DOI:
10.1039/C8RA03353F
(Paper)
RSC Adv., 2018,
8, 20016-20024
The one-step preparation of green-emission carbon dots based on the deactivator-reducing reagent synergistic effect and the study on their luminescence mechanism†
Received
19th April 2018
, Accepted 25th May 2018
First published on 31st May 2018
Abstract
The preparation of high-quality green-emission carbon dots (g-CDs) has always been the hot spot in the field of nano-materials. This paper reports the synthesis of high-quality g-CDs based on the synergistic effect between the deactivator (polyethyleneimine) and the reducing reagent (citric acid). The emission wavelength and the fluorescence intensity of the prepared CDs are adjustable by controlling the ratio of raw materials and other synthesis parameters, with quantum yields of 22.2% under optimal conditions. The g-CDs can be used as a fluorescent ink without any chemical modification. This paper further proposes the synthesis mechanism as well as the fluorescence mechanism of CDs based on the synergistic effect, laying the foundation of the synthesis and research of CDs.
1. Introduction
Compared with traditional quantum dots and organic dyes, carbon dots (CDs) as a novel type of carbon nanomaterial, exhibit obvious merits, such as low toxicity, excellent biocompatibility, adjustable emission wavelength, easy feasibility to be functionalized, endowing them with a promising outlook in biomedicine, photoelectron, catalysis and sensing.1–7 The raw materials of CDs are abundant and easily available (i.e. glucose,8 citric acid (CA),9 candle soot,10 etc.). Many methods have been reported for the preparation of CDs, but most prepared CDs emit blue fluorescence under ultraviolet excitation.3,11–13 Tan et al. has successfully used polyethyleneimine (PEI) and CA to prepare blue-emission CDs (b-CDs) with quantum yields (QYs) of 48% (λem ≈ 460 nm).12 However, the blue fluorescence of prepared CDs exhibits weak penetrating ability, and it can be easily interfered with by the self-luminescence of biological tissue. g-CDs or red-emission CDs (r-CDs), therefore, show great potential in practical applications to in vivo imaging,14 cancer curing,15,16 and light emitting diodes,2 and their preparation is thus the focus of the research.
There are a few reports on the synthesis of CDs (green to yellow emission), and their preparation is mainly achieved by surface modification17 or heteroatom doping,18,19 especially the selection of better raw materials.20,21 For example, Gu et al. used sugar as a carbon source and DEG as a reaction medium, and successfully prepared green-emission CDs (g-CDs) through a microwave method.21 Although the reaction time (1 min) is very short, the QY of the prepared CDs was only 2.4%. Lin and his team prepared N-doped multi-colored CDs by dissolving N-content aromatic compounds into organic reagent (120–180 °C, 12 h),18,19 and the prepared CDs exhibited strong QYs (g-CDs: 10.4%, b-CDs: 4.8% and r-CDs: 20.6%). However, the reaction time was quite long, and the application of organic reagents was not environment-friendly. The products need a complicated purification process, including high speed centrifugation and chromatographic separation. Hence, the simple and fast preparation of long wavelength-emission CDs is urgently needed in this field.
Ascorbic acid (AA) is an environment-friendly raw material for g-CDs,20,22 and the synthesized product is simple without further purification. Wu et al. has synthesized g-CDs with AA as carbon source, ethanediol and water as solvents (160 °C, 70 min), but the QYs of the products are only 6.79%, which are not appropriate for the application in biological or medical fields.20 Researchers then discovered that though the bare CDs is low in QYs, the proper modification would greatly improve their QYs.8,20,23 Zhang et al. acquired g-CDs with AA as carbon source, and KH791 as deactivator in the mixture of ethanol and water (92 °C, 12 h).8 They discovered that the presence of KH791 could improve QYs from 3.8% to 8.6%.
PEI is a typical water-soluble macromolecular polymer and an ideal material as deactivator in the preparation of CDs.24,25 Guo et al. prepared bare CDs with AA as carbon source in the mixture of ethanol and water (140 °C, 1 h), and then mixed them with PEI (70 °C, 3 h) to acquire PEI-modified CDs. The QYs of modified CDs (5.9%) is four times higher than that of bare ones.25 The improvement in QYs through PEI modification is affirmative, but the effect is limited.
Based on the previous studies, it is discovered that AA could work as the carbon source to prepare g-CDs, and its presence could improve the QYs of prepared CDs, but the improvement is limited. We proposed that the carbon source AA is reductive, prone to be destroyed in the process of preparation, especially with the presence of oxidant or in high temperature and pressure environment, which is unbeneficial to the formation of CDs. The introduction of reducing agent would stabilize AA. Synergistic reaction is great meaningful in the study of nano-materials, which could reduce the reaction time and temperature, improve the conversion ratio, reduce the side reactions and further improve the quality of the products.26 Some research teams have reported the preparation of CDs based on synergistic effect. For example, some researchers discovered that N–S-doped CDs exhibit higher QYs than N-doped or S-doped CDs.27
This paper reports the synthesis of high-quality g-CDs based on the synergistic effect between PEI (deactivator) and CA (reducing agent) with AA as the carbon source. Further studies have been carried out on the impacts of the ratio of reactants, reaction time and temperature upon the prepared CDs. Besides, the mechanisms of synthesis and photoluminescence are also explored through fluorescence spectra and IR spectra and fluorescence lifetime. The study is meaningful for the future studies on the synthesis and the mechanism of the g-CDs. Furthermore, the synthesized g-CDs have durable fluorescence, soluble in water very well and they can be used as a fluorescent ink without any chemical modification.
2. Experimental section
2.1. Chemicals
Polyethylenimine (PEI, Mw = 6 k, 8 k, 10 k, 99%) was purchased from Aladdin (Shanghai, China). Citric acid (CA, ≥99.5%), ascorbic acid (AA, ≥99.7%) and methanol (≥99.5%) were obtained from Sinopharm Chemical Reagent Co., Ltd. All chemicals used were of analytical grade or of the highest purity available. All solutions were prepared using Milli-Q water (Millipore) as the solvent.
2.2. Preparation of C-dots
The g-CDs(AA–PEI–CA) were prepared as detailed in the following: 0.15 g (0.85 mmol) of AA, 0.18 g (0.1 mmol) of PEI and 0.8 g (3.8 mmol) of CA was dissolved in 10 mL of distilled H2O and the mixture was stirred at room temperature for 5 min. Then the solution was transferred to an autoclave for further reaction at 150 °C for 1 h.
For the purification of CDs(AA–PEI–CA), methanol was added dropwise until the reaction mixture became turbid. Then the turbid dispersion was centrifuged (8000 rpm) for 10 min. Subsequently, the isolated CDs(AA–PEI–CA) precipitate was dried under vacuum.
2.3. Characterization
UV-vis absorption spectra were acquired with a Lambda-35 UV-vis spectrophotometer (PerkinElmer Company). Fluorescence spectra were recorded on a LS55 spectrofluorometer (PerkinElmer Company). HRTEM images were obtained with a JEM-2100F transmission electron microscope (Japen Electron Optics Laboratory Company). Fourier transform infrared spectra were obtained on a Nicolet 6700 (IR) spectrometer (Thermo Fisher Scientific), and the sample was tested as solid powder. A FELIX32 system was used to obtain the fluorescence intensity decay curves (Photon Technology International, USA).
2.4. QYs calculations
The QYs of CDs(AA–PEI–CA) and CDs(AA–PEI) were calculated by comparing the integrated fluorescence intensities and absorbance values of the samples (exited at 420 nm), using rhodamine 6G as the standard (QYs = 95% in ethanol).28 The relative QYs can be calculated by using the below equation:
where Φ is the QYs, Grad is the gradient from the plot of integrated fluorescence intensity versus absorbance, and η is the refractive index (1.33 for water and 1.36 for ethanol); ST denotes the standard and x denotes the sample. To minimize reabsorption effects, absorption was always kept below 0.1 at the excitation wavelength.
Unless otherwise indicated, the conditions were as follows: AA: 0.15 g, PEI (Mw = 1800): 0.18 g, CA: 0.8 g, temperature: 150 °C, time: 60 min.
3. Results and discussion
3.1. The synthesis of CDs(AA–PEI)
Without the presence of reducing reagents, the g-CDs(AA–PEI) were synthesized through hydrothermal route with AA as the carbon source and PEI as the deactivator under ideal conditions (AA:0.2 g, PEI (Mw = 1.8 k):0.18 g, pH = 5, 100 °C, 60 min). The QYs of prepared CDs was only 1.43% (λex = 420 nm). The optimization process of reaction parameters was presented in ESI (Fig. S1–S5†). Fig. 1(a) shows the UV-vis absorption spectra and fluorescence spectra of prepared CDs. There exists an obvious absorption peak at 380 nm in the UV-vis spectra of CDs(AA–PEI). When the excitation wavelength moves from 360 nm to 480 nm, the emission peak shifts from 511 nm to 541 nm with obvious decrease in fluorescence intensity.
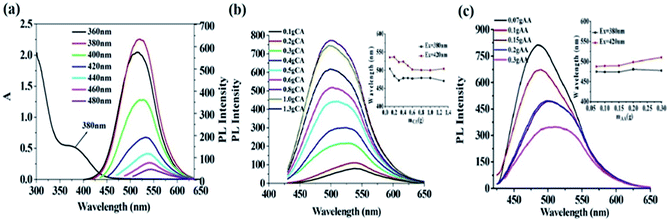 |
| Fig. 1 (a) UV-vis absorption spectra and emission spectra of CDs(AA–PEI) obtained at the excitation wavelength of 360–480 nm with 20 nm increase (slit width: 10–15 nm); (b) impacts of different amount of CA on the fluorescence intensity of CDs(AA–PEI–CA). The inset: the relationship between the amount of CA and emission wavelengths of CDs(AA–PEI–CA) (λex = 380 and 420 nm); (c) impacts of different amount of AA on the fluorescence intensity of CDs(AA–PEI–CA). The inset: the relationship between the amount of AA and emission wavelengths of CDs(AA–PEI–CA) (λex = 380 and 420 nm). | |
Under the same reaction environment, the carbon source AA itself only produced clear solvent without color or fluorescence, showing AA alone could not prepare fluorescent CDs. The experiment results illustrate that the presence of the deactivator PEI plays a very important role in the synthesis of g-CDs. As a net-structured macromolecule rich in amino, PEI could combine with AA, and form g-CDs after the processes of dehydration and the growth of crystal nucleus. Although AA and PEI together could produce g-CDs, the QYs (1.43%) of the products are extremely low, and the adjustability is poor in the emission peak, making it unfit for the application in the fields of biology and medicine.
According to some related researches and our previous work, we believe that the reaction conditions have a significant effect on the properties of the prepared CDs. The carbon dots prepared at low temperature and pressure exhibited low QYs,29 while the hydrothermal route, due to its high reaction temperature and pressure, showed obvious merits like fast reaction rate, less surface defects, better crystallization, and less congregation, which could improve the fluorescence properties of prepared CDs.30 However, the experiments showed that the mere presence of PEI with AA would produce black CDs solvent with weak fluorescence under high temperature (140 °C) or longer reaction time (80 min) (ESI, Fig. S3 and S4†). It is proposed that the active AA exhibits excellent reducibility in the reaction, prone to be oxidized into dehydroascorbic acid and can be further hydrolyzed into diketogulonic acid under acidic condition (ESI, Fig. S6†). The oxidization process would be more violent under high temperature and pressure. This process destroyed the structure and active groups of AA, unbeneficial to the formation of carbon core and surface fluorophores, leading to the extremely low QYs of prepared CDs. To solve this problem, CA was introduced into the system as the reducing reagent to consume the produced oxidation materials during the process, and to enhance the stability of AA under such high temperature and pressure. The experiments show that the presence of CA is beneficial to the formation of high-quality g-CDs.
3.2. The synthesis of high quality g-CDs(AA–PEI–CA)
3.2.1 The impact of CA upon the fluorescence property of CDs. The experiments show that the fluorescence of CDs is obviously improved with the presence of reducing reagent. The impact of CA amount upon the fluorescence properties of prepared CDs(AA–PEI–CA) was investigated with other experiment parameters fixed (AA: 0.15 g, PEI1800: 0.18 g, 150 °C 60 min). As shown in Fig. 1(b), with the increase of the amount of CA, the fluorescence of prepared CDs first increases, and then decreases when the amount reaches 0.8 g. The excitation wavelength is correlated with the emission wavelength (inset of Fig. 1(b)). With the increase of CA, the emission wavelengths all shift to short-wavelength direction, either excited at 380 nm or 420 nm.It can thus be concluded that the presence of CA in the system can effectively protect AA, beneficial to the synthesis of high-quality g-CDs. When the amount of CA is not enough to protect AA, the fluorescence of g-CDs is weak. The abundant amount of CA would ensure effective protection for the stability of AA, and is conducive to the preparation of high-quality g-CDs. However, the excessive amount of CA would react with PEI to form b-CDs (proven by the blue-shift of the emission peak of prepared CDs), causing insufficient amount of PEI for its reaction with AA to form g-CDs. The optimal CA amount used in the system was then set at 0.8 g.
3.2.2 The impact of AA amount upon the fluorescence properties of CDs. With other reaction parameters fixed, the impact of AA amount upon the fluorescence properties of CDs was then examined. As shown in Fig. 1(c), with the increase of AA amount, in the range of 0.07 g–0.3 g, the fluorescence intensity of prepared CDs(AA–PEI–CA) decreases gradually with the emission wavelength shifting to long-wavelength direction. Since the purpose of the experiments is to acquire g-CDs, taking the fluorescence intensity into consideration, we set AA at an optimal amount of 0.15 g. More interestingly, as shown in the inset of Fig. 1(c), when using 380 nm and 420 nm as the excitation wavelengths, the difference of emission wavelengths Δλem (Δλem = λem420 − λem380) gradually enlarges with the increase of AA amount.It can be shown that the g-CDs synthesized by AA and PEI and the b-CDs formed by CA and PEI would superpose. When AA is insufficient, the g-CDs formed by AA and PEI were less than the b-CDs formed by relatively excessive CA and PEI. The overlapping of blue and green luminescence makes the emission wavelength short. With the increased amount AA, the produced g-CDs exceed b-CDs, resulting in the red-shifting of the emission wavelength. However, because the QYs of g-CDs is lower than those of b-CDs, and the excessive amount of AA would decompose without sufficient protection from CA under high temperature and pressure, the fluorescence intensity of prepared CDs gradually decreases. The acquired spectra are actually the mixed spectra of b-CDs prepared by CA and PEI and g-CDs prepared by AA and PEI.
3.2.3 The impact of PEI upon the fluorescence property of CDs. The previous experiments show that PEI is important for the synthesis of g-CDs. According to some researches, there are various deactivators, including polyethyleneglycol (PEG), PEI, ethylenediamine (EDA), and amino acid.25,31–33 With other experiment parameters fixed, PEI and EDA were used as deactivators in the system separately to investigate their effects on the fluorescence properties of the system. As shown in Fig. 2(a), both PEI and EDA could prepare fluorescent CDs, which are obviously better than the bare CDs made purely by AA, showing again the importance of deactivator in the system. However, the fluorescence intensity and the emission wavelength (λem ≈ 512 nm) of CDs prepared with PEI are better than those prepared with EDA (λem ≈ 488 nm).
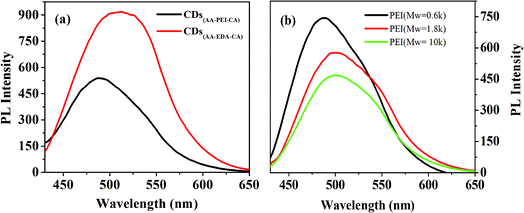 |
| Fig. 2 (a) impacts of different kinds of deactivators on the fluorescence intensity of CDs; (b) impacts of different molecular weight of PEI on the fluorescence intensity of CDs(AA–PEI–CA). | |
The bare CDs would generally contain electron-withdrawing group, which would reduce the fluorescence of prepared CDs.34 The amino groups of the deactivator (PEI or EDA) could combine with the electron-withdrawing groups which are on the surface of the CDs, effectively reducing their amount of electron-withdrawing groups and thus improving the fluorescence intensity of prepared CDs. However, the small molecular weight, simple structure and the low degree of polymerization of EDA make it hard to combine with the carbon source to form fluorescent CDs. In contrast, PEI is a high molecular polymer rich in amino groups, and its high polymerization degree ensures the effective combination with AA to form carbon cores with large particle size, which is propitious to the preparation of long-wavelength g-CDs. Therefore, PEI is an ideal deactivator in the system.
PEI is a high molecular polymer that molecular weight greatly affects its degree of polymerization and viscosity.
The properties of CDs prepared by PEI with different molecular weights might be diverse. With other experiment parameters fixed, the effects of the molecular weight of PEI (Mw = 0.6 k, 1.8 k, 10 k) on the fluorescence properties of prepared CDs(AA–PEI–CA) have been investigated. As shown in Fig. 2(b), the PEI with low molecular weight (Mw = 0.6 k) produces the CDs with strongest fluorescence intensity (QYs = 22.5%) and short wavelength, while the PEI with high molecular weight (Mw = 1.8 k) produces the CDs with weaker fluorescence intensity (QYs = 22.2%) and wavelength red-shifting 15 nm. Further increase in molecular weight (Mw = 10 k) would cause weaker intensity. So, the PEI with molecular weight at 1.8 k was then chosen for the system.
The molecular weight and the degree of polymerization of the deactivator are considered to have effects on the synthesis of CDs. EDA is not beneficial to the form of carbon core because of its low molecular weight, and PEI, due to its high molecular weight, is beneficial to that. However, PEI with the excessive high molecular weight shows excessive high congregation degree and bad water-dispersivity, preventing its sufficient contact with AA. Moreover, PEI with excessive high molecular weight would be serious carbonized in the experiment environment (high temperature and pressure), and the luminescence groups on the surface are easily destroyed, resulting in dark color of prepared CDs and weak fluorescence intensity.
With other experiment parameters fixed, the impact of PEI1800 amount upon the fluorescence properties of prepared CDs(AA–PEI–CA) was examined. The experiment results are presented in ESI(Fig. S7†). The result shows that the amount of PEI1800 does not influence the emission wavelength of prepared CDs(AA–PEI–CA), but the fluorescence intensity obviously. We consider that the little amount of PEI leads to uncompleted or thin modification layer, failing to fill up the surface defects of CDs and thus causing weak fluorescence intensity, while the excessive amount of PEI1800 would make AA disperse among different PEI1800 molecules, which reduce the amount of AA on single PEI molecule. Due to the repulsive forces between like charges among PEI1800 molecules, the dispersed situation of PEI1800–AA combinations is unbeneficial to the form of large-sized carbon cores and consequently the improvement in fluorescence intensity.
3.2.4 The impacts of reaction temperature and time upon the synthesis of CDs. The reaction temperature and time are important factors for the synthesis of CDs. Improper time and temperature would deter the formation of CDs.12 With other experiment parameters fixed, the impact of reaction temperature upon the fluorescence intensity of prepared CDs was then examined. As shown in Fig. 3(a), the fluorescence intensity enhances with the increase of reaction temperature, and reaches the peak at 150 °C (reaction time 60 min). It illustrates that high temperature is beneficial to the fast formation of carbon core through the dehydration and carbonization of the raw material, and the reduction of the surface defects of the prepared CDs, which can thus improve the fluorescence intensity.
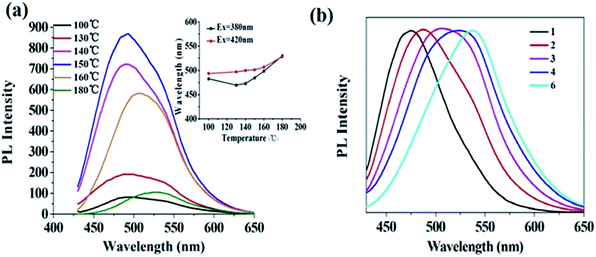 |
| Fig. 3 (a) Impacts of reaction temperature on the fluorescence intensity of CDs(AA–PEI–CA). The inset: the relationship between the reaction temperature and emission wavelengths of CDs(AA–PEI–CA) obtained at the excitation wavelength of 380 and 420 nm; (b) fluorescence spectra of CDs(AA–PEI–CA) under different synthetic conditions (λex = 420 nm). | |
As shown in Fig. 3(a), the reaction temperature has obvious effect on the emission wavelength of prepared CDs. With the increase of temperature, the emission wavelength is red-shifted. Interestingly, the emission wavelengths of prepared CDs excited by 380 nm and 420 nm get close with the increase of reaction temperature, showing that proper temperature (150 °C) is beneficial to the synthesis of high-quality g-CDs. However, when the temperature surpasses 160 °C, the color of the product turns dark with obvious decrease of fluorescence intensity. This may be the result of carbonization and decomposition of raw material before their interaction under high temperature, which prevents the formation of carbon core and destroys the luminescence groups on the surface of CDs and thus results in the sharp decrease of fluorescence intensity.
The impact of reaction time (40–80 min) upon the prepared CDs(AA–PEI–CA) was also examined, and the experiment results are presented in the ESI (Fig. S8†). The result showed that the optimum reaction time is 60 min.
3.2.5 The preparation of CDs(AA–PEI–CA) with controlled emission wavelength. Through the study on the previous experiments, it is discovered that the amount of AA and CA, reaction time and temperature could not only impact the emission wavelength, but also the fluorescence intensity of prepared CDs, while the amount of deactivator PEI exhibits limited impact on the emission wavelength. Therefore, the emission wavelength of CDs can be adjusted by controlling of the synthesis parameters (474–537 nm, blue emission to yellow emission). Table 1 shows the emission wavelength of prepared CDs under different synthesis conditions, and Fig. 3(b) shows the fluorescence spectra of each CDs (the fluorescence intensity of no.5 CDs is too weak, and thus omitted in the figure).
Table 1 Synthesis conditions of CDs with Different Emission Wavelengths
Sample |
λem/nm |
AA/g |
CA/g |
PEI1800/g |
t/min |
T/°C |
1 |
474 |
— |
0.8 |
0.18 |
60 |
150 |
2 |
486 |
0.1 |
0.8 |
0.18 |
60 |
150 |
3 |
504 |
0.15 |
0.8 |
0.18 |
60 |
150 |
4 |
512 |
0.15 |
0.5 |
0.18 |
60 |
150 |
5 |
521 |
0.15 |
— |
0.18 |
60 |
150 |
6 |
537 |
0.2 |
0.2 |
0.18 |
60 |
150 |
3.3. The characterization of CDs(AA–PEI–CA)
3.3.1 Fluorescence spectra. Fig. 4 shows the acquired CDs(AA–PEI) and CDs(AA–PEI–CA) three-dimensional (3D) fluorescence spectra by increasing the excitation wavelength scanned every 10 nm in the range of 300–480 nm. The 3D fluorescence spectra show that though the wavelength of CDs(AA–PEI) and CDs(AA–PEI–CA) both shift with the increase of excitation wavelength, there is a big difference between their spectra. The optimal excitation and emission wavelengths of CDs(AA–PEI) are at 380 nm and 517 nm, while those of CDs(AA–PEI–CA) are at 420 nm and 504 nm. CDs(AA–PEI–CA) maintains strong fluorescence in the wide range of excitation wavelength (475–535 nm), while the effective wavelength for CDs(AA–PEI) is quite narrow (500–535 nm), and the fluorescence intensity sharply drops with the increase of excitation wavelength, which is obviously weaker than that of CDs(AA–PEI–CA).
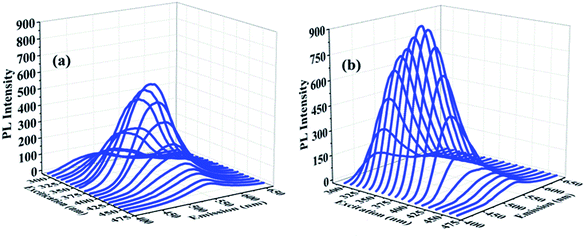 |
| Fig. 4 3D fluorescence spectra of CDs(AA–PEI) (a) (slit width: 10–15) and CDs(AA–PEI–CA) (b) (slit width: 10–10). | |
3.3.2 TEM. TEM has been extensively utilized as a powerful tool to study the morphology of CDs from which the size can be identified. HRTEM images (Fig. 5) clearly reveal that the as-prepared CDs(AA–PEI–CA) are spherical and monodisperse, and the average diameter of CDs was about 3.6 nm.
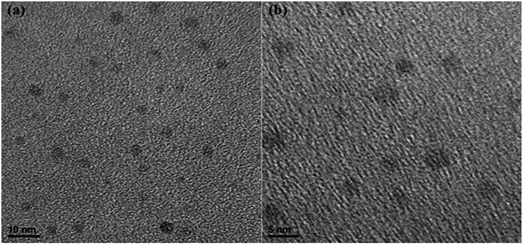 |
| Fig. 5 HRTEM images of CDs(AA–PEI–CA). | |
3.3.3 IR spectra. The IR spectrum was used to analyze the surface structure of prepared CDs. As shown in Fig. S9,† IR spectra of CDs(AA–PEI–CA), CDs(PEI–CA) and CDs(AA–PEI) were measured. The results show that CDs(AA–PEI–CA) have many similar characteristic absorption bands to that of CDs(PEI–CA) (O–H of 3424 cm−1, –CONH– of 1713 cm−1, C–H of 1401 cm−1 and C–O/C–O–C of 1215 cm−1), but significantly different from that of CDs(AA–PEI). Based on the IR spectra, it can be inferred that the source of –CONH– is the interaction between COOH and NH2. The inconspicuous O–H peak in IR spectrum of CDs(AA–PEI) shows the fully reaction of OH from AA with NH2, while the O–H group from CDs may come from OH of CA. The IR spectra prove that CA and PEI interact with AA through synergistic effect.
3.3.4 Fluorescence lifetime. The decay fitting results are listed in Table 2 and the fluorescence decay curves of CDs(AA–PEI–CA) and CDs(AA–PEI) are presented in ESI (Fig. S10†). The results show that CDs(AA–PEI–CA) has three fluorescence lifetimes (τ1, τ2, τ3) and CDs(AA–PEI) has two fluorescence lifetimes (τ1, τ2). The average fluorescence lifetimes of CDs(AA–PEI–CA) and CDs(AA–PEI) are 4.349 ns and 2.914 ns, respectively. Compared with CDs(AA–PEI), CDs(AA–PEI–CA) has a longer fluorescence lifetime and enhanced fluorescence intensity.
Table 2 Fitting parameters of the corresponding fluorescence decay curve
Sample |
λex [nm] |
λem [nm] |
τ1 [ns] |
A1 [%] |
τ2 [ns] |
A2 [%] |
τ3 [ns] |
A3 [%] |
Ave.τ [ns] |
CDs(AA–PEI–CA) |
481 |
535 |
3.115 |
70.75 |
11.23 |
18.89 |
0.226 |
10.36 |
4.349 |
CDs(PEI–CA) |
481 |
535 |
0.3446 |
21.4 |
3.614 |
78.6 |
— |
— |
2.914 |
3.4. Fluorescent ink
The g-CDs can be used as a new type of fluorescent ink due to their excellent dispersibility, solubility and strong fluorescence. The aqueous solution of g-CDs was directly injected into a pen without any chemical modification. Moreover, it was readily flow while writing without any leakage and coagulation within the pen. The handwriting on a filter paper was colorless under ambient light but showed bright green fluorescence under UV-light at a wavelength of 365 nm (Fig. 6). The “vis-invisible” and “UV-visible” properties make it possible for the application of g-CDs in anti-counterfeit fields. Therefore, the g-CDs ink might be an alternative and potential for fluorescent pens.35
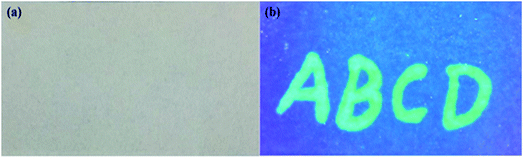 |
| Fig. 6 The fluorescent text is written on a filter paper under ambient light (a) and a UV-light with a wavelength of 365 nm (b) using g-CDs aqueous solution. | |
3.5. The discussion of mechanisms
3.5.1 The mechanism of synthesis. To further understand the synthesis mechanism of CDs(AA–PEI–CA), the process of preparation was studied in detail. AA, CA and PEI were stirred at room temperature for 5 min and then the precursor solution was placed at a suitable temperature (150 °C) for a suitable reaction time (60 min) to obtain a high quality g-CDs (λex = 420 nm, QYs = 22.2%). The CDs exhibit excitation wavelength-dependent fluorescence property and emit strong green fluorescence under the irradiation of UV light, yellow-green fluorescence under purple light (400–425 nm), and bright yellow fluorescence under blue light (465–475 nm).Fig. 7 shows the possible synthesis mechanism of CDs(AA–PEI–CA), where AA works as carbon source, CA as the reducing reagent and PEI as the deactivator. Because of the synergistic effect between the deactivator and the reducing reagent, AA, CA and PEI can form a variety of organic luminescent groups under room temperature. The high temperature (150 °C) of the hydrothermal environment is beneficial to form more organic luminescent groups, and the existence of CA could prevent AA from decomposition, and then the hydroxyl, carboxyl and amino groups could fast aggregate and dehydrate to form carbon core.9 The existence of luminescent groups (amido bond) on the surface of CDs(AA–PEI–CA) as shown in IR spectra (Fig. S9†) verifies the formation of fluorescence groups in the reaction. Furthermore, we hold that the deactivator PEI plays a significant role in the reaction: on the one hand, the branched structure of PEI can work as the backbone to combine with AA or CA, forming compact chain structure, which is beneficial to the process of dehydration and carbonization to form the carbon core; on the other hand, the amino group in PEI is prone to combine with the electron-withdrawing groups on the surface of CDs, reducing the surface defects and thus improving the fluorescence intensity of acquired CDs.36
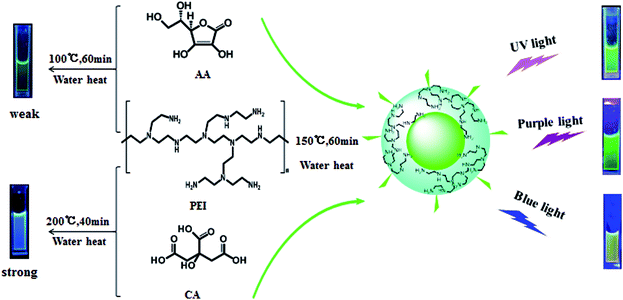 |
| Fig. 7 The possible synthesis mechanism of CDs(AA–PEI–CA). | |
The synergistic effect is also important in the synthesis of CDs(AA–PEI–CA). Under the same reaction condition, the products of AA and CA exhibit no fluorescence, but PEI and CA produce CDs with blue fluorescence, and AA and PEI produce CDs with extreme weak green fluorescence (QYs = 1.43%). However, the presence of these three raw materials could produce g-CDs with high QYs (22.2%), showing the synergistic effect between the deactivator PEI and the reducing reagent CA.
3.5.2 The mechanism of fluorescence. The fluorescence mechanism of CDs has always been a hot spot, but there is still lacking of consensus explanation to it.9,36–39 Yang et al.39 regarded the fluorescence of CDs under low temperature originates from the luminescence groups on the surface (like amide). At high temperature, the carbon core starts forming and the fluorescence of CDs originates from the synergistic effect between the carbon core and the surface luminescent groups, and the carbon core greatly affects the fluorescence of CDs with the increasing reaction temperature.39 Sun et al. consider that the fluorescence come from the radiative recombination of excitons on the surface energy traps of CDs. After the deactivation of the surface, these energy traps become stable, and thus turn to be fluorescence emissivity.36 However, according to the experiments, we propose that the fluorescence of CDs(AA–PEI–CA) comes from two sources: the organic fluorescence groups and the carbon core. Since PEI is the macromolecular substance with branched structure, the hydroxyl (from AA and CA), carboxyl (from CA) and amino (from PEI) interact to form a variety of luminescent groups (mainly amide bond), which results in the weak green fluorescence in precursor solution. And the emission wavelength shifts with the movement of excitation wavelength.The high-quality g-CDs(AA–PEI–CA) (λex = 420 nm, QYs = 22.2%) can be synthesized at a suitable temperature and reaction time. We consider that in the process of synthesis, the hydroxyl, carboxyl and amino would form large conjugated system polymers, which then dehydrate and carbonize under high pressure and temperature, and produce the carbon core capped by luminescent groups. As stated, the fluorescence of this structure originates from carbon core and the surface luminescent groups. Meanwhile, the synergistic effect between hydroxyl and amino group endows the prepared CDs excitation wavelength-dependent property. Under higher temperature (>150 °C) or longer reaction time (>60 min), the over-carbonization would make the product become dark or even black, with weak fluorescence, which shows that the high temperature environment would destroy the luminescent group on the surface of CDs, forming the carbon core without the capping of luminescent groups. Therefore, we believe that different synthesis conditions have a great influence on the structure and luminescence mechanism of prepared CDs.
4. Conclusions
This paper reports the preparation of g-CDs with AA as the carbon source, based on the synergistic effect of the deactivator (PEI) and the reducing reagent (CA). The as-prepared CDs exhibit strong luminescence and the QYs reach 22.2%. Moreover, the g-CDs can be applied as a new type of fluorescent ink. Importantly, through the comparison of CDs prepared by different raw material combinations, we propose the synthesis mechanism based on the synergistic effect between the deactivator and the reducing reagent. The results reveal that different conditions have great effects on the structure and luminescence mechanism of prepared CDs. The reported synthesis method is an ideal way for g-CDs for its simple operation, low temperature and short reaction, and the proposed mechanism offers inspiration for the future synthesis of CDs as well as the studies on them.
Conflicts of interest
There are no conflicts to declare.
Acknowledgements
This research was supported by Natural Science Foundation of Hubei Province (2016CFB615), and the Fundamental Research Funds for the “Central Universities”, South Central University for Nationalities (CZY15020).
Notes and references
- Y. Song, S. Zhu and B. Yang, Bioimaging based on fluorescent carbon dots, RSC Adv., 2014, 4, 27184–27200 RSC.
- X. Y. Zhang, Y. Zhang, Y. Wang, S. Kalytchuk, S. V. Kershaw and Y. H. Wang, et al., Color-switchable electroluminescence of carbon dot light-emitting diodes, ACS Nano, 2013, 7, 11234–11241 CrossRef PubMed.
- R. H. Liu, H. Huang, H. T. Li, Y. Liu, J. Zhong and Y. Y. Li, et al., Metal nanoparticle/carbon quantum dot composite as a photocatalyst for high-efficiency cyclohexane oxidation, ACS Catal., 2013, 4, 328–336 CrossRef.
- F. Y. Yan, Y. Zou, M. Wang, X. L. Mu, N. Yang and L. Chen, Highly photoluminescent carbon dots-based fluorescent chemosensors for sensitive and selective detection of mercury ions and application of imaging in living cells, Sens. Actuators, B, 2014, 192, 488–495 CrossRef.
- Y. Zhang, L. Ge, M. Li, M. Yan, S. G. Ge and J. H. Yu, et al., Flexible paper-based ZnO nanorod light-emitting diodes induced multiplexed photoelectrochemical immunoassay, Chem. Commun., 2014, 50, 1417–1419 RSC.
- S. W. Hu, S. Qiao, B. Y. Xu, X. Peng, J. J. Xu and H. Y. Chen, Dual-Functional Carbon Dots Pattern on Paper Chips for Fe3+ and Ferritin Analysis in Whole Blood, Anal. Chem., 2017, 89, 2131–2137 CrossRef PubMed.
- W. Guo, F. W. Pi, H. X. Zhang, J. D. Sun, Y. Z. Zhang and X. L. Sun, A novel molecularly imprinted electrochemical sensor modified with carbon dots, chitosan, gold nanoparticles for the determination of patulin, Biosens. Bioelectron., 2017, 98, 299–304 CrossRef PubMed.
- Z. C. Yang, M. Wang, A. M. Yong, S. Y. Wong, X. H. Zhang and H. Tan, et al., Intrinsically fluorescent carbon dots with tunable emission derived from hydrothermal treatment of glucose in the presence of monopotassium phosphate, Chem. Commun., 2011, 47, 11615–11617 RSC.
- F. Gao, S. Y. Ma, J. Li, K. Dai, X. C. Xiao and D. Zhao, et al., Rational design of high quality citric acid-derived carbon dots by selecting efficient chemical structure motifs, Carbon, 2017, 112, 131–141 CrossRef.
- X. H. Wang, K. G. Qu, B. L. Xu, J. S. Ren and X. G. Qu, Multicolor luminescent carbon nanoparticles: Synthesis, supramolecular assembly with porphyrin, intrinsic peroxidase-like catalytic activity and applications, Nano Res., 2011, 4, 908–920 CrossRef.
- H. Y. Liu, Z. M. He, L. P. Jiang and J. J. Zhu, Microwave-assisted synthesis of wavelength-tunable photoluminescent carbon nanodots and their potential applications, ACS Appl. Mater. Interfaces, 2015, 7, 4913–4920 Search PubMed.
- J. Y. Li, Y. Liu, Q. W. Shu, J. M. Jiang, F. Zhang and X. P. Chen, et al., One-pot hydrothermal synthesis of carbon dots with efficient up-and down-converted photoluminescence for the sensitive detection of morin in a dual-readout assay, Langmuir, 2017, 33, 1043–1050 CrossRef PubMed.
- C. X. Wang, Z. Z. Xu and C. Zhang, Polyethyleneimine-functionalized fluorescent carbon dots: water stability, pH sensing, and cellular imaging, ChemNanoMat, 2015, 1, 122–127 CrossRef.
- J. C. Ge, Q. Y. Jia, W. M. Liu, L. Guo, Q. Y. Liu, M. H. Lan, H. Y. Zhang and X. M. Meng, Red-emissive carbon dots for fluorescent, photoacoustic, and thermal theranostics in living mice, Adv. Mater., 2015, 27, 4169–4177 CrossRef PubMed.
- J. C. Ge, Q. Y. Jia, W. M. Liu, M. H. Lan, B. Z. Zhou and L. Guo, et al., Carbon dots with intrinsic theranostic properties for bioimaging, red–light-triggered photodynamic/photothermal simultaneous therapy in vitro and in vivo, Adv. Healthcare Mater., 2016, 5, 665–675 CrossRef PubMed.
- C. J. Liu, P. Zhang, X. Y. Zhai, F. Tian, W. C. Li and J. H. Yang, et al., Nano-carrier for gene delivery and bioimaging based on carbon dots with PEI-passivation enhanced fluorescence, Biomaterials, 2012, 33, 3604–3613 CrossRef PubMed.
- X. J. Mao, H. Z. Zheng, Y. J. Long, J. Du, J. Y. Hao and L. L. Wang, et al., Study on the fluorescence characteristics of carbon dots, Spectrochim. Acta, Part A, 2010, 75, 553–557 CrossRef PubMed.
- K. Jiang, S. Sun, L. Zhang, Y. H. Wang, C. Z. Cai and H. W. Liu, Bright-yellow-emissive N-doped carbon dots: preparation, cellular imaging, and bifunctional sensing, ACS Appl. Mater. Interfaces, 2015, 7, 23231–23238 Search PubMed.
- K. Jiang, S. Sun, L. Zhang, Y. Lu, A. G. Wu and C. Z. Cai, et al., Red, green, and blue luminescence by carbon dots: full-color emission tuning and multicolor cellular imaging, Angew. Chem., Int. Ed., 2015, 54, 5360–5363 CrossRef PubMed.
- H. Y. Wu, C. C. Mi, H. Q. Huang, B. F. Han, J. Li and S. K. Xu, Solvothermal synthesis of green-fluorescent carbon nanoparticles and their application, J. Lumin., 2012, 132, 1603–1607 CrossRef.
- Y. Liu, N. Xiao, N. Q. Gong, H. Wang, X. Shi and W. Gu, et al., One-step microwave-assisted polyol synthesis of green luminescent carbon dots as optical nanoprobes, Carbon, 2014, 68, 258–264 CrossRef.
- L. M. Hu, Y. Sun, S. L. Li, X. L. Wang, K. L. Hu and L. R. Wang, et al., Multifunctional carbon dots with high quantum yield for imaging and gene delivery, Carbon, 2014, 67, 508–513 CrossRef.
- C. J. Liu, P. Zhang, F. Tian, W. C. Li, F. Li and W. G. Liu, One-step synthesis of surface passivated carbon nanodots by microwave kassisted pyrolysis for enhanced multicolor photoluminescence and bioimaging, J. Mater. Chem., 2011, 21, 13163–13167 RSC.
- L. M. Shen, L. P. Zhang, M. L. Chen, X. W. Chen and J. H. Wang, The production of pH-sensitive photoluminescent carbon nanoparticles by the carbonization of polyethylenimine and their use for bioimaging, Carbon, 2013, 55, 343–349 CrossRef.
- A. J. Hao, X. J. Guo, Q. Wu, Y. Sun, C. R. Cong and W. J. Liu, Exploring the interactions between polyethyleneimine modified fluorescent carbon dots and bovine serum albumin by spectroscopic methods, J. Lumin., 2016, 170, 90–96 CrossRef.
- K. C. Hsu and D. H. Chen, Green synthesis and synergistic catalytic effect of Ag/reduced graphene oxide nanocomposite, Nanoscale Res. Lett., 2014, 9, 484 CrossRef PubMed.
- Y. Q. Dong, H. C. Pang, H. B. Yang, C. X. Guo, J. W. Shao and Y. W. Chi, et al., Carbon-based dots co-doped with nitrogen and sulfur for high quantum yield and excitation-independent emission, Angew. Chem., Int. Ed., 2013, 52, 7800–7804 CrossRef PubMed.
- R. X. Wang, X. F. Wang and Y. M. Sun, Aminophenol-based carbon dots with dual wavelength fluorescence emission for determination of heparin, Microchim. Acta, 2017, 184, 187–193 CrossRef.
- X. F. Jia, J. Li and E. K. Wang, One-pot green synthesis of optically pH-sensitive carbon dots with upconversion luminescence, Nanoscale, 2012, 4, 5572–5575 RSC.
- P. F. Xu, R. B. Yu, C. R. Wang, X. C. Yan, D. Wang and J. X. Deng, et al., Synthesis of CePO4 nano-wires with improved photoluminescent properties by co-crystallizing with nano-sized CeO2, J. Nanosci. Nanotechnol., 2013, 13, 1498–1502 CrossRef PubMed.
- A. Sachdev, I. Matai, S. U. Kumar, B. Bhushan, P. Dubey and P. Gopinath, A novel one-step synthesis of PEG passivated multicolour fluorescent carbon dots for potential biolabeling application, RSC Adv., 2013, 3, 16958–16961 RSC.
- A. Cayuela, M. L. Soriano and M. Valcárcel, Reusable sensor based on functionalized carbon dots for the detection of silver nanoparticles in cosmetics via inner filter effect, Anal. Chim. Act., 2015, 872, 70–76 CrossRef PubMed.
- W. H. Liu, H. S. Choi, J. P. Zimmer, E. Tanaka, J. V. Frangioni and M. Bawendi, Compact cysteine-coated CdSe (ZnCdS) QDs for in vivo applications, J. Am. Chem. Soc., 2007, 129, 14530–14531 CrossRef PubMed.
- L. Tian, Y. Song, X. J. Chang and S. W. Chen, Hydrothermally enhanced photoluminescence of carbon nanoparticles, Scr. Mater., 2010, 62, 883–886 CrossRef.
- L. Zhou, F. M. Wu, J. H. Yu, Q. H. Deng, F. A. Zhang and G. Wang, Titanium carbide (Ti3C2Tx) MXene: A novel precursor to amphiphilic carbide-derived graphene quantum dots for fluorescent ink, light-emitting composite and bioimaging, Carbon, 2017, 118, 50–57 CrossRef.
- Y. P. Sun, B. Zhou, Y. Lin, W. Wang, K. A. S. Fernando and P. Pathak, et al., Quantum-sized carbon dots for bright and colorful photoluminescence, J. Am. Chem. Soc., 2006, 128, 7756–7757 CrossRef PubMed.
- X. H. Hu, X. Q. An and L. L. Li, Easy synthesis of highly fluorescent carbon dots from albumin and their photoluminescent mechanism and biological imaging applications, Mater. Sci. Eng. C, 2016, 58, 730–736 CrossRef PubMed.
- M. J. Krysmann, A. Kelarakis, P. Dallas and E. P. Giannelis, Formation mechanism of carbogenic nanoparticles with dual photoluminescence emission, J. Am. Chem. Soc., 2011, 134, 747–750 CrossRef PubMed.
- S. J. Zhu, Q. N. Meng, L. Wang, J. H. Zhang, Y. B. Song and H. Jin, et al., Highly photoluminescent carbon dots for multicolor patterning, sensors, and bioimaging, Angew. Chem., 2013, 125, 4045–4049 CrossRef.
Footnote |
† Electronic supplementary information (ESI) available. See DOI: 10.1039/c8ra03353f |
|
This journal is © The Royal Society of Chemistry 2018 |
Click here to see how this site uses Cookies. View our privacy policy here.