DOI:
10.1039/C8RA02656D
(Paper)
RSC Adv., 2018,
8, 21926-21932
Synthesis of high cis-1,4 polybutadiene with narrow molecular weight distribution via a neodymium-based binary catalyst
Received
27th March 2018
, Accepted 5th June 2018
First published on 14th June 2018
Abstract
This work explores the synthesis of polybutadiene (PB) with high cis-1,4 unit content and narrow molecular weight distribution using a neodymium-based complex, consisting of neodymium(III) trifluoromethane sulfonate (Nd(CF3SO3)3) and coordinated with tris(2-ethylhexyl)phosphate (TOP). The complex's composition and structure are characterized by elemental analysis, XPS and FT-IR. Combined with Al(i-Bu)3, a binary catalyst is obtained and then applied to initiate the polymerization of butadiene. Results indicate that the catalyst system displays a high catalyst activity and the product polymer (PB) features a high cis-1,4 content (98.8%) and a remarkably narrow molecular weight distribution (Mw/Mn = 1.32). Compared with PB initiated by the current well-studied catalyst Nd(CF3SO3)3*3TBP/Al(i-Bu)3, the Nd(CF3SO3)3*3TOP allows significantly narrowing the molecular weight distribution of the PB, because the Nd(CF3SO3)3*3TOP is more easily dissolved in hexane. Therefore, butadiene was polymerized in a homogenous system and each monomer's polymerization was initiated in the single molecular state.
1. Introduction
Butadiene rubber (PB, high cis-1,4 unit) is one of the most important synthetic rubbers after styrene-butadiene rubber. Due to its regularity and highly linear molecular structure, butadiene rubber exhibits unique properties such as excellent elasticity, low heat built-up and strong resistance versus abrasive and fatigue stress.1,2 Recently it was shown that the cis-1,4 unit content in PB is an extremely important factor and is beneficial for the strain-induced crystallization of rubbers.3–5 Consequently, by increasing the cis-1,4 unit content, not only are PB's tensile strength and elongation ratio mutually increased, but the fatigue and crack resistance are also significantly improved.6,7 Therefore, strategies urgently need to be developed to raise the cis-1,4 unit content in PB rubbers. In parallel to this aspect, studies are devoted to narrowing the PB molecular weight distribution (Mw/Mn < 3.0), which was directly related to the uniformity of the vulcanized cross-linked network, and was shown to strongly affect the physical properties of PB.8–15 Recent research showed that PB with high molecular weight, narrow molecular weight distribution (<1.1) and high cis-1,4-regularity (>99%) could be synthesized by using a series of cationic alkyl rare-earth metal catalysts. However, these catalysts are very complicated and expensive, which limited the industrial application of the catalysts.16–19
Neodymium sulphonate is a simple and easily obtained rare earth compound, having the advantage of possessing a high catalytic activity and good directional function for the butadiene polymerization. It was already reported20,21 that rare earth based binary catalysts can be synthesized by combining neodymium sulphonate with alkyl aluminum. Recently, Hu and his team22 synthesized the complex Nd(CF3SO3)3*3TBP using neodymium(III) trifluoromethane sulfonate (Nd(CF3SO3)3) and tributyl phosphate (TBP) under reflux in THF. After combining this compound with Al(i-Bu)3, a binary catalyst was formed which was shown to effectively initiate the polymerization of butadiene. Furthermore, cis-1,4 unit content in the product was demonstrated to be up to 99%. Unfortunately, the associated molecular weight distribution (Mw/Mn > 5) was relatively broad. The authors concluded that as Nd(CF3SO3)3*3TBP was difficult to be thoroughly dissolved in aliphatic solvent, the obtained catalyst system might not form a homogeneous solution with butadiene monomers. As a result, the initiation of the polymerization performed in the hetero phase state, which allowed the final product having a broader molecular weight distribution.
Based on the previous results, with the aim of synthesizing PB with both high cis-1,4 unit content and narrow molecular weight distribution in this paper, we developed a new rare earth based catalyst in which Nd(CF3SO3)3 was utilized to synthesize a more stable complex by using a long-alkyl segment compound, tris(2-ethylhexyl)phosphate (TOP). The main purpose was to tentatively enhance the solubility of the complex in cyclohexane. The complex was then combined with Al(i-Bu)3, and a binary catalyst, Nd(CF3SO3)3*3TOP/Al(i-Bu)3, was prepared. This catalyst is proved to possess the ability not only to promote the increase of 1,4 unit content but also to effectively narrow the molecular weight distribution of PB. The related structure characterizations of the catalyst and PB are provided, together with the discussions on the relationship between the catalyst properties and the polymer chain propagation mechanism.
2. Experimental section
2.1 Materials
Neodymium(III)trifluoromethane sulfonate (Nd(CF3SO3)3, 97%), was purchased from TCI, Japan. Triisobutylaluminum (Al(i-Bu)3, 1 M in hexane) was purchased from J&K, China, and used as received. Tris(2-ethylhexyl)phosphate (TOP, 98%), tributylphosphate (TBP, 98%) and 2,6-di-tert-butyl-p-cresol (Antiager 264, CP) were purchased from Aladdin Bio-Chem Technology, China. 1,3-butadiene (Bd, 1.9 M in hexane) was obtained from Energy, China. Cyclohexane was distilled with calcium hydride and stored with 4 Å molecular sieve for one week before use. Methanol (AR) was used as received.
2.2 Synthesis of Nd(CF3SO3)3*3TOP complex
Under argon atmosphere, Nd(CF3SO3)3 1.00 g (1.7 mmol) and TOP 2.20 g (5.1 mmol) were put in a dry three-necked flask. The mixture was then heated to 120 °C stirred for 12 hours. The product was then washed three times with methanol and dried under vacuum at 60 °C for 12 hours to obtain a reddish and viscous liquid. The process yield was 90.7%. The resulting product was dissolved in cyclohexane to form a stable complex solution with the concentration of 0.025 M.
TOP: IR (KBr, cm−1): 2961, 2930, 2866, 1464, 1283, 1026. Nd(CF3SO3)3*3TOP: IR (KBr, cm−1): 2961, 2930, 2866, 1464, 1225, 1032, 636. Anal. calcd for C75H153O21P3NdS3F9: C, 47.53%; S, 5.08%; H, 8.14%. Found: C, 46.98%; S, 5.19%; H, 7.96%.
2.3 Synthesis of Nd(CF3SO3)3*3TBP complex
For comparison, we synthetized the Nd(CF3SO3)3*3TBP complex under the same conditions. In argon atmosphere, 1.00 g (1.7 mmol) of Nd(CF3SO3)3 and 1.35 g (5.1 mmol) of TBP were added in a dried three-necked flask. The mixture was heated to 120 °C stirred for 12 hours. The product obtained was washed with methanol three times and dried under vacuum at 60 °C for 12 hours to obtain a viscously reddish liquid. The process yield was 92.5%. The resulting complex was dissolved in cyclohexane to give a catalyst mixture solution with the concentration of 0.025 M.
TBP: IR (KBr, cm−1): 2963, 2930, 2874, 1464, 1279, 1029. Nd(CF3SO3)3*3TBP: IR (KBr, cm−1): 2962, 2935, 2873, 1463, 1223, 1033, 638. Anal. calcd for C39H81O21P3NdS3F9: C, 33.69%; S, 6.92%; H, 5.87%. Found: C, 33.46%; S, 6.98%; H, 5.83%.
2.4 Preparation of binary catalyst and polymerization of PB
All synthesis operations were conducted in dry argon atmosphere. The detailed typical polymerization procedure is provided in Table 1 (run 2). Specifically, a binary catalyst solution was prepared in a Schlenk tube with a rubber septum by the following procedure: butadiene (0.16 mL, 1.9 M solution in hexane), Nd(CF3SO3)3*3TOP (0.4 mL, 0.025 M solution in cyclohexane) and Al(i-Bu)3 (0.2 mL) with [Al]/[Nd] = 20 were mixed in the reaction tube and aged with stirring at 50 °C for 15 min to obtain a yellowish catalyst solution.
Table 1 XPS data for Nd(CF3SO3)3, complex of Nd(CF3SO3)3*3TOP and Nd(CF3SO3)3*3TBP
Compound |
Bonding energy (eV) |
Nd 3d5/2 |
O 1s |
P 2p |
Nd–O |
Nd–O–S |
S O |
P–O |
P–O |
Nd(CF3SO3)3 |
980.5 |
529.7 |
530.7 |
— |
— |
Nd(CF3SO3)3*3TOP |
978.5 |
530.9 |
531.8 |
532.4 |
133.5 |
Nd(CF3SO3)3*3TBP |
978.6 |
531.0 |
531.3 |
532.5 |
133.7 |
Then butadiene (10.5 mL, 1.9 M solution in hexane) with ([Bd]/[Nd] = 2000) was injected, using a medical injector, into the Schlenk tube filled with the binary catalyst solution (0.013 M, 0.76 mL). The polymer synthesis was carried out at 20 °C for 2 hours and then quenched by adding 2 mL ethanol containing 2,6-di-tert-butyl-p-cresol (1 wt%) as a stabilizer. The product was precipitated in methanol and repeatedly washed with ethanol. The product was dried under vacuum at 40 °C to reach a constant weight. Finally, a white solid was obtained. The polymerization yield was 88.2%.
2.5 Characterization of the catalyst structure
X-ray photoelectron spectroscopy (XPS) was carried to measure the composition of the complexes including Nd(CF3SO3)3*3TOP, Nd(CF3SO3)3*3TBP and the pure Nd(CF3SO3)3 compound. The instrument's model is K-Alpha spectrometer (Axis Ultra, Kratos Analytical Ltd., U.K.). A monochromatic Al Kα with 150 W working power was used as the X-ray source (hν = 1486.7 eV). Binding energies were referred to the adventitious hydrocarbon C 1s line at 285.0 eV.
Bruker Nicolet iS10 FT-IR spectrophotometer was used to compare the structural modification of complexes between TOP and TBP before and after coordinating the molecular interaction. The sample preparation consisted on coating a KBr disc with the complex solution, and then followed by the evaporation of cyclohexane.
Elementar Vario III elemental analyzer was employed for elemental analysis on the complexes.
31P NMR spectra of Nd(CF3SO3)3*3TOP was recorded with Bruker 400 MHz at room temperature using DMSO as the solvent and tetramethylsilane (TMS) as the internal reference. The 1H NMR spectra of La(CF3SO3)*3TOP with Al(i-Bu)3 were recorded with Bruker 400 MHz using C6D6 as the solvent and tetramethylsilane (TMS) as the internal reference.
2.6 Characterization of PB
The cis-1,4 unit content in PB was determined by FT-IR analysis to measure. The content of cis-1,4, trans-1,4 and 1,2-unit were measured via IR absorption bands at 738, 967 and 911 cm−1, respectively. The calculation equations were used as reported in the literature.23
1H NMR spectra were recorded with Bruker 400 MHz using CDCl3 as the solvent and tetramethylsilane (TMS) as the internal reference at room temperature.
The number-average molecular weights and polydispersity indices (Mw/Mn) of PBs were determined from DAWN EOS size exclusion chromatography/multi-angle light scatter instrument (SEC-MALLS, Wyatt Technology). We used HPLC grade THF as the eluent at a flow rate of 0.5 mL min−1 at 25 °C.
3. Results and discussion
3.1 Catalyst synthesis and characterization
In order to improve the solubility of Nd(CF3SO3)3*3TBP and narrow the resulting PB molecular weight distribution, TBP was replaced by TOP, which displays a longer alkyl segment. For purpose of reducing the influence of the solvent THF on the coordination process of Nd(CF3SO3)3 and ligand TOP, thereby forming a well-defined complex in the solvent, Nd(CF3SO3)3 was directly reacted with TOP without addition of any solvent at 120 °C for 12 hours. Compared to the synthesis route22 of Nd(CF3SO3)3*3TBP which needed refluxing in THF for 12 hours, Nd(CF3SO3)3*3TOP synthesis approach was much simpler. The resulting product could also be easily dissolved in cyclohexane to form a stable complex solution. The reaction route is presented in Scheme 1.
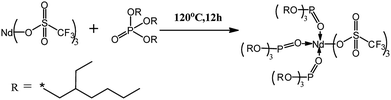 |
| Scheme 1 Synthesis route of Nd(CF3SO3)3*3TOP. | |
For verifying the chemical composition of Nd(CF3SO3)3*3TOP complex, XPS was employed to conduct the surface characterization, the spectra are shown in Fig. 1, and the data of XPS peak-differentiating are listed in Table 1.
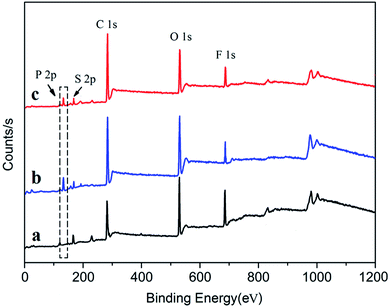 |
| Fig. 1 XPS spectra of complex Nd(CF3SO3)3*3TOP, Nd(CF3SO3)3*3TBP and Nd(CF3SO3)3 compound. (a) Pure Nd(CF3SO3)3 compound. (b) Nd(CF3SO3)3*3TBP. (c) Nd(CF3SO3)3*3TOP. | |
According to the Fig. 1(c) and Table 1, the characteristic P 2p peak in Nd(CF3SO3)3*3TOP results at 133.5 eV, and the deconvolution of the O 1s spectrum confirmed the presence of Nd–O, S
O and P–O bonding. These results indicated the presence of element phosphorus in the Nd(CF3SO3)3*3TOP complex. The binding energy O 1s in S
O for Nd(CF3SO3)3*3TOP was higher than that of in pure Nd(CF3SO3)3, while the binding energy of Nd 3d2/5 in Nd(CF3SO3)3*3TOP was lower than that of in Nd(CF3SO3)3. This result implies that the lone pair electrons of oxygen atom in TOP was successfully coordinated with the neodymium atom.24
To further investigate the Nd(CF3SO3)3*3TOP complex, FT-IR spectra have been analyzed as shown in Fig. 2. Unambiguously, the strong IR absorption peak at 636 cm−1 indicates the existence of Nd–O groups in Nd(CF3SO3)3*3TOP, which demonstrates the coordination linkage formation. The IR peak at 1283 cm−1 disappears in the Nd(CF3SO3)3*3TOP complex while it seen in the original TOP compound. This indicates that the reaction of TOP and Nd(CF3SO3)3 was complete. Furthermore, these data indicate that there are no free TOP molecules are found in the solution, and that an additional, strong absorption peak appears at 1225 cm−1 reflecting the existence of a coordination interaction between P
O and the metal.25
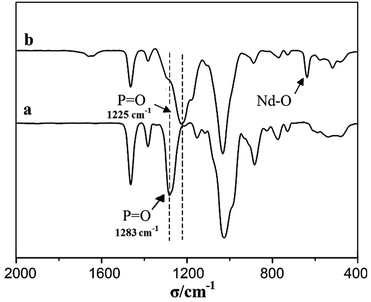 |
| Fig. 2 FI-TR spectra of complex Nd(CF3SO3)3*3TOP and ligand TOP. (a). TOP. (b). Nd(CF3SO3)3*3TOP. | |
To discuss the impact of the solubility of the catalyst on the polymer molecular distribution, we compared the result using Nd(CF3SO3)3*3TBP and Nd(CF3SO3)3*3TOP. The related XPS characterization is shown in Fig. 1(b) and Table 1. In Nd(CF3SO3)3*3TBP we can observe the appearance of a characteristic peak in the P 2p spectrum at 133.7 eV binding energy, together with the evident change in the Nd 3d2/5 and S 2p (S
O) indicating the formation of coordination linkage between Nd(CF3SO3)3 and TBP.24
31P NMR spectroscopy was used to further detect the structure of the Nd(CF3SO3)3*3TOP. The chemical shift of P in TBP occurs at −0.64 ppm, and it completely disappeared associated with the appearance at 1.21 ppm (Fig. 3), suggesting that all phosphorus was converted into the newly generated Nd(CF3SO3)3*3TOP. The change of the chemical shift was attributed to the coordination of ligand TOP to Nd(CF3SO3)3.
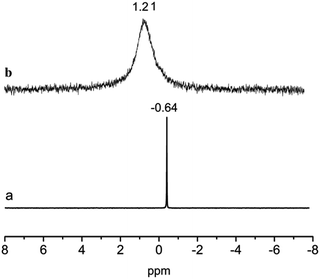 |
| Fig. 3 31P NMR spectra of complex Nd(CF3SO3)3*3TOP and ligand TOP. (a) TOP. (b) Nd(CF3SO3)3*3TOP. | |
The FT-IR spectra of Nd(CF3SO3)3*3TBP complex are shown in Fig. 4, the P
O characteristic peak in TBP results at about 1279 cm−1, however the strong absorption observed at 1223 cm−1 in Nd(CF3SO3)3*3TBP, could soundly confirm a coordination linkage structure in Nd(CF3SO3)3*3TBP.
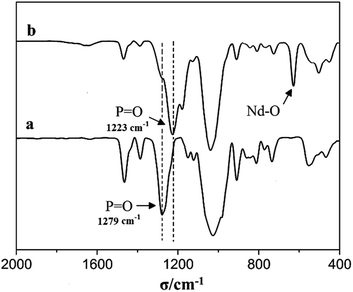 |
| Fig. 4 FI-TR spectra of complex Nd(CF3SO3)3*3TBP and ligand TBP. (a) TBP. (b) Nd(CF3SO3)3*3TBP. | |
3.2 Initiation of the polymerization of Bd via two different catalysts
Before conducting the polymerization, Nd(CF3SO3)3*3TOP should be firstly aged with a small amount of butadiene and Al(i-Bu)3 in hexane, to obtain a homogeneous binary catalyst. To make a comparison, Nd(CF3SO3)3*3TBP with Al(i-Bu)3 was also used for the polymerization of Bd. The cis-1,4 unit content and the molecular weight distribution data of PB obtained with two catalysts are presented in Table 2. The polymerization didn't occur in the absence of TOP or TBP coligands, no polymer was obtained after 24 hours (run 1). In contrast, the two catalysts show a high activity at both 20 °C and 50 °C reflecting that in a short time and at relatively low temperature both catalysts could easily initiate the Bd polymerization. However, Nd(CF3SO3)3*3TOP/Al(i-Bu)3 catalyst system demonstrated a higher activity as under the same polymerization conditions, not only the product yield was higher, but also the molecular weight distribution was narrower as can be seen from Table 2.
Table 2 Comparison of PB molecular parameters prepared via Nd(CF3SO3)3*3TOP and Nd(CF3SO3)3*3TBP systems a
Run |
Binary catalyst |
Temperature (°C) |
Yield (%) |
Mn × 104e (g mol−1) |
Mw/Mne |
cis-1,4f (%) |
trans-1,4f (%) |
1,2f (%) |
Polymerization conditions: solvent is hexane, [Nd] = 8.9 × 10−4 mol L−1, [Al]/[Nd] = 20, [Bd]/[Nd] = 2000. Polymerization time is 24 h. Polymerization time is 2 h. Polymerization time is 30 min. Measured by SEC-MALLS. Measured by FT-IR spectroscopy. |
1b |
Nd(CF3SO3)3 |
50 |
— |
— |
— |
— |
— |
— |
2c |
Nd(CF3SO3)3*3TOP |
20 |
88.2 |
9.8 |
1.32 |
98.8 |
0.5 |
0.7 |
3d |
Nd(CF3SO3)3*3TOP |
50 |
90.3 |
10.3 |
1.46 |
98.7 |
0.6 |
0.7 |
4c |
Nd(CF3SO3)3*3TBP |
20 |
83.5 |
8.7 |
3.21 |
98.6 |
0.5 |
0.9 |
5d |
Nd(CF3SO3)3*3TBP |
50 |
86.9 |
9.0 |
3.45 |
98.6 |
0.6 |
0.8 |
To further investigate the difference of the polymer structures prepared by the two catalysts, 1H NMR spectra are presented in Fig. 5, showing that for both PB, we could observe a strong chemical shifts at 5.45 ppm indicating the presence of a high cis-1,4 unit content. Meanwhile, FT-IR spectra in Fig. 6 also show a strong absorption peak at 738 cm−1. According to the calculations of the data in IR spectra, the results demonstrated that trans-1,4 and 1,2-unit contents in both PB polymers are very low (Table 2).
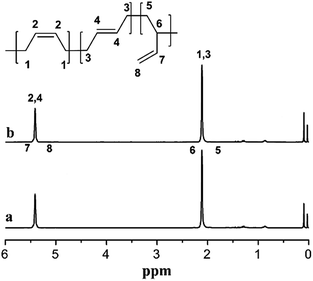 |
| Fig. 5 1H NMR spectra of PBs obtained via Nd(CF3SO3)3*3TOP and Nd(CF3SO3)3*3TBP systems. (a). Nd(CF3SO3)3*3TBP. (b). Nd(CF3SO3)3*3TOP. Polymerization conditions: in cyclohexane at 20 °C for 2 h, [Nd] = 8.9 × 10−4 mol L−1, [Al]/[Nd] = 20, [Bd]/[Nd] = 2000. | |
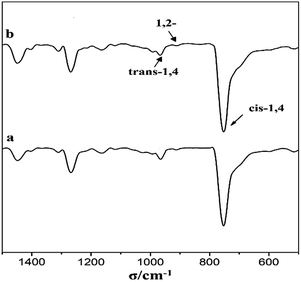 |
| Fig. 6 FT-IR spectra of PB obtained via Nd(CF3SO3)3*3TOP and Nd(CF3SO3)3*3TBP systems. (a). Nd(CF3SO3)3*3TBP. (b). Nd(CF3SO3)3*3TOP. Polymerization conditions: in cyclohexane at 20 °C for 2 h, [Nd] = 8.9 × 10−4 mol L−1, [Al]/[Nd] = 20, [Bd]/[Nd] = 2000. | |
As showed in Fig. 7, the SEC-MALLS curves indicated that both PBs exhibited a mono-modal molecular weight distribution; however, the distribution of PB using Nd(CF3SO3)3*3TOP system was much narrower than Nd(CF3SO3)3*3TBP system.
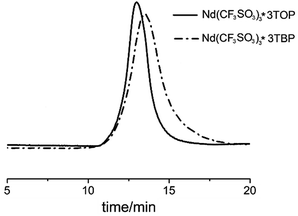 |
| Fig. 7 SEC-MALLS curves of PBs obtained via Nd(CF3SO3)3*3TOP and Nd(CF3SO3)3*3TBP systems. Polymerization conditions: in cyclohexane at 20 °C for 2 h, [Nd] = 8.9 × 10−4 mol L−1, [Al]/[Nd] = 20, [Bd]/[Nd] = 2000. | |
3.3.1 Initiation mechanism for two binary catalyst systems. As showed in Table 2, polybutadienes synthesized via both catalyst systems display a high cis-1,4 unit content. This is due to neodymium-based catalyst, which increased the coordination effect and the stereo-selectivity.26,27 At the same time, Nd(CF3SO3)3 groups have marked electron-withdrawing ability leading to the increase of the coordination and orientation effects of neodymium atom during the polymerization. Therefore, the two catalyst systems are not only efficient for initiating the polymerization of butadiene, but also allow achieving a high cis-1,4 unit content (>98%) in PB. Referring to the literatures,28–30 the detailed steps of monomer addition were described in Scheme 2.
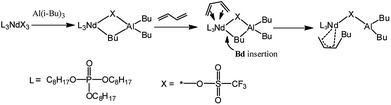 |
| Scheme 2 The monomer addition mechanism via catalyst active center of Nd(CF3SO3)3/Al(i-Bu)3 binary system. | |
Because the Nd(CF3SO3)3 has the paramagnetic property, 1H NMR was used to study the alkylation of complexes by substituting the Nd(CF3SO3)3 with La(CF3SO3)3, as shown in Fig. 8. These two characteristic peaks appeared correspondingly at δ 1.40 ppm (a, in Fig. 8) and δ 1.79 ppm (b, in Fig. 8) after alkylation of La(CF3SO3)3*3TOP, which also proved the completion of the alkylation.31
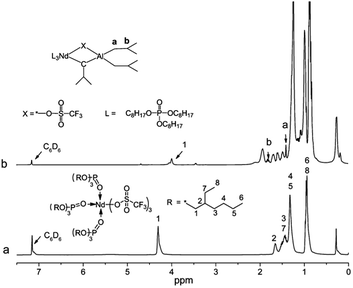 |
| Fig. 8 1H NMR of the alkylation process of La(CF3SO3)3*3TOP. (a) La(CF3SO3)3*3TOP. (b) La(CF3SO3)3*3TOP with Al(i-Bu)3. | |
It was also found from Table 2 that the molecular weight distribution of PB via Nd(CF3SO3)3*3TOP was significantly narrower than that via Nd(CF3SO3)3*3TBP. In order to understand the possible reason for this result, two system's external appearances was checked before conducting polymerization as showed in Fig. 9.
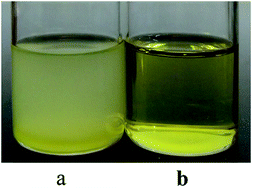 |
| Fig. 9 Solubility comparison of two catalyst systems with addition of monomer. (a) The state of the Nd(CF3SO3)3*3TBP/Al(i-Bu)3 catalyst system. (b) The state of the Nd(CF3SO3)3*3TOP/Al(i-Bu)3 catalyst system. | |
Obviously, the Nd(CF3SO3)3*3TOP system result in a clear and homogeneous state after adding the monomer solution, while the Nd(CF3SO3)3*3TBP solution was turbid and in a micro-phase separated state. This result is attributed to the fact that TOP ligand has relatively longer alkyl segments with eight carbon atoms, while TBP has only four carbon atoms. Based on the principle of similarity and intermiscibility, the Nd(CF3SO3)3*3TOP catalyst should display a better solubility in hexane. Moreover, after the addition of monomer solution to this binary catalyst system, monomer and the solvent could be in a completely homogeneous state. The polymerization could proceed from each single initiating active center to conduct the chain propagation and termination.32 Consequently, the PB with narrower molecular weight distribution could be successfully achieved. On the contrary, since Nd(CF3SO3)3*3TBP has a poor solubility in hexane, the catalyst active center are in a heterogeneous state. Although these active centers of the particle surface could still initiate the polymerization of butadiene, the heterogeneous state might limit the monomers diffusion to the active center.33 As a result, the monomer's initiation and the chain propagation are in a much heterogeneous state which could cause a wider molecular weight distribution.
4. Conclusions
A neodymium-based binary catalyst system, Nd(CF3SO3)3*3TOP/Al(i-Bu)3, was designed and synthesized by new process. With the utilization of XPS and FTIR analyses, the catalyst's composition and its coordination structure could be precisely determined. This binary catalyst system was applied to initiate the polymerization of butadiene which confirmed its efficient catalytic activity. The resulting PB product displays not only a high cis-1,4 unit content (98.8%) but also a remarkably narrow molecular weight distribution (Mw/Mn = 1.32). The high cis-1,4 unit content is attributed to the neodymium-based complex providing for a strong coordination effect and stereo-selectivity. The narrow molecular weight distribution is attributed to the longer alkyl segments in TOP, these segments could be easily dissolved in hexane and created a better homogeneous polymerization environment. These properties can lead to the monomer's initiation and chain propagation at a single molecular stage. Compared with other catalyst systems reported in the current literature, Nd(CF3SO3)3*3TOP appears as appropriate for future application thanks to its advanced initiation effect and simple preparation method.
Conflicts of interest
There are no conflicts to declare.
Acknowledgements
This work was supported by the scientific research project of Ministry of Industry and Information Technology of P. R. China (grant number JSJL2016140B004) and the Key Laboratory Project of Department of Science and Technology of Shaanxi Province, China (grant number 2013SZS17-Z02). The authors declare no competing financial interest.
References
- N. M. T. Pires, F. M. B. Coutinho and M. A. S. Costa, Eur. Polym. J., 2004, 40, 2599 CrossRef.
- N. Oskar, Neodymium-Based Ziegler-Natta Catalysts and their Application in Diene Polymerization, Springer, Berlin, 2006, p. 5 Search PubMed.
- W. Gao and D. M. Cui, J. Am. Chem. Soc., 2008, 130, 4984 CrossRef PubMed.
- Z. C. Zhang, D. M. Cui, B. L. Wang, B. Liu and Y. Yang, Polymerization of 1,3-Conjugated Dienes with Rare-Earth Metal Precursors, Springer-Verlag, Berlin Heidelberg, 2010 Search PubMed.
- G. H. Kwag, P. S. Kim, S. Han and H. K. Choi, Polymer, 2005, 46, 3782 CrossRef.
- X. F. Liu, T. Zhou, Y. C. Liu, A. M. Zhang, C. Y. Yuan and W. D. Zhang, RSC Adv., 2015, 5, 10231 RSC.
- L. X. Zhang, T. Suzuki, Y. Luo, M. Nishiura and Z. M. Hou, Angew. Chem., Int. Ed., 2007, 119, 1941 CrossRef.
- Y. M. Hu, C. L. Kong, Y. Li, L. Chang, D. D. Xu and Y. R. Wang, Polym. Mater. Sci. Eng., 2011, 27, 9 Search PubMed.
- H. Leicht, I. Göttker-Schnetmann and S. Mecking, Macromolecules, 2017, 50, 8464 CrossRef.
- D. M. Roitershtein, A. A. Vinogradov, A. A. Vinogradov, K. A. Lyssenko, Y. V. Nelyubina, I. V. Anan, I. E. Nifant'ev, V. A. Yakovlev and N. N. Kostitsyna, Organometallics, 2013, 32, 1272 CrossRef.
- A. Fischbach, F. Perdih, E. Herdtweck and R. Anwander, Organometallics, 2006, 25, 1626 CrossRef.
- P. Li, K. Y. Zhang, M. Nishiura and Z. M. Hou, Angew. Chem., Int. Ed., 2011, 50, 12012 CrossRef PubMed.
- C. L. Fan, C. X. Bai, H. G. Cai, Q. Q. Dai, X. Q. Zhang and F. S. Wang, J. Polym. Sci. A Polym. Chem., 2010, 48, 4768 CrossRef.
- Z. B. Jian, S. J. Tang and D. M. Cui, Chem.–Eur. J., 2010, 16, 14007 CrossRef PubMed.
- S. Kaita, Z. M. Hou and Y. Wakatsuki, Macromolecules, 1999, 32, 9078 CrossRef.
- L. F. Wang, D. M. Cui, Z. M. Hou, W. Li and Y. Li, Organometallics, 2011, 30, 760 CrossRef.
- Y. Yang, K. Lv, L. F. Wang, Y. Wang and D. M. Cui, Chem. Commun., 2010, 46, 6150 RSC.
- D. F. Li, S. H. Li, D. M. Cui and X. Q. Zhang, Organometallics, 2010, 29, 2186 CrossRef.
- L. X. Zhang, T. Suzuki, Y. Luo, N. Masayoshi and Z. M. Hou, Angew. Chem., Int. Ed., 2007, 46, 1909 CrossRef PubMed.
- Q. Q. Dai, X. Q. Zhang, Y. M. Hu, J. Y. He, C. Shi, Y. Q. Li and C. X. Bai, Macromolecules, 2017, 50, 7887 CrossRef.
- J. Q. Wen, X. Q. Zhang and Q. Q. Dai, Chin. J. Polym. Sci., 2015, 33, 475 CrossRef.
- Z. Y. Hu, Q. Q. Dai, H. Y. Liu, G. Z. Zhang, Y. Q. Wang, S. X. Chen, S. W. Wang and X. Q. Zhang, China Synth. Rubber Ind., 2014, 37, 96 Search PubMed.
- W. K. E. Schmalz, Rubber Chem. Technol., 1960, 33, 639 CrossRef.
- L. H. Wang and W. G. Zhang, Acta Polym. Sin., 1997, 2, 25 Search PubMed.
- Y. M. Hu, C. Q. Zhang, X. G. Liu, K. K. Gao, Y. M. Cao, C. Y. Zhang and X. Q. Zhang, J. Appl. Polym. Sci., 2014, 131, 40153 CrossRef.
- C. J. Shan, T. Sun, S. F. Feng, X. Z. Ji and S. H. Yang, Acta Polym. Sin., 1989, 06, 709 Search PubMed.
- C. Costabile, G. Milano, L. Cavallo, P. Longo, G. Guerra and A. Zambelli, Polymer, 2004, 45, 467 CrossRef.
- F. M. B. Coutinho, T. C. J. Rocha, I. L. Mello, D. S. S. Nunes, B. G. Soares and M. A. S. Costa, J. Appl. J. Appl. Polym. Sci., 2005, 98, 2539 CrossRef.
- Y. T. Jin, X. T. Zhang, F. K. P. Min and Y. Wu, J. Chin. Rare Earth Soc., 1990, 8, 79 Search PubMed.
- F. Wang, C. Y. Zhang, Y. M. Hu, X. Y. Jia, C. X. Bai and X. Q. Zhang, Polymer, 2012, 53, 6027 CrossRef.
- K. Lv and D. M. Cui, Organometallics, 2010, 29, 2987 CrossRef.
- C. Y. Ren, G. L. Li, W. M. Dong, L. S. Jiang, X. Q. Zhang and F. S. Wang, Polymer, 2007, 48, 2470 CrossRef.
- D. J. Wilson and D. K. Jenkins, Polym. Bull., 1992, 27, 407 CrossRef.
|
This journal is © The Royal Society of Chemistry 2018 |
Click here to see how this site uses Cookies. View our privacy policy here.