material; if the loading time is too long, then the mass transfer and microorganism activity may be reduced. Different immobilized materials prepared using different loading times, ranging from 5 to 48 h, were added to different aliquots of the same acenaphthene solution. The acenaphthene removal rates were determined and the results are shown in Fig. 4b. In this study, using a loading time of 24–48 h, the pollutant-degrading bacteria were stably attached to the biochar, and the acenaphthene removal efficiency was highest.
3.3 Improvement of immobilized bacterial material with Triton X-100 and its mechanisms
Using sorption-based immobilization technology, an acenaphthene-degrading bacterial material, biochar-immobilized P. aeruginosa JXQ, was prepared in the presence of different concentrations of Triton X-100. As seen in Fig. 5, for the rapid treatment with a short period of time (5–24 h), low concentrations of Triton X-100 (5–25 mg L−1) significantly enhanced the acenaphthene removal by the immobilized degradation bacteria (P < 0.05). The strongest enhancing effect was observed by using 10 mg L−1 Triton X-100, at which concentration the removal rate increased from 40–65% to 60–80% (P < 0.05). However, at higher concentrations of Triton X-100 (50–300 mg L−1), its enhancing effect on the immobilized bacteria decreased. Specifically, when the Triton X-100 concentration was higher than 100 mg L−1, the acenaphthene removal rate was not significantly different from that without Triton X-100 addition (P > 0.05); when the Triton X-100 concentration was greater than 300 mg L−1, the removal rate was even lower than that without Triton X-100 addition. On the other hand, for long treatment times (72–120 h), Triton X-100 had no significant enhancement of the immobilized degradation bacteria (P > 0.05). Therefore, the addition of 10 mg L−1 Triton X-100 during the immobilization process favoured the most the significant improvement in the rapid removal efficiency of acenaphthene in water by this material.
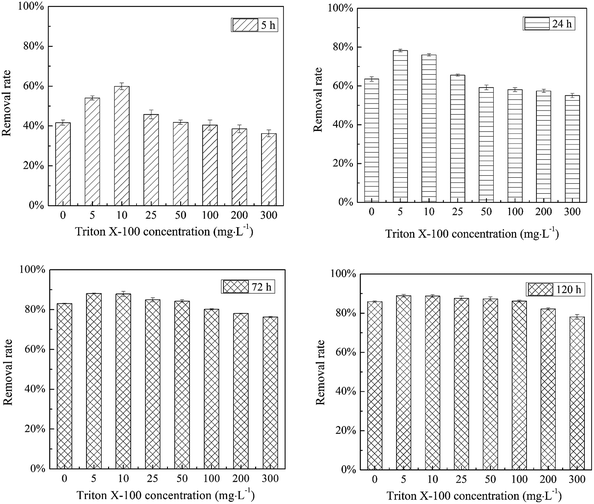 |
| Fig. 5 Removal of acenaphthene by TFBIP materials with different concentrations of Triton X-100. | |
The mechanism by which Triton X-100 enhances the properties of biochar-immobilized microbial materials may occur by two routes. One is the possible effect on the sorption of acenaphthene by the biochar, while the second is its effect on the degradation of acenaphthene by the bacteria immobilized onto the biochar. To further investigate the mechanism of how Triton X-100 enhances the removal of organic pollutants in water by biochar-immobilized bacteria, the effects of Triton X-100 on biochar sorption and on acenaphthene utilization by P. aeruginosa JXQ were studied.
As shown in Fig. 6, when the used Triton X-100 concentration is 0–100 mg L−1, it has a significant enhancing effect on acenaphthene sorption by biochar. As the concentration of Triton X-100 used increases to 200–300 mg L−1, it has a weakened enhancement effect or even displays an inhibitory effect. This is similar to the patterns in previous studies concerning the effect of surfactants on the sorption of organic pollutants in soil.24,25 At low concentrations, surfactants exist as monomers in solution and can form semimicelles on the biochar surfaces, which can effectively attract the hydrophobic organic pollutants in the solution via a solid-phase partitioning effect. Within a limited range, the partition effect increases with the in adsorbed surfactants. When the surfactant concentration in the solution increases to a level above the critical micelle concentration (CMC), the monomers begin to form micelles in the solution. These micelles can compete with the biochar in the sorption of hydrophobic organic pollutants,26 leading to weakened sorption of the organic pollutants onto the biochar and resulting in more organic pollutants being present in solution. This experiment demonstrated that a low-concentration of Triton X-100 could promote the sorption of acenaphthene by biochar and ultimately promote the overall sorption and removal of acenaphthene ability of the biochar-immobilized bacterial material.
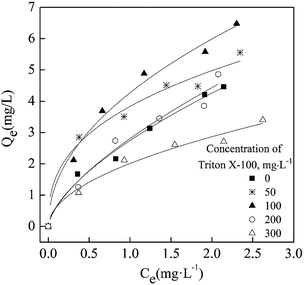 |
| Fig. 6 Equilibrium isotherms for sorption of acenaphthene by S500 biochar in the presence of Triton X-100. | |
Fig. 7 shows the degradation of acenaphthene in MSM medium within 72 h by P. aeruginosa JXQ under different Triton X-100 conditions. The degradation of acenaphthene by P. aeruginosa JXQ at all concentrations of Triton X-100 was stronger than in the absence of Triton X-100, with the strongest degradation of acenaphthene by P. aeruginosa JXQ occurring at a concentration of 200 mg L−1 Triton X-100. The mechanism of how surfactants impact the degradation of organic pollutants by microorganisms could be due to the change of the cell surface hydrophobicity by the surfactant, or alternatively, the promotion of the transmembrane behavior and intracellular degradation of the pollutants.16,27,28 Similar to the results observed in this study, it has been reported that the Tween series of nonionic surfactants can effectively promote the degradation of pyrene by Klebsiella oxytoca at concentrations less than its CMC.18 The result demonstrated that Triton X-100 could also promote the degradation of acenaphthene by P. aeruginosa JXQ at the concentrations less than its CMC.
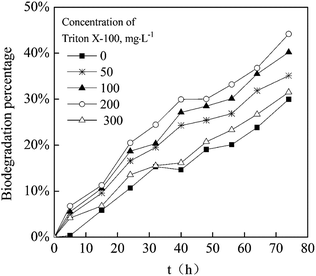 |
| Fig. 7 Degradation of acenaphthene by P. aeruginosa JXQ in the presence of Triton X-100. | |
Based on the separate study of the effects of Triton X-100 on the acenaphthene sorption by biochar and on acenaphthene degradation by the bacteria, it can be speculated that in the Triton X-100-facilitated immobilized system, Triton X-100 can promote both the sorption of acenaphthene by biochar and the degradation of acenaphthene in water by the immobilized bacteria, thereby enhancing the overall ability of the immobilized bacterial material to remove acenaphthene from water.
3.4 Effects of environmental factors on the effectiveness of the application of the TFBIP
In the practical application of immobilized bacterial materials, the complexity of environmental conditions would inevitably affect the removal efficiency of organic pollutants in water. In this study, by using the optimized TFBIP material, the removal of acenaphthene in water was investigated under three common environmental conditions of wastewater. As shown in Fig. 8a and b, between 10 and 50 °C and pH values of 4.5 and 10.5, the water temperature and pH change had no significant effect on acenaphthene removal by the TFBIP material. The optimal growth temperature of P. aeruginosa JXQ in MSM medium containing acenaphthene as the sole carbon source was 37 °C, and the optimal pH was 7.0, with growth observed between pH values of 5.0 and 9.0. The immobilized material exhibited a certain degree of impact resistance to temperature and pH. As shown in Fig. 8c, with an increase in the NaCl concentration, the acenaphthene removal efficiency by the TFBIP material decreased slightly and was significantly inhibited at NaCl concentrations higher than 0.5 mol L−1, primarily because ultra-high salinity completely inhibited the physiological activity of P. aeruginosa JXQ.
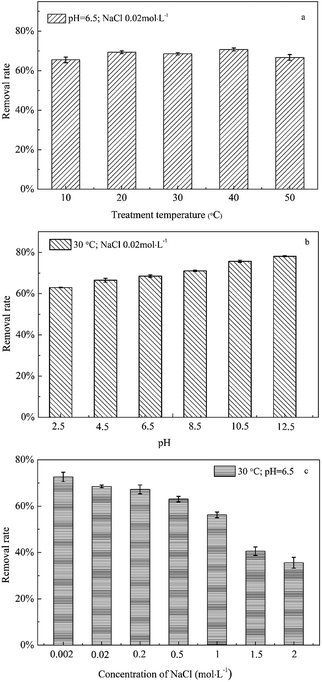 |
| Fig. 8 Effect of different environmental factors on the removal of acenaphthene by TFBIP material. | |
According to the results, the suitable working conditions for the TFBIP material to remove PAHs in water were a temperature of 10–50 °C, a pH of 4.5–10.5, and an NaCl concentration of no greater than 0.2 mol L−1.
4 Conclusions
Triton X-100-facilitated biochar-immobilized P. aeruginosa (TFBIP) material could efficiently remove acenaphthene in water, and the optimal immobilization conditions were a loading time of 24 h, a bacterial solution concentration of OD600 = 0.2, and a Triton X-100 concentration of 10 mg L−1. The addition of Triton X-100 promoted both the sorption capacity of biochar and the degradation activity of P. aeruginosa JXQ, thereby significantly improving the overall efficiency of acenaphthene removal in water by the immobilized material. Low-concentration Triton X-100 could enhance the rapid removal of acenaphthene by the TFBIP. After 24 h of treatment, the removal rate of acenaphthene was 76–78% when Triton X-100 was 5–10 mg L−1, which was 21–24% higher than that without Triton X-100. The suitable application conditions for the TFBIP material to process organic pollutants in water were as follows: waste water temperature of 10–50 °C, pH of 4.5–10.5, and concentration of salt (by NaCl) no greater than 0.2 mol L−1. The TFBIP material can not only be used for the treatment of wastewater, but also be directly used for the remediation of soils contaminated with organic pollutants.
Conflicts of interest
There are no conflicts of interest to declare.
Acknowledgements
This work was supported by the National Natural Science Foundation of China (41301327, 41501521 and 21407037).
Notes and references
- M. S. McLachlan, Environ. Sci. Technol., 1996, 30, 252–259 CrossRef.
- B. C. Kelly, M. G. Ikonomou, J. D. Blair, A. E. Morin and F. A. P. C. Gobas, Science, 2007, 317, 236–239 CrossRef PubMed.
- D. Zhang and L. Z. Zhu, Environ. Pollut., 2012, 164, 169–174 CrossRef PubMed.
- S. X. Wang, X. J. Li, W. Liu, P. J. Li, L. X. Kong, W. J. Ren, H. Y. Wu and Y. Tu, J. Environ. Sci., 2012, 24, 1662–1669 CrossRef.
- H. Park, Environ. Toxicol. Pharmacol., 2012, 34, 345–350 CrossRef PubMed.
- D. V. Tarabukin, M. A. Torlopov, T. N. Shchemelinina, E. M. Anchugova, N. N. Shergina, E. I. Istomina and V. A. Belyy, J. Biotechnol., 2017, 260, 31–37 CrossRef PubMed.
- J. P. Yu, H. J. He, W. L. Yang, C. P. Yang, G. M. Zeng and X. Wu, Bioresour. Technol., 2018, 03, 103, DOI:10.1016/j.biortech.
- C. Y. Zhu, W. L. Yang, H. J. He, C. P. Yang, J. P. Yu, X. Wu, G. M. Zeng, S. Tarre and M. Green, Chemosphere, 2018, 200, 380–387 CrossRef PubMed.
- B. L. Chen and Z. M. Chen, Chemosphere, 2009, 76, 127–133 CrossRef PubMed.
- D. A. Laird, P. Fleming, D. D. Davis, R. Horton, B. Q. Wang and D. L. Karlen, Geoderma, 2010, 158, 443–449 CrossRef.
- X. Peng, L. L. Ye, C. H. Wang, H. Zhou and B. Sun, Soil Tillage Res., 2011, 112, 159–166 CrossRef.
- T. T. Doan, T. Henry-des-Tureaux, C. Rumpel, J. L. Janeau and P. Jouquet, Sci. Total Environ., 2015, 514, 147–154 CrossRef PubMed.
- M. Uchimiya, I. M. Lima, K. T. Klasson, S. Chang, L. H. Wartelle and J. E. Rodgers, J. Agric. Food Chem., 2010, 58, 5538–5544 CrossRef PubMed.
- D. Mohan, A. Sarswat, Y. S. Ok and C. U. Pittman Jr, Bioresour. Technol., 2014, 160, 191–202 CrossRef PubMed.
- M. X. Yuan, Master thesis, Zhejiang University, 2011.
- Z. Y. Zhao, A. Selvam and J. W. C. Wong, Bioresour. Technol., 2011, 102, 3999–4007 CrossRef PubMed.
- H. W. Jin, W. J. Zhou and L. Z. Zhu, J. Environ. Sci., 2013, 25, 1355–1361 CrossRef.
- D. Zhang, L. Z. Zhu and F. Li, Bioresour. Technol., 2013, 142, 454–461 CrossRef PubMed.
- L. Lu and L. Z. Zhu, J. Soils Sediments, 2012, 12, 161–168 CrossRef.
- X. Q. Ji, Master thesis, Zhejiang Gongshang University, 2015.
- Y. Chun, G. Y. Sheng, C. T. Chiou and B. S. Xing, Environ. Sci. Technol., 2004, 38, 4649–4655 CrossRef PubMed.
- H. S. Yu, L. Z. Zhu and W. J. Zhou, J. Hazard. Mater., 2007, 142, 354–361 CrossRef PubMed.
- K. Isaka, S. Yoshie, T. Sumino, Y. Inamori and S. Tsuneda, Biochem. Eng. J., 2007, 37, 49–55 CrossRef.
- W. J. Zhou and L. Z. Zhu, Chemosphere, 2005, 60, 1237–1245 CrossRef PubMed.
- L. Z. Zhu and W. J. Zhou, Environ. Pollut., 2008, 152, 130–137 CrossRef PubMed.
- L. Lu and L. Z. Zhu, Environ. Sci. Pollut. Res., 2012, 19, 1515–1523 CrossRef PubMed.
- J. H. Su, J. H. Xu and Z. L. Wang, Appl. Biochem. Biotechnol., 2010, 160, 1116–1123 CrossRef PubMed.
- Y. Shoji, T. Igarashi, H. Nomura, T. Eitoku and K. Katayama, Anal. Sci., 2012, 28, 339–343 CrossRef PubMed.
|
This journal is © The Royal Society of Chemistry 2018 |