DOI:
10.1039/C8RA03070G
(Paper)
RSC Adv., 2018,
8, 19786-19790
Carbon dots as fluorescent probes for detection of VB12 based on the inner filter effect†
Received
10th April 2018
, Accepted 11th May 2018
First published on 29th May 2018
Abstract
In this study, we constructed a new fluorescent sensing for VB12 and investigated the mechanism of vitamin B12 (VB12) quenching fluorescence of carbon dots (CDs). The fluorescence suppression is attributed to the inner filter effect (IFE) because of the overlap between UV-vis absorption spectrum of VB12 and emission/excitation spectra of CDs. This CDs-based sensor provides obvious advantages of simplicity, convenience, rapid response, high selectivity and sensitivity, which has potential application for the detection of VB12 in the medical and food industry.
Introduction
Vitamin B12 (VB12), also called cobalamin, is an essential water soluble nutrient that can be found in foods such as meat, eggs and dairy products. It can effectively prevent diseases such as pernicious anemia, senile dementia, depression. Therefore, VB12 plays an integral role in keeping the human body healthy. However, excess VB12 can also produce toxic side effects, such as a lack of folic acid. So, the detection of VB12 is very important.
In the past years, numerous analytical methods for the determination of VB12 in different sample matrices have been successfully developed, including microorganism method, HPLC-UV, atomic absorption spectroscopy, thin layer chromatography, etc. However, most of these methods are not perfect due to the requirement of expensive equipment, being time consuming or complicated sample pretreatment. Therefore, a simple, sensitive, and selective method for VB12 detection is highly demanded. Fluorescence analysis has been applied in the detection of VB12 due to its high sensitivity and easy operation. In 2014, Kolekar's group1 detected VB12 based on FRET between CdS and VB12, and this method could be used in serum and urine actual samples. Other groups used rhodamine B2 or gold nanoclusters modified by bovine serum protein3 as fluorescent probes to detect VB12. However, these methods had the following problems: the fluorescent probes had biological toxicity, the operations were complex and the sensitivities were not high. So choosing a green fluorescent probe and a simple operation can help constructing a better fluorescent method to detect VB12.
Compared with traditional quantum dots and fluorescent dyes, carbon dots (CDs) have a series of unique properties such as excellent water solubility, anti-photobleaching, easy of modification, low toxicity, low cost, tunable excitation and emission spectra.4–7 These attractive features indicate the prominent advantages of CDs in chemical sensing, biosensing, bioimaging, nanomedicine and catalyst. CDs have been applicated in the sensing of ions (such as Hg2+, Cu2+, Fe3+, F− and BrO3−, etc.),8–12 molecular substances (for instance, glucose,13 hemoglobin,14 p-nitrophenol15 etc.) and pH.16 The mechanisms of CDs-based fluorescence sensor include photo induced electron transfer (PET), intramolecular charge transfer (ICT), fluorescence resonance energy transfer (FRET) and twisted intramolecular charge transfer (TICT), etc. Inner filter effect (IFE) is also a mechanism of fluorescence sensing. The IFE refers that the excitation and emission spectra of fluorophores are absorbed by absorbers, then the fluorophores suppress.17 In the beginning, IFE was an inevitable error, the research on the IFE mainly focused on the correction of IFE. Since metal nanoparticles have good absorption coefficient, they can be used as good absorbers.18 Now, the IFE has been developed into an analytical method.19–24 Fluorescence detection based on IFE includes two forms. IFE can happen between metal nanoparticles and other fluorophores, and an analyte can make fluorescence recovery. IFE can also happen between an analyte and fluorophores, and the analyte can be detected based on fluorescence quenching. In this study, VB12 was detected based on the IFE between VB12 and CDs. This method has an extremely low LOD for detecting VB12 of 93 nM, and the detection system is simple and fast.
Experimental
Reagents and equipments
Ammonium citrate (AR) and all the coexisting vitamins were obtained from Aladdin Chemistry Company Limited (Shanghai, China). Vitamin B12 (VB12) was purchased from Beijing Fangcao Medicine Chemical Industry Developed Company. NaH2PO4·2H2O, Na2HPO4·12H2O, NaOH, H3PO4, H3BO3, CH3COOH and all the coexisting ions were obtained from Guoyao Company (Shanghai, China). VB12 tablets were purchased from Yunpeng Medical Company (Shanxi, China) and VB12 injections were obtained from Ruicheng Tiantong Company Limited (Shanxi, China). All chemicals were used in the experiments without further purification. Deionized water, purified by Millipore system (18.0 MΩ cm at 25 °C), were employed for all experiments.
pH was measured by a Model 1828 digital pH meter. PL spectra were acquired by a Hitachi F-7000 spectrometer. UV-vis absorption spectra were recorded by a Shimadzu UV-2600 spectrophotometer. FTIR-4800S spectra were employed for obtaining IR spectra in KBr discs in the 4000–400 cm−1 region. X-Ray Diffraction (XRD) results were recorded on a Rigaku Smart lab with a speed of 6° per minute. Transmission electron microscopy (TEM) experiments were done on a TECNAI-F30 system. Zeta potential and dynamic light scattering (DLS) size distribution were obtained by a Zetasizer Nano.
Preparation of CDs
The fluorescent CDs were prepared through a simple and low-cost hydrothermal treatment.19 Briefly, 2.0 g of ammonium citrate was added into 25 mL distilled water. The solution was heated from room temperature to 160 °C in a 50 mL para polyphenol (PPL) equipped stainless steel autoclave and held at 160 °C for 6 h. The color of the solution gradually turned into deep blue from colorless in appearance. When the resulting solution was cooled to room temperature, the solution was placed in the fridge for further application. The product can be used directly without any further passivation or purification.
Vitamin B12 sensing
For sensing vitamin B12 (VB12), 1.2 μM CDs solution and 2 mL 0.2 M PB buffer solution of pH 7.0 were mixed with the solution containing VB12 of various concentration to afford a fixed volume of 5 mL. After stirring, the mixed solution was maintained at room temperature for 15 min, PL spectra were measured. Moreover, the experiments of coexistence of vitamins or heavy metal ions were conducted for further investigating the selectivity under similar experimental conditions of this sensing system. The excitation wavelength was set as 350 nm.
Interference experiments of VB12
To investigate the effects of other coexisting substances to VB12 detection, some vitamins (VB1, VB3, VB5, VB7, VB9, VC) and some metal ions (Ba2+, Ca2+, Cd2+, Co2+, Cr3+, Cu2+, Zn2+, Ni2+, Mn2+, Fe2+, Fe3+, Hg2+, Pb2+) were added to CDs solution with VB12. The concentration of Hg2+ was 10 μM, Fe2+ and Fe3+ were 40 μM. Then vitamins and other metal ions were 100 μM.
Results and discussion
Synthesis and characterization of CDs
The CDs with blue fluorescent were prepared by a hydrothermal treatment. The optical properties of the CDs are shown in Fig. S1.† As shown in the UV-vis absorption spectra, one absorption peak was at 234 nm, due to π → π* transition of C
C bond,20 and another peak at 338 nm was attributed to n → π* transition of C
O bond.21 The photoluminescence (PL) spectra of CDs showed that the maximum peak centered at 440 nm under a 350 nm excitation wavelength, which presented a bright blue color under UV lamp. And the emission spectra didn't shift red with the excitation changing.
The QY of CDs was 0.24 by calibrating against reference quinine sulfate in H2SO4 and the lifetime was 6.52 ns fitting with single index. From Fig. 1, we found that the as-prepared CDs had an average size of 3.03 nm and they were mono-dispersed. XRD showed the CDs had 002 facet which means graphene layers. The FTIR spectrum showed the surface of CDs exist amide functional, hydroxyl groups and carboxyl groups. Zeta potential results showed a value of −11.73 mV, probably resulted from the slight ionization of hydroxylgroup of the surface of the resulting CDs. DLS measurement revealed that the CDs particles had good size distribution with an average size of 9.68 nm. The synthesized CDs had good light stability, as showed in Fig. S2.†
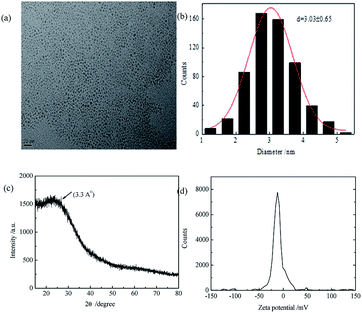 |
| Fig. 1 (a) TEM image of CDs; (b) particle size distribution of CDs; (c) XRD pattern of CDs (d) zeta potential of CDs. | |
The optimization of important factors for the probe
The effects of pH on the PL of CDs were investigated. As shown in Fig. 2(a), under acidic pH, the PL intensity of CDs decreased. This could be attributed to the carboxyl groups on the CDs surface accumulated by combining the protons. The PL intensity and the quenching kept almost constant in the pH range of 6.0–8.0. In this experiment, we selected pH = 7.0. Types of buffer were also investigation. In PB buffer, the most quenching was obtained as shown in Fig. 2(b). Then the reaction was fully completed within 15 min, as shown in Fig. 2(c), suggesting a rapid method to detect VB12. The CDs concentration was investigated and the optimal concentration was 1.2 μM.
 |
| Fig. 2 (a) PL intensity of CDs in the absence (black) and presence (red) of 15 μM VB12 in BR buffer solution of various pHs; (b) PL intensity of CDs in the absence (black) and presence (red) of 15 μM VB12 in different buffer solutions; (c) time-dependent fluorescence intensity of CDs to 15 μM VB12; (d) effect of CDs concentration on fluorescence quenching. | |
Establishment of the sensing system for VB12
Fig. 3(a) shows the PL response of the CDs towards VB12. It was found that the PL intensity of CDs gradually decreased with increasing VB12 concentrations (0, 0.3, 0.5, 2.0, 4.0, 6.0, 8.0, 10.0, 12.0, 15.0 μM). Fig. 3(b) shows a linear correlation curve could be fitted between VB12 concentration and (F0 − F)/F0. In which F0 is the PL intensity of CDs without VB12 and F with different VB12 concentrations. A good linear range was within 0.3–15 μM and the linear correlation coefficient could be obtained to be 0.9940. The limit of detection (LOD) was also calculated to be 93 nM, according to the equation of LOD = 3σ/k, in which σ is the standard deviation from 11 blank solutions and k is the linear slope fitted. Compared with other methods of fluorescence detection of vitamin B12, this method is green environment and less pollution. The detection system is simple and fast, the linear range is wide and the sensitivity is high, as shown in Table 1.
 |
| Fig. 3 (a) PL spectra of CDs in VB12 with different concentrations; (b) the relationship curve between (F0 − F)/F0 and VB12 concentration. | |
Table 1 Comparison of our proposed method and the reported cases to detect VB12 based on PL
Sensor |
Linear range |
LOD |
Ref. |
Graphene oxide nanolayer |
0–1.08 μM |
320 nM |
1 (ref. 18) |
CdS |
3.7–73.8 μg mL−1 |
5.1 μM |
2 (ref. 19) |
CdTe |
0.7–1.8 μM |
0.1 μM |
3 (ref. 20) |
CDs |
0.3–15 μM |
93 nM |
This work |
Effect of coexisting substances in the determination of VB12
In order to explore the possibility of practical application in the determination of VB12, the interferences from other vitamins and anions were tested under the optimized conditions. Fig. 4 showed other vitamins have no influence to the detection of VB12. And Table 2 showed that some anions quenched the fluorescence of CDs, such as Hg2+. From Fig. S3,† the coexisting Hg2+ could suppress the PL intensity of CDs heavily while the effect of Hg2+ coexisting could be eliminated by adding EDTA.
 |
| Fig. 4 Selectivity of CDs for VB12 over other vitamins (VB12 concentration was 8 μM. VB9 was 10 μM and other vitamins were 100 μM). | |
Table 2 Effect of metal irons (8 μM) on the detection of VB12
Coexisting substance |
Concentration (μmol L−1) |
Change of fluorescence intensity (%) |
Ba2+ |
100 |
+0.01 |
Ca2+ |
100 |
+3.50 |
Cd2+ |
100 |
+3.07 |
Co2+ |
100 |
+5.13 |
Cr3+ |
100 |
+2.42 |
Pb2+ |
100 |
−5.31 |
Cu2+ |
100 |
+0.77 |
Zn2+ |
100 |
+0.80 |
Ni2+ |
100 |
+2.09 |
Mn2+ |
100 |
+3.60 |
Fe2+ |
40 |
+2.81 |
Fe3+ |
40 |
+2.87 |
Hg2+ |
10 |
−73.60 |
Fluorescence quenching mechanism of CDs by VB12
It was found that the fluorescence of CDs could be quenched by VB12, revealing the possibility of applying the as-prepared CDs as a sensitive fluorescent sensor of VB12. From the spectra in Fig. 5, we could see a good spectral overlap between the absorption spectrum of VB12 and the excitation and emission spectra of CDs, suggesting that the fluorescence quenching might be related to FRET or IFE. Since lifetimes of CDs both in the absence and presence of VB12 remained unchanged (Fig. 6), fluorescence quenching was unreasonable ascribed to the FRET process. Then there wasn't blue shift or red shift of CDs emission which shows no interaction appeared between CDs and VB12. Furthermore, the selectivity toward VB12 could be explained by the IFE mechanism (Fig. 7). The IFE can be estimated according to the following equation.25 |
 | (1) |
wherein, Fobsd is the measured maximum fluorescence intensity and Fcor is the corrected maximum fluorescence intensity by removing IFE from Fobsd. Aex and Aem represent the absorbance at the excitation wavelength (λex = 350 nm) and maximum emission wavelength (λem = 446 nm), respectively; s is the thickness of excitation beam (0.10 cm26,27); g is the distance between the edge of the excitation beam and the edge of the cuvette (0.40 cm) and d is the width of the cuvette (1.00 cm). In Table S1,† CF (correction factor) was that Fcor/Fobsd, which could be calculated by eqn (1.1). In order to ensure the credibility of the correction, the maximum value of CF could not exceed 3. Fcor was the PL intensity of CDs with different VB12 concentrations after IFE correction and it could be calculated by eqn (1). Fcor,o was the PL intensity of CDs without VB12 after IFE correction and in Table S1† it was 964.95. Fcor,o/Fcor could reflect the degree of quenching after IFE correction. The closer the ratio was to 1, the greater the effect of the IFE. E% (E = 1 − F/F0) was suppressed efficiency, F0 and F were the FL intensities of CDs in the absence and presence of VB12, respectively. In Fig. 8, we found that approximately all of the suppressed effect came from the IFE of VB12. After removing the IFE, there was almost no quenching in linear range. Therefore, approximately all of the quench effects come from the IFE of VB12.
 |
| Fig. 5 Normalized UV-vis absorption spectrum of VB12, excitation and emission spectra of CDs. | |
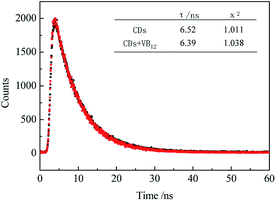 |
| Fig. 6 Lifetime of CDs in the absence (black) and presence (red) of 4 μM VB12. | |
 |
| Fig. 7 Schematic illustration of the detection of VB12 by CDs. | |
 |
| Fig. 8 Suppressed efficiency (E%) of observed (black) and corrected (red) measurements for CDs after each addition of different concentrations of VB12. | |
The correction factor at each concentration of VB12 thus could be calculated (Table S1†), Fcor,o. is the corrected fluorescence intensity in the absence of VB12. After the IFE was removed from the totally observed suppressed fluorescence, the suppressed efficiency E = 1 − F/F0, for the totally observed and the corrected fluorescence of VB12 is figured out, as shown in Fig. 8. We found that approximately all of the quench effects come from the IFE of VB12.
Practical application
The method in real application was also investigated in the determination of VB12 in injections and tablets samples. VB12 samples of various known concentrations were employed to conduct the recovery experiments. The experimental results were summarized in Table 3. The recoveries ranging from 93.3% to 109.2% were acceptable which indicated our method had good reliability of the sensing system.
Table 3 Detection of VB12 in real samples (n = 3)
Samples |
Added (μM) |
Spiked (μM) |
Found (μM) |
Recovery (%) |
RSD (%) |
Injections |
1.8 |
3.0 |
4.7 |
96.7 |
1.27 |
1.8 |
8.0 |
9.6 |
97.5 |
1.19 |
1.8 |
13.0 |
14.2 |
95.4 |
0.67 |
Tablets |
1.8 |
3.0 |
4.6 |
93.3 |
0.96 |
1.8 |
8.0 |
9.7 |
98.8 |
0.51 |
1.8 |
13.0 |
16.0 |
109.2 |
1.62 |
Conclusions
In this paper, a fluorescence analysis method for the detection of VB12 was established. The fluorescence suppression is attributed to inner filter effect because of the overlap between UV-vis absorption spectrum of VB12 and emission/excitation spectra of CDs. There is a good linear relation between VB12 concentration and (F0 − F)/F0 with the LOD is 93 nM. Compared with other method of fluorescence detection of VB12, this method is green environment and less pollution. The detection system is simple and fast, the linear range is wider and the sensitivity is higher.
Conflicts of interest
There are no conflicts to declare.
Acknowledgements
This work is financially supported by the Natural Science Foundation of China (No. 21275059 and 21575044), the Natural Science Foundation of Fujian Province (No. 2015J01054 and 2016J01062), Foundation of Graphene Powder & Composite Research Center of Fujian Province (2017H2001).
References
- A. H. Gore, M. B. Kale, P. V. Anbhule, S. R. Patil and G. B. Kolekar, RSC Adv., 2014, 4, 683 RSC.
- H. Xu, Y. Li and C. Liu, Talanta, 2008, 77, 176 CrossRef PubMed.
- F. Samari, B. Hemmateenejad and Z. Rezaei, Anal. Methods, 2012, 4, 4155 RSC.
- M. Zheng, Z. G. Xie, D. Qu, D. Li, P. Du, X. B. Jing and Z. C. Sun, ACS Appl. Mater. Interfaces, 2013, 5(24), 13242 Search PubMed.
- X. Miao, D. Qu, D. X. Yang, B. Nie, Y. K. Zhao, H. Y. Fan and Z. C. Sun, Adv. Mater., 2018, 30, 1704740 CrossRef PubMed.
- D. Qu, X. Miao, X. T. Wang, C. Nie, Y. Li, L. Luo and Z. C. Sun, J. Mater. Chem. B, 2017, 5, 4988 RSC.
- X. Miao, X. L. Yan, D. Qu, D. B. Li, F. F. Tao and Z. C. Sun, ACS Appl. Mater. Interfaces, 2017, 9, 1854 Search PubMed.
- A. Gupta, A. Chaudhary and P. Mehta, Chem. Commun., 2015, 51, 10750 RSC.
- X. Liu, N. Zhang and T. Bing, Anal. Chem., 2014, 86, 2289 CrossRef PubMed.
- H. Zhang, Y. Chen and M. Liang, Anal. Chem., 2014, 86, 9846 CrossRef PubMed.
- X. Y. Sun, L. L. Wu and J. S. Shen, RSC Adv., 2016, 6, 97346 RSC.
- P. Li, X. Y. Sun and J. S. Shen, RSC Adv., 2016, 6, 61891 RSC.
- P. Shen and Y. Xia, Anal. Chem., 2014, 86, 5323 CrossRef PubMed.
- A. Barati, M. Shamsipur and H. Abdollahi, Biosens. Bioelectron., 2015, 71, 470 CrossRef PubMed.
- H. Yuan, J. Yu and S. Feng, RSC Adv., 2016, 6, 15192 RSC.
- T. Zhang, Y. Zhai and H. Wang, RSC Adv., 2016, 6, 61468 RSC.
- P. Yuan and D. R. Walt, Anal. Chem., 1987, 59, 2391 CrossRef.
- H. Li and X. Yang, Anal. Methods, 2015, 7, 8445 RSC.
- Z. Yang, M. Xu and Y. Liu, Nanoscale, 2014, 6, 1890 RSC.
- Y. Park, J. Yoo and B. Lim, J. Mater. Chem. A, 2016, 4, 11582 Search PubMed.
- M. Chen, W. Wang and X. Wu, J. Mater. Chem. B, 2014, 2, 3937 RSC.
- J. Gholami, M. Manteghian and A. Badiei, Fullerenes, Nanotubes, Carbon Nanostruct., 2015, 23, 878 CrossRef.
- A. H. Gore, M. B. Kale and P. V. Anbhule, RSC Adv., 2014, 4, 683 RSC.
- E. Vaishnavi and R. Renganathan, Spectrochim. Acta, Part A, 2013, 115, 603 CrossRef PubMed.
- M. M. Puchalski, M. J. Morra and R. Von Wandruszka, Fresenius. J. Anal. Chem., 1991, 340, 341 CrossRef.
- X. Zhu, T. Zhao and Z. Nie, Anal. Chem., 2015, 87, 8524 CrossRef PubMed.
- W. Zhai, C. Wang and P. Yu, Anal. Chem., 2014, 86, 12206 CrossRef PubMed.
Footnote |
† Electronic supplementary information (ESI) available. See DOI: 10.1039/c8ra03070g |
|
This journal is © The Royal Society of Chemistry 2018 |