DOI:
10.1039/C8RA03324B
(Paper)
RSC Adv., 2018,
8, 20406-20410
Synthesis of phosphine oxide based amphiphilic molecules via ring-opening Wittig olefination of a macrocyclic phosphoranylidene and their property study as non-ionic surfactants†
Received
18th April 2018
, Accepted 25th May 2018
First published on 5th June 2018
Abstract
A novel ring-opening Wittig olefination approach was developed for the synthesis of amphiphilic phosphine oxides (PO) as non-ionic surfactants. The approach concurrently introduces the crucial functional groups (lipophilic chain and phosphine oxide moiety) present in the known PO surfactants and additional hydrophilic group (i.e., ethylene glycol units) in one step via Wittig olefination of a macrocyclic phosphoranylidene. A series of novel PO compounds were obtained from a variety of aldehydes and selected compounds were examined for their physiochemical properties (surface tension, critical micelle concentration and interfacial tension) and also for their abilities to form emulsions as non-ionic surfactants.
Introduction
Wittig olefination involving stabilized phosphorus ylides is a well-known chemical reaction as an efficient and robust C–C coupling strategy for organic synthesis1–3 as well as for protein bio-conjugation and labelling.4,5 However, the traditional scope of this reaction predominantly targets the synthesis of olefinic products wherein the phosphorus ylide works as an activation group only and phosphine oxide is generated as a by-product (Fig. 1, I), resulting in poor atom efficiency. A few exceptions were reported by Zhou's group who made use of the waste phosphine oxide in tandem reactions wherein it was generated from the first step Wittig reaction and sequentially worked as catalyst/co-catalyst in the next stereo-selective reaction step.6 In our efforts in developing green and efficient synthesis of specialty chemicals, we were inspired by a class of phosphine oxide (PO) based non-ionic surfactants.7 These PO surfactants, exemplified by alkyl dimethyl (CnDMPO) and alkyl diethyl (CnDEPO) phosphine oxides with typically C8–C14 alkyl chain (Fig. 2), have recently attracted renewed interests owing to their excellent detergency properties and potential industrial applications as surfactants/emulsifiers.7–9 Conventional PO based amphiphilic molecules contain only phosphine oxide as their hydrophilic heads, and their synthesis involve the preparation of a Grignard reagent dialkylphosphinylmagnesium bromide from diethyl phosphonate followed by further alkylation using alkyl bromide (Fig. 2).10 This method uses flammable Grignard reagent, and the target molecules have limited structural diversity. We envisioned that diversification for this family of surfactants can be conveniently achieved by utilizing a Wittig olefination strategy, which involves a macrocyclic phosphoranylidene intermediate (Fig. 1, II). This ring-opening Wittig olefination approach not only enables the convergence of two different molecular architectures, but also concurrently introduces a required phosphine oxide functionality and imparts additional hydrophilic group, i.e., oligomeric ethylene glycol, into the structure of the surfactant which further increases its hydrophilicity, and hence could potentially promote its surfactant properties. In this work, we examined the route for the synthesis of macrocyclic phosphoranylidenes and the following ring-opening Wittig reactions with a variety of aldehydes.
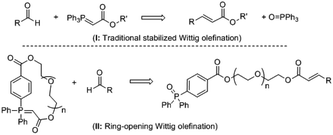 |
| Fig. 1 Traditional Wittig olefination (I) and modified ring-opening Wittig olefination from a macrocyclic triphenyl phosphoranylidene (II). | |
 |
| Fig. 2 Synthesis of alkyl dimethyl (CnDMPO) and alkyl diethyl (CnDEPO) phosphine oxides.10 | |
Results and discussion
As a proof of concept for the synthesis of a macrocyclic triphenyl phosphoranylidene, we began with the synthesis of cyclic phosphoranylidene 4a which bears a hexyl chain as linker. 6-Bromohexyl 2-bromoacetate (1a) and 1,4-diphenylphosphinobenzoic acid (2) were initially employed for this purpose. While the insertion of the bromoacetate 1a to 2 by heating (80 °C) in toluene provided the phosphonium salt 3a successfully (Scheme 1), the cyclization reaction of 3a to form cyclic phosphoranylidene 4a failed to proceed under various basic conditions (i.e., Et3N, iPr2NEt, K2CO3 and tBuOK). Considering the hexyl chain might not be sufficiently long for the cyclization to take place, nonyl bromoacetate (1b) was studied next.11 Indeed, cyclic triphenyl phosphoranylidene 4b was successfully obtained from triphenylphosphonium salt 3b upon base-mediated macrolactonization. The formation of 4b was confirmed by mass spectroscopy and subsequent formation of the Wittig olefination product 5 from 3-chlorobenzaldehyde in one pot, albeit in low yield (13%). By-product 5a (17%) was also isolated together, indicating the cyclization reaction was incomplete. Nevertheless, this demonstrated for the first time the reaction of ring-opening Wittig olefination of a cyclic phosphoranylidene.
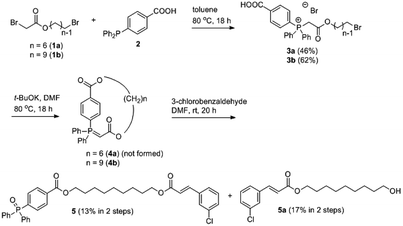 |
| Scheme 1 Synthesis towards a cyclic triphenylphosphoranylidene 4 and its olefination. | |
Taking into account that the presence of a hydrophilic moiety is required for the amphiphilicity of a non-ionic surfactant, tetraethylene glycol was introduced into the next synthesis as the spacer replacing the nonyl group (Scheme 2), wherein the cyclization method was also improved. The synthesis of the target molecule 9 commenced with the acylation of tetraethylene glycol with bromoacetyl bromide, which provided compound 6 in 49% yield. Heating bromide 6 with 4-(diphenylphosphaneyl)benzoic acid 2 provided the triphenylphosphonium salt 7 in a similar fashion. The initial attempt of macrocyclization via esterification was carried out using EDC/HOBt as coupling reagent. Similar to the result for the synthesis of 4b (Scheme 1), the formation of the desired cyclic phosphorus ylide 8 was confirmed by both MS and NMR analyses of the crude product. However purification of 8 by column chromatography became a challenge. Further examination of the crude product revealed that the cyclic ylide 8 was unstable and readily hydrolysed by the ambient water to produce the undesired ring-opening phosphine oxide 10 (Scheme 2). Non-stabilized phosphorus ylide is known to undergo alkaline hydrolysis or alcoholysis to form alkane/arene and phosphine oxide.12–14 However such hydrolysis/alcoholysis rarely happens for stabilized ylides due to its lower pKa as compared to water and alcohol, and indeed, Wittig olefinations carried out in aqueous media were commonly reported for linear stabilized phosphoranylidenes.3–5 The instability of ylide 8 towards hydrolysis is likely due to the conformational strain imposed on its P
C bond within the cyclic system. It is interesting that recently Byrne's group reported a new mechanism for the phosphorus ylide hydrolysis via concerted addition of O–H bond to P
C bond, which is particularly applicable to more acidic (or more stabilized) phosphorus ylide.15,16 Such degradation mechanism could explain the hydrolysis of 8 under ambient condition.
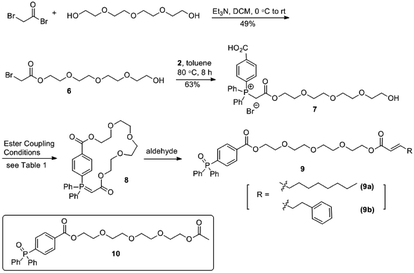 |
| Scheme 2 Synthesis of 9a and 9b via ring-opening Wittig olefination. | |
With this understanding, we next carried out the cyclisation and Wittig olefination reactions in one-pot without isolating the phosphoranylidene 8. However, the intramolecular coupling of 7 using EDC/HOBt/K2CO3 was inefficient and gave the Wittig products 9a and 9b in only 17% and 14% yields from nonanal and 3-phenylpropioaldehyde respectively in two steps (Entry 1 of Table 1). The use of organic base iPr2NEt instead of K2CO3 did not increase the yield. Upon switching coupling reagents to PyBOP/Cs2CO3, the yields of the same products 9a and 9b increased to 36% and 29% respectively (Entry 2). Further optimization of the reaction under slightly elevated temperature (40 °C) for Wittig olefination using DMF as the solvent improved the yields to 63% and 74% respectively (Entry 3).
Table 1 Optimization of intramolecular cyclization of 7 and the yields of Wittig olefination with nonanal and 3-phenylpropioaldehyde in two-step one-pot reactions
Entry |
Coupling condition |
Yield of 9a (%) |
Yield of 9b (%) |
1 |
EDC, HOBt, K2CO3, DCM, rt, 16 h |
17 |
14 |
2 |
PyBOP, Cs2CO3, DCM, rt, 16 h |
36 |
29 |
3 |
PyBOP, Cs2CO3, DMF, rt, 4 h, then 40 °C upon addition of aldehyde |
63 |
74 |
We next extended the substrate scope by subjecting a variety of aliphatic and aromatic aldehydes bearing different chain lengths, branches as well as electronic property to the ring-opening Wittig olefination. As shown in Table 2, linear aldehydes without substitution at 2-position gave higher yields than those with methyl substitution (71% of 9c vs. 48% of 9d), and no product of 9i was isolated from reaction with pivalaldehyde. This result is in consistent to the report that carbonyls with more steric hindrance work less efficiently in Wittig olefination.1 For comparison, substitution at 3-position (9f, 61% yield) rendered less influence on the reaction. Aldehydes with a longer aliphatic chain appeared less efficient than that with shorter chain (63% of 9a vs. 71% 9c) but this effect became marginal when the chain was further elongated (9a vs. 9e). The electronic property on the aromatic aldehydes seems to have no significant influence on their reactivity (9g vs. 9h), which is out of our expectation that aromatic aldehyde with electron-withdrawing group (9g) should be more reactive toward attacking the carbon anion of ylide 7 than that with electron-donating substitution (9h). This could indicate that the role of steric effect of the aldehyde overcomes its electrophilicity in the efficacy of Wittig olefination here.
Table 2 One-pot ring-opening Wittig olefination of various aldehydes
Having demonstrated a convenient route to novel phosphine oxides, compounds 9(a,e,f) (with longer lipophilic chain) were selected based on the estimated hydrophile–lipophile balance (HLB) values17 as potential non-ionic surfactants for physiochemical properties studies, i.e., surface tension, critical micelle concentration (CMC), interfacial tension (IFT), as well as the emulsification ability.
The surface tensions of the three compounds in aqueous phase were measured by tensiometry, and their critical micelle concentration (CMC) values were determined by plotting the curve of surface tension versus the logarithm of the concentration of the aqueous surfactant solution and the results are summarized in Table 3. The commercial non-ionic surfactant, polyoxyethylene monostearate (11) which contains ethylene glycol and alkyl units was used as reference for comparison of physiochemical properties. The measured CMC values of these synthetic surfactants fall within the reasonable range of a typical non-ionic surfactant CMC at about ×10−4 M and close to that of 11.18 Their surface tensions at CMC (γCMC) follow the similar trends of those known PO surfactants (CnDMPO) in that the surfactant having the lowest mass in hydrophobic chain (9a) gives the highest surface tension.7 Compound 9e shows the best surface activity with lowest surface tension (γCMC: 39.2 mN m−1) at CMC among the three, and is closer to that of the reference sample (11, γCMC: 36.4 mN m−1).
Table 3 Surface activity data for surfactants 9a, 9e, 9f and reference non-ionic surfactant 11
Compound |
γCMC (mN m−1) |
CMC (mmol L−1) |
9a |
42.9 |
0.291 |
9e |
39.2 |
0.532 |
9f |
40.6 |
0.591 |
11 |
36.4 |
0.184 |
The effect of surfactants to the interfacial tension (IFT) of a biphasic system was studied in water/n-decane interface for 9(a,e,f) and 11, the IFT values are listed in Table 4. It was shown that the three PO surfactants can reduce IFT of the system in approximately 3.5–4 fold as compared to that without surfactant (52 mN m−1), although weaker than reference compound 11.
Table 4 Interfacial tension of water/n-decane phase in the presence of surfactants (conc at 0.04 wt%) at 25 °Ca
Entry no |
Sample |
IFT (mN m−1) |
IFT of water/n-decane (at 25 °C) is 52 mN m−1.19 |
1 |
9a |
12.65 ± 0.50 |
2 |
9e |
15.08 ± 0.47 |
3 |
9f |
11.73 ± 0.33 |
4 |
11 |
2.97 ± 0.04 |
Surfactant 9a was further studied for its emulsification ability and compared with that of ref. 11. The biphasic mixture of water/n-decane (1
:
1) containing surfactant and rhodamine dye was homogenized using ultrasonic homogenizer for five minutes and the resulting emulsion was visualized by camera as well as optical microscopy. As shown in Fig. 3, both surfactants gave rise to emulsions with droplet size at micrometer range, albeit the emulsification by 9a is less efficient than that by 11, with a layer of n-decane still present at the top (Fig. 3a).
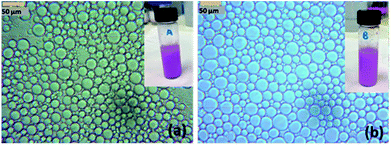 |
| Fig. 3 Optical microscopic (40×) and camera images taken after fresh preparation of emulsions via ultrasonic homogenization. (a) 9a/water/n-decane; (b) 11/water/n-decane (both with rhodamine dye for better visualization). | |
Conclusions
In summary, we have here demonstrated for the first time the reaction of ring-opening Wittig olefination of a cyclic phosphoranylidene, from which a convenient and efficient approach for the synthesis of novel functional phosphine oxides based amphiphilic molecules was developed. Preliminary physicochemical studies of selected compounds (9a, 9e, 9f) showed that they possess good surfactant properties and were capable of forming emulsion in a biphasic water/n-decane system, although further work remains to be done in terms of in depth property evaluation and structure-activity relationship studies. In the context of recently rekindled interest in the development of more advanced PO surfactants, the strategy we have developed here opens a new avenue for constructing novel scaffold of PO based surfactants.7
Conflicts of interest
There are no conflicts to declare.
Acknowledgements
The authors thank the Agency for Science, Technology and Research (A*STAR), Singapore for funding this work (SERC Grant No. 1528000049). We are also grateful to Prof. Alex M. van Herk for valuable discussion, Dr Ludger P. Stubbs, Mr Wenguang Zhao for providing lab instruments and technical supports.
Notes and references
- B. E. Maryanoff and A. B. Reitz, The Wittig olefination reaction and modifications involving phosphoryl-stabilized carbanions. stereochemistry, mechanism, and selected synthetic aspects, Chem. Rev., 1989, 89, 863 CrossRef.
- C. J. O'Brien, J. L. Tellez, Z. S. Nixon, L. J. Kang, A. L. Carter, S. R. Kunkel, K. C. Przeworski and G. A. Chass, Recycling the waste: the development of a catalytic Wittig reaction, Angew. Chem., Int. Ed., 2008, 48, 6836 CrossRef PubMed.
- A. El-Batta, C. Jiang, W. Zhao, R. Anness, A. L. Cooksy and M. Bergdahl, Wittig reactions in water media employing stabilized ylides with aldehydes. synthesis of α,β-unsaturated esters from mixing aldehydes, α-bromoesters, and Ph3P in aqueous NaHCO3, J. Org. Chem., 2007, 72, 5244 CrossRef PubMed.
- M.-J. Han, D.-C. Xiong and X.-S. Ye, Enabling Wittig reaction on site-specific protein modification, Chem. Commun., 2012, 48, 11079 RSC.
- K. M. Lum, V. J. Xavier, M. J.-H. Ong, C. W. Johannes and K.-P. Chan, Stabilized Wittig olefination for bioconjugation, Chem. Commun., 2013, 49, 11188 RSC.
-
(a) J.-J. Cao, F. Zhou and J. Zhou, Improving the atom efficiency of the Wittig reaction by a “waste as catalyst/co-catalyst” strategy, Angew. Chem., Int. Ed., 2010, 49, 4976 CrossRef PubMed;
(b) L. Chen, Y. Du, X.-P. Zeng, T.-D. Shi, F. Zhou and J. Zhou, Successively recycle waste as catalyst: A one-pot wittig/1,4-reduction/Paal–Knorr sequence for modular synthesis of substituted furans, Org. Lett., 2015, 17, 1557 CrossRef PubMed;
(c) X.-P. Zeng, Z.-Y. Cao, X. Wang, L. Chen, F. Zhou, F. Zhu, C.-H. Wang and J. Zhou, Activation of chiral (salen)AlCl complex by phosphorane for highly enantioselective cyanosilylation of ketones and enones, J. Am. Chem. Soc., 2016, 138, 416 CrossRef PubMed.
- C. Stubenrauch, N. Preisig and R. G. Laughlin, Phosphine oxide surfactants revisited, Adv. Colloid Interface Sci., 2016, 230, 2 CrossRef PubMed.
- J. T. Yoke III, T. Ariz and R. G. Laughlin. Tertiary phosphine oxide compounds, The Procter & Gamble Company, Cincinnati, Ohio, 1962, ser. no. 173, vol. 834, p. 5 Search PubMed.
- E. Carey and C. Stubenrauch, Foaming properties of mixtures of a non-ionic (C12DMPO) and an ionic surfactant (C12TAB), J. Colloid Interface Sci., 2010, 346, 414 CrossRef PubMed.
-
(a) R. G. Laughlin, The synthesis of unsymmetrical aliphatic phosphine oxides via diphenyl alkylphosphonates and Grignard reagents, J. Org. Chem., 1965, 30, 1322 CrossRef;
(b) H. R. Hays, Reaction of diethyl phosphonate with methyl and ethyl Grignard reagents, J. Org. Chem., 1968, 33, 3690 CrossRef.
- The use of 1,2-diphenylphosphinobenzoic acid in which the two groups are in closer proximity was also attempted in this reaction but failed to yield any desired macrocyclization product, and some unidentified by-products were obtained.
- M. Zanger, C. A. Vander Werf and W. E. McEwen, Kinetic study of the decomposition of quaternary phosphonium hydroxides, J. Am. Chem. Soc., 1959, 81, 3806–3807 CrossRef.
- W. E. McEwen, in Topics in Phosphorus Chemistry, ed. M. Grayson and E. J. Griffith, Interscience, New York, 1965, vol. 2, pp. 5–9 Search PubMed.
- H. J. Cristau and F. Plénat, in The Chemistry of Organophosphorus Compounds, ed. F. R. Hartley, Wiley, Chichester, 1994, vol. 3, pp. 111–138 Search PubMed.
- B. E. Maryanoff and A. B. Reitz, The mechanism of phosphonium ylide alcoholysis and hydrolysis: concerted addition of the O–H bond across the P
C bond, Chem.–Eur. J., 2016, 22, 9140 CrossRef PubMed. - P. A. Byrne, Y. Ortin and D. G. Gilheany, First ever observation of the intermediate of phosphonium salt and ylide hydrolysis: P-hydroxytetraorganophosphorane, Chem. Commun., 2015, 51, 1147 RSC.
- H. Mollet and A. Grubenmann. Formulation technology: emulsions, suspensions, solid Forms: Chapter 2. Emulsions – properties and production, Wiley-VCH Verlag GmbH, 2001, p. 70 Search PubMed.
- S. K. Hait and S. P. Moulik, Determination of critical micelle concentration (CMC) of nonionic surfactants by donor–acceptor interaction with Iodine and correlation of CMC with hydrophile–lipophile balance and other parameters of the surfactants, J. Surfactants Deterg., 2001, 4, 303 CrossRef.
- S. Zeppieri, J. Rodríguez and A. L. L. de Ramos, Interfacial tension of alkane + water systems, J. Chem. Eng. Data, 2001, 46, 1086 CrossRef.
Footnote |
† Electronic supplementary information (ESI) available: Experimental details for compound synthesis and characterisation, methods for property studies; copies of NMR spectra. See DOI: 10.1039/c8ra03324b |
|
This journal is © The Royal Society of Chemistry 2018 |
Click here to see how this site uses Cookies. View our privacy policy here.