DOI:
10.1039/C8RA02291G
(Paper)
RSC Adv., 2018,
8, 18030-18037
Retracted Article: Overexpression of PCDH8 inhibits proliferation and invasion, and induces apoptosis in papillary thyroid cancer cells
Received
15th March 2018
, Accepted 4th May 2018
First published on 16th May 2018
Abstract
Protocadherin8 (PCDH8), a member of the protocadherin (PCDH) family, is involved in the progression of several types of cancers. However, the expression and biological roles of PCDH8 in papillary thyroid carcinoma (PTC) remain largely unknown. Therefore, in the present study, we detected the expression of PCDH8 in human PTC tissues and cell lines, and evaluated its role in PTC cells. Our results demonstrated that PCDH8 was lowly expressed in human PTC tissues and cell lines. In addition, ectopic expression of PCDH8 efficiently inhibited the proliferation, migration and invasion of PTC cells, as well as prevented the epithelial-mesenchymal transition (EMT) phenotype in PTC cells. Furthermore, PCDH8 efficiently induced apoptosis and autophagy in PTC cells. Mechanistically, overexpression of PCDH8 significantly prevented the activation of the Wnt/β-catenin pathway in PTC cells. Taken together, these findings showed that overexpression of PCDH8 inhibits proliferation and migration/invasion, and induces apoptosis of PTC cells through the Wnt/β-catenin signaling pathway.
Introduction
Thyroid cancer is the most common endocrine carcinoma. The incidence of thyroid cancer has been increasing annually. Papillary thyroid carcinoma (PTC) is the most common histological type, accounting for 85–90% of all cases.1 Although surgical approaches, adjuvant chemotherapy and radiotherapy have been improved over the past decade, the 10 year survival rate is poor in PTC patients with recurrence or metastasis.2–4 Therefore, there is an urgent need to explore novel biological markers for early diagnosis and develop new strategies for the treatment of PTC.
Protocadherins (PCDHs) are a group of transmembrane proteins that belong to the cadherin superfamily.5 They are expressed predominantly in the nervous system.6 Previous studies have shown that PCDHs play important roles in regulating multiple biological processes, such as cell proliferation, adhesion, cell cycle arrest, migration, apoptosis, dendrite arborization and neural development.7–9 Protocadherin8 (PCDH8), a member of the protocadherins family, is frequently dysregulated in several types of cancers and plays a critical role in tumor progression.10–12 Most recently, one study showed that the expression levels of PCDH8 were significantly decreased in human glioma tissues, and restoration of PCDH8 markedly inhibited glioma cell proliferation in vitro.13 However, the biological roles of PCDH8 in PTC and the related mechanisms remain unclear. Therefore, in the present study, we detected the expression of PCDH8 in human PTC tissues and cell lines, and evaluated its role in PTC progression. Our results demonstrated that PCDH8 is lowly expressed in human PTC, and overexpression of PCDH8 inhibits the proliferation, migration and induces the apoptosis of PTC cells through the Wnt/β-catenin signaling pathway.
Materials and methods
Tissue specimens
Fresh PTC tissues and their paired normal tissues were collected from ten patients undergoing surgery in our hospital. The specimens were preserved in liquid nitrogen and stored at −80 °C until use. Written informed consent was obtained from each participant, and the specimen collection procedure was approved by the Medical Ethics Committee of Huaihe Hospital, Henan University (China).
Cell culture
Human PTC cell lines (TPC-1, BCPAP and KTC-1) and the normal thyroid epithelial cell-derived cell line (HTori-3) were purchased from the American Type Culture Collection (ATCC, Manassas, VA, USA) and cultured in Dulbecco's modified Eagle's medium (DMEM; Invitrogen, Carlsbad, CA, USA), supplemented with 10% fetal bovine serum (FBS; Gibco, Rockville, MD) and 1% penicillin/streptomycin (Sigma, St. Louis, MO, USA). All cells were maintained at 37 °C with 5% CO2 in a humidified incubator.
Construction of PCDH8 overexpressing cell lines
The full-length PCDH8 open reading frame was amplified from human PTC cells by RT-PCR, and cloned into the pcDNA3.1 vector. Twenty-four hours prior to transfection, cells were plated onto a 96-well plate for 24 h. Then, the recombinant plasmid pcDNA3.1-PCDH8 and pcDNA3.1 (empty vector) were transferred into PTC cells using Lipofectamine™2000 (Invitrogen) according to the manufacturer's protocols.
RNA extraction and quantitative reverse transcription polymerase chain reaction (RT-qPCR)
Total RNA was isolated from PTC tissues or cells using the Trizol reagent (Invitrogen). Four microgram of total RNA was reverse-transcribed to complementary DNA (cDNA) using the Transcriptor First Strand cDNA Synthesis Kit (Invitrogen). PCR amplification was carried out by ABI PRISM 7900 thermocycler using SYBR Green Supermix (Invitrogen). The specific primers for PCDH8 were forward, 5′-GCCCAACATGTTCGACGTGC-3′; and reverse, 5′-GGAGTGTCCTTTCCACACCG-3′; and for β-actin were forward, 5′-AAATCGTGCGTGACATCAAAGA-3′ and reverse, 5′-GGCCATCTCCTGCTCGAA-3′. The relative quantification of gene expression level was calculated using the 2−ΔΔCt method.14
Western blot
Total protein was extracted from PTC tissues or cells using RIPA lysis buffer (Beyotime, Nantong, China). Protein concentrations were measured using Bio-Rad protein assay reagent (Bio-Rad Laboratories, Berkeley, CA, USA). Equivalent amounts of protein (30 μg) were separated by 10% SDS-PAGE and transferred to PVDF membrane (Millipore, Boston, MA, USA). The membranes were treated using the following procedure with blocking with 5% non-fat dry milk for 1 h followed by incubation in the primary antibodies at 4 °C overnight. The following antibodies were used: anti-PCDH8, anti-E-cadherin, anti-N-cadherin, anti-vimentin, anti-Bcl-2, anti-Bax, anti-LC3BI, anti-LC3BII, anti-p62, anti-β-catenin, anti-cyclin D1, anti-c-Myc and anti-GAPDH. All primary antibodies were purchased from Santa Cruz Biotechnology, Inc. (Santa Cruz, CA, USA). Subsequently, the membrane was washed and incubated with HRP-conjugated secondary antibody for 1 h at room temperature. Finally, immuno-complexes were visualized using enhanced chemiluminescence reagents (Pierce, Rockford, IL, USA).
Cell proliferation assay
Cell proliferation was evaluated using the 3-(4,5-dimethylthiazol-2-yl)-2,5-diphenyltetrazolium bromide (MTT) assay. In brief, PTC cells (1 × 104 cells per well) were seeded into 96-well culture plates and cultured for 1–4 days after transfection. At each time point, 100 μl of MTT solution (5 mg ml−1; Sigma) was added to each well, followed by incubation at 37 °C for 4 h. Then, 150 μl of dimethylsulfoxide (DMSO; Sigma) was added to each well to lyse the cells. The absorbance was measured at 490 nm using an ELX-800 spectrometer reader (Bio-Tek Instruments, Winooski, USA).
Cell migration and invasion assays
Cell migration and invasion assays were performed by the Transwell chamber with 8 μm pore size polycarbonate membrane (Millipore). For migration assay, the transfected TPC-1 and BCPAP cells (5 × 104 cells per well) suspended in 100 μl serum-free medium were seeded in the upper compartment, while the lower chamber was filled with 500 μl of DMEM containing 10% FBS. After 24 h, the migrated cells on the lower surface of the filter were fixed, stained and counted under a light microscope (Olympus Corp., Tokyo, Japan). Cell invasion assay was done by the same procedure, except that the chamber was pre-coated with Matrigel (BD Biosciences) to form a matrix barrier.
Flow cytometry
The transfected TPC-1 and BCPAP cells were washed twice with cold phosphate-buffered saline (PBS) and then re-suspended in 500 μl 1× binding buffer, followed by 20 μg ml−1 Annexin V-FITC (KeyGENE Biotech) staining and 20 μg ml−1 propidium iodide (PI; Sigma) incubation for 20 min at the room temperature in dark. Finally, the cells were immediately analyzed by a flow cytometry (BD Bioscience, CA, USA).
Caspase-3 activity assay
After transfection, the cell culture medium was centrifuged, and the supernatant was collected. Caspase-3 activity in the supernatant was determined using a caspase 3 Activity Assay Kit (Beyotime) following the manufacturer's protocols.
Statistical analysis
All statistical analyses were performed using SPSS software (ver. 13.0; SPSS, Chicago, IL, USA). Results are expressed as means ± SD. Differences between two groups were analyzed by Student's t test. Differences between multiple groups were analyzed by ANOVA followed by Tukey's post hoc test. P < 0.05 was considered to be statistically significant.
Results
PCDH8 is lowly expressed in PTC tissues and cell lines
First, we examined the expression of PCDH8 in human PTC tissues using qRT-PCR and western blot analysis. As indicated in Fig. 1A, PCDH8 mRNA expression was lower in PTC tissues than that of in their paired normal tissues. Similarly, PCDH8 protein was also significantly down-regulated in PTC tissues (Fig. 1B). Additionally, the expression of PCDH8 in human PTC cell lines was also evaluated. The results indicated that the expression of PCDH8 at both mRNA and protein levels was markedly decreased in all PTC cell lines as compared with the normal thyroid epithelial cell-derived cell line (Fig. 1C and D).
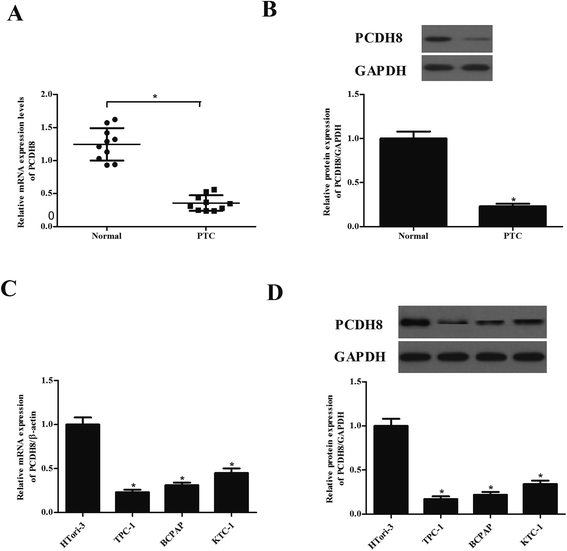 |
| Fig. 1 PCDH8 is lowly expressed in PTC tissues and cell lines. (A) PCDH8 mRNA expression levels in human PTC tissues were evaluated using qRT-PCR assay. (B) PCDH8 protein expression levels in human PTC tissues were evaluated using western blot analysis. *P < 0.05 compared with the normal group. (C) PCDH8 mRNA expression levels in human PTC cell lines were evaluated using qRT-PCR assay. (D) PCDH8 protein expression levels in human PTC cell lines were evaluated using western blot analysis. Data are presented as the mean ± S.D. of the three independent experiments. *P < 0.05 compared with the HTori-3 group. | |
Overexpression of PCDH8 inhibits the proliferation of PTC cells in vitro
To examine the functional role of PCDH8 in PTC progression, TPC-1 and BCPAP cells were transfected with pcDNA3.1-PCDH8 or empty vector, respectively. The expression of PCDH8 was dramatically increased in TPC-1 (Fig. 2A) and BCPAP cells (Fig. 2B) after transfection, respectively, suggesting that the transfection was successful. Then, the effect of PCDH8 on PTC cell proliferation was measured using the MTT assay. As shown in Fig. 2C, as compared with the vector group, overexpression of PCDH8 greatly suppressed the proliferation of TPC-1 cells. Similar results were observed in BCPAP cells (Fig. 2D).
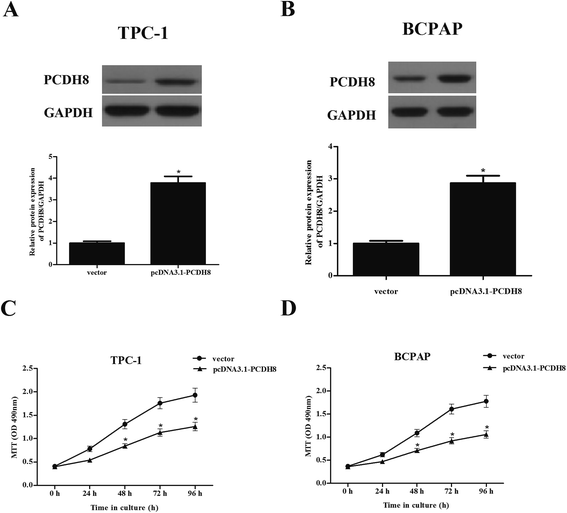 |
| Fig. 2 Overexpression of PCDH8 inhibits the proliferation of PTC cells in vitro. TPC-1 and BCPAP cells were transfected with pcDNA3.1-PCDH8 or empty vector for 24 h, respectively. (A and B) The transfection efficiency was detected in TPC-1 and BCPAP cells. (C and D) Cell proliferation was measured using the MTT assay in TPC-1 and BCPAP cells. Data are presented as the mean ± S.D. of the three independent experiments. *P < 0.05 compared with the vector group. | |
Overexpression of PCDH8 inhibits the EMT phenotype in PTC cells
Next, we investigated the potential impacts of PCDH8 on PTC cell migration and invasion. The results of Transwell migration assay indicated that the number of migrated cell was significantly reduced in TPC-1 cells transfected with pcDNA3.1-PCDH8, which was comparable to the vector group (Fig. 3A). Matrigel invasion assay indicated that overexpression of PCDH8 sharply inhibited the invasion of TPC-1 cells (Fig. 3B). Additionally, we found that overexpression of PCDH8 greatly inhibited the migration and invasion of BCPAP cells (Fig. 3C and D). Then, we examined the effect of PCDH8 on the expression of EMT-related markers in PTC cells. As indicated in Fig. 3E, as compared with vector group, the expression of E-cadherin was markedly increased in TPC-1 cells transfected with pcDNA3.1-PCDH8; whereas, the expression of N-cadherin and vimentin was significantly reduced. Furthermore, overexpression of PCDH8 dramatically up-regulated E-cadherin expression and down-regulated the expression of N-cadherin and vimentin in BCPAP cells (Fig. 3F).
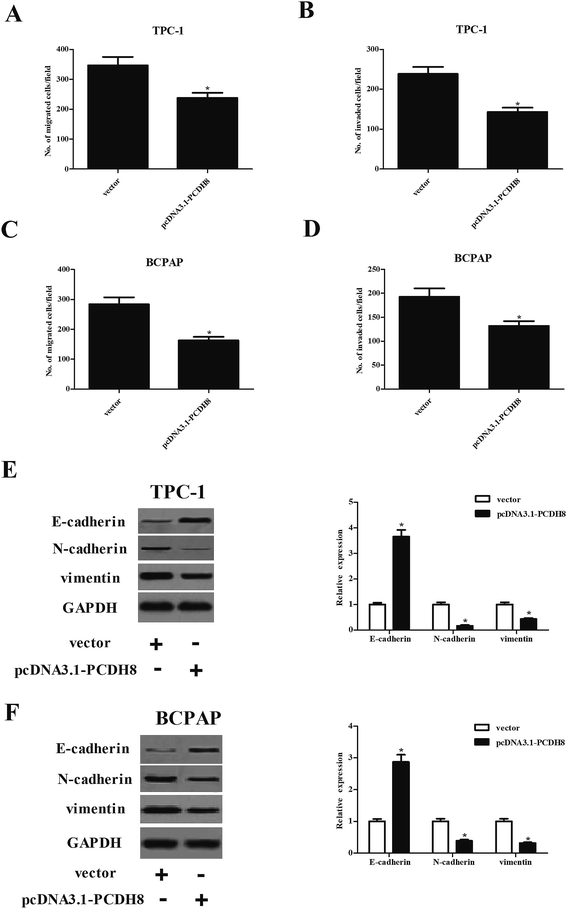 |
| Fig. 3 Overexpression of PCDH8 inhibits the EMT phenotype in PTC cells. TPC-1 and BCPAP cells were transfected with pcDNA3.1-PCDH8 or empty vector for 24 h, respectively. (A and C) Cell migration was detected by Transwell migration assay in TPC-1 and BCPAP cells. (B and D) Cell invasion was detected by Matrigel invasion assay in TPC-1 and BCPAP cells. (E and F) The expression of E-cadherin, N-cadherin and vimentin in TPC-1 and BCPAP cells. Data are presented as the mean ± S.D. of the three independent experiments. *P < 0.05 compared with the vector group. | |
Overexpression of PCDH8 promotes the apoptosis and autophagy of PTC cells
Flow cytometry analysis demonstrated that overexpression of PCDH8 dramatically resulted in an increasing of apoptotic rate in TPC-1 and BCPAP cells in comparison to the control groups (Fig. 4A–C). In addition, PCDH8 markedly increased Bax expression and suppressed Bcl-2 expression, as well as induced caspase-3 activity in both TPC-1 (Fig. 4D and E) and BCPAP cells (Fig. 4D and F). Furthermore, the results of western blot analysis indicated that overexpression of PCDH8 significantly up-regulated the expression of LC3BI and LC3BII, as well as down-regulated p62 expression in TPC-1 cells, as compared with the vector group (Fig. 4G). Similar results were observed in BCPAP cells (Fig. 4H).
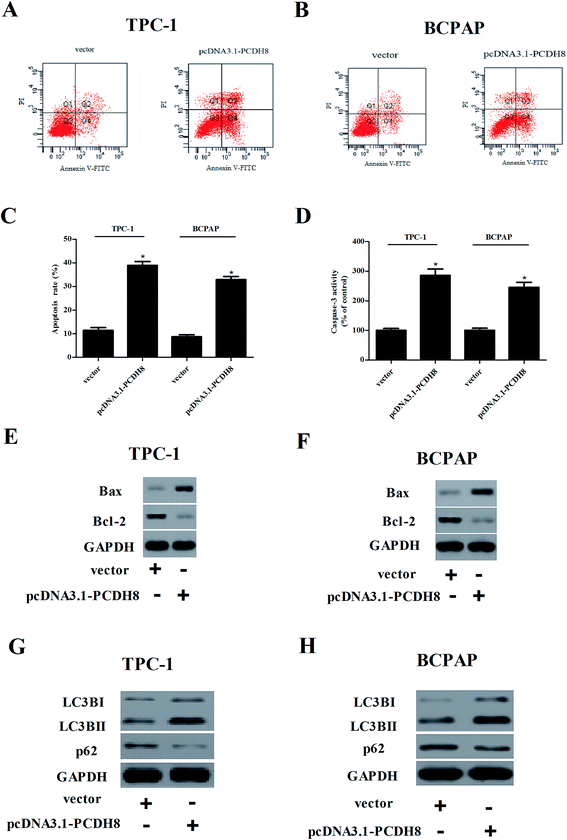 |
| Fig. 4 Overexpression of PCDH8 promotes the apoptosis and autophagy of PTC cells. TPC-1 and BCPAP cells were transfected with pcDNA3.1-PCDH8 or empty vector for 24 h, respectively. (A and B) Cell apoptosis was detected by flow cytometric analysis in TPC-1 and BCPAP cells. (C) Quantification of cell apoptosis in TPC-1 and BCPAP cells. (D) The activity of caspase-3 was detected by caspase 3 Activity Assay Kit in TPC-1 and BCPAP cells. (E and F) The expression of Bax and Bcl-2 was detected by western blot in TPC-1 and BCPAP cells. (G and H) The expression of LC3BI, LC3BII and p62 in TPC-1 and BCPAP cells. Data are presented as the mean ± S.D. of the three independent experiments. *P < 0.05 compared with the vector group. | |
Overexpression of PCDH8 inhibits the activation of Wnt/β-catenin pathway in PTC cells
In order to explore the molecular mechanism by which PCDH8 regulates PTC progression, we investigated the effect of PCDH8 on the activation of Wnt/β-catenin pathway in TPC-1 cells. The results of western blot analysis demonstrated that ectopic expression of PCDH8 efficiently downregulated the expression of β-catenin, cyclin D1 and c-Myc in TPC-1 cells, as compared with the vector group (Fig. 5A). We observed that the activation of Wnt signaling by LiCl treatment impaired the inhibitory effects of PCDH8 on the proliferation (Fig. 5C), metastasis (Fig. 5D) and apoptosis (Fig. 5E) of TPC-1 cells.
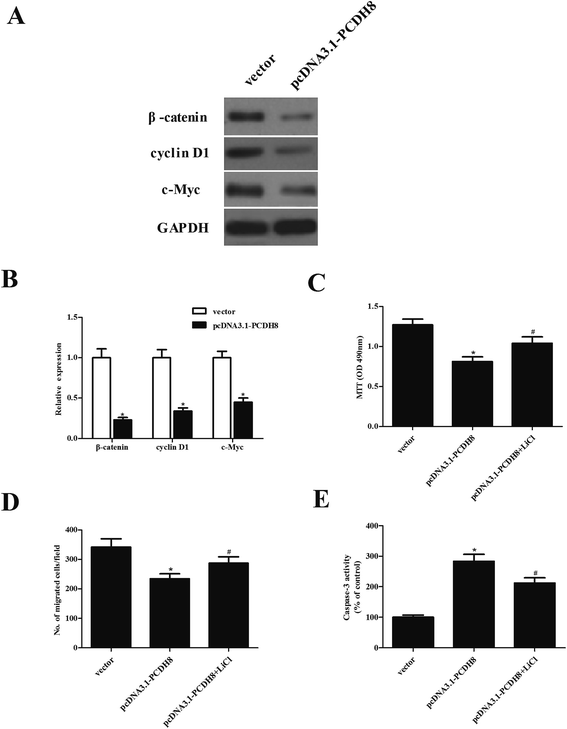 |
| Fig. 5 Overexpression of PCDH8 inhibits the activation of Wnt/β-catenin pathway in PTC cells. TPC-1 cells were transfected with pcDNA3.1-PCDH8 or empty vector for 24 h, respectively. (A) The expression of β-catenin, cyclin D1 and c-Myc was measured using western blot analysis. (B) Quantification of β-catenin, cyclin D1 and c-Myc. TPC-1 cells were transfected with pcDNA3.1-PCDH8 or empty vector in the presence or absence of the Wnt pathway activator LiCl (20 mmol L−1) for 24 h. (C) Cell proliferation was determined by the MTT assay. (D) Cell migration was evaluated by the Transwell migration assay. (E) The activity of caspase-3 was detected by caspase 3 Activity Assay Kit. Data are presented as the mean ± S.D. of the three independent experiments. *P < 0.05 compared with the vector group; #P < 0.05 compared with the pcDNA3.1-PCDH8 group. | |
Discussion
In this study, we showed that PCDH8 was lowly expressed in human PTC tissues and cell lines. Overexpression of PCDH8 significantly suppressed the proliferation, migration/invasion and the EMT process in PTC cells. Furthermore, PCDH8 efficiently induced the apoptosis and autophagy of PTC cells. Mechanistically, overexpression of PCDH8 significantly prevented the activation of Wnt/β-catenin pathway in PTC cells.
Several studies have demonstrated that PCDH8 is frequently dysregulated in several types of cancers.10,11,15 It was reported that the expression of PCDH8 was significantly reduced in liver cancer specimens.11 Another study showed that PCDH8 is frequently silenced by aberrant promoter methylation in clear cell renal cell carcinoma.16 In line with these results, in the present study, we observed that PCDH8 was lowly expressed in human PTC tissues and cell lines, suggesting that PCDH8 may act as a tumor suppressor in PTC.
Metastasis remains the most common cause of lethal outcomes in PTC after curative resection. Tumor metastasis is the result of cancer cell migration and invasion. The EMT is a crucial program for the invasion and metastasis of tumors. During EMT procedure, the actin cytoskeleton is reorganized and cells acquire increased cell-matrix contacts, leading to dissociation from surrounding cells and enhanced migration and invasion.17 Down-regulation of E-cadherin expression is usually associated with advanced stage and progression.18 In this study, we observed that overexpression of PCDH8 dramatically suppressed PTC cell migration/invasion in vitro, as well as prevented the EMT phenotype in PTC cells. These data imply that PCDH8 can prevent EMT phenotype, resulting in the inhibition of PTC cell migration and invasion.
Apoptosis is also the key biological feature of PTC progression.19 Caspase-3 is a frequently activated death protease, catalyzing the specific cleavage of various key cellular proteins.20 In addition, a study by Zhang et al. reported that ectopic expression of PCDH8 markedly promoted apoptosis in gastric cancer cells.21 In agreement with previous studies, we observed that PCDH8 induces apoptosis in PTC cells through caspase-3 signaling via increasing the ratio of Bax/Bcl-2. Furthermore, mounting evidence indicates that autophagy is correlated with the process of cancer cell apoptosis.22–24 The role of autophagy in cancer is controversially discussed, where it can act both as a tumor promoter or a stimulator of apoptotic cell death.25 Herein, we found that PCDH8 efficiently induced expression of LC3BI and LC3BII, as well as down-regulated p62 expression in PTC cells.26 These data imply that PCDH8 promoted cell apoptosis in PTC cells by inducing autophagy signaling.
The Wnt/β-catenin signaling pathway plays a critical role in regulating proliferation, survival, metastasis and apoptosis of cancer cells.27 Previous studies indicate that the Wnt/β-catenin pathway is frequently activated in a variety of human cancers, including PTC, and promotes tumor progression.28–31 β-catenin is a main downstream effector of the canonical Wnt signaling pathway and induces the transcription of several target genes, including cyclin D1 and c-Myc, which are implicated in cell proliferation, metastasis, EMT and apoptosis.32 Therefore, blocking Wnt/β-catenin signaling pathway could suppress metastatic potential and tumorigenesis in PTC.33,34 Most importantly, a study has recently shown that PCDH8 significantly decreased the level of β-catenin in glioma cells.35 Similarly, in this study, we found that ectopic expression of PCDH8 efficiently downregulated the expression of β-catenin, cyclin D1 and c-Myc in PTC cells, and LiCl treatment impaired the inhibitory effects of PCDH8 on PTC cells. These results suggest that PCDH8 suppresses the proliferation and metastasis and promotes the apoptosis and autophagy of PTC cells at least in part through the negative regulation of the Wnt/β-catenin signaling pathway.
Conclusion
In conclusion, our findings showed that PCDH8 was lowly expressed in human PTC tissues and cell lines, and overexpression of PCDH8 inhibits the proliferation, migration/invasion and as well as induces the apoptosis and autophagy in PTC cells through the Wnt/β-catenin signaling pathway.
Conflicts of interest
The authors have no competing interests to disclose.
Acknowledgements
None.
References
- Y. Mao and M. Xing, Endocr.-Relat. Cancer, 2016, 23, 313–322 CrossRef PubMed.
- E. Scott, D. Learoyd and R. J. Clifton-Bligh, Future Oncol., 2016, 12, 2603–2613 CrossRef PubMed.
- J. Zidan, D. Karen, M. Stein, E. Rosenblatt, W. Basher and A. Kuten, Cancer, 2003, 98, 1181–1185 CrossRef PubMed.
- P. Suman, C. H. Wang, S. S. Abadin, T. A. Moo-Young, R. A. Prinz and D. J. Winchester, Surgery, 2016, 160, 1717 CrossRef PubMed.
- S. Y. Kim, S. Yasuda, H. Tanaka, K. Yamagata and H. Kim, Cell Adhes. Migr., 2011, 5, 97–105 CrossRef.
- K. Sano, H. Tanihara, R. L. Heimark, S. Obata, M. Davidson, T. St John, S. Taketani and S. Suzuki, EMBO J., 1993, 12, 2249–2256 Search PubMed.
- Z. Li, J. Xie, W. Li, A. Tang, X. Li, Z. Jiang, Y. Han, J. Ye, J. Jing, Y. Gui and Z. Cai, Gene, 2011, 475, 49–56 CrossRef PubMed.
- Y. Yang, J. Liu, X. Li and J. C. Li, Histol. Histopathol., 2012, 27, 217–224 Search PubMed.
- J. Etzrodt, K. KrishnaK and C. Redies, BMC Neurosci., 2009, 10, 153 CrossRef PubMed.
- J. S. Yu, S. Koujak, S. Nagase, C. M. Li, T. Su, X. Wang, M. Keniry, L. Memeo, A. Rojtman and M. Mansukhani, Oncogene, 2008, 27, 4657–4665 CrossRef PubMed.
- C. Zhang, Y. Peng, F. Yang, R. Qin and W. Liu, Cancer J., 2016, 7, 446–452 CrossRef PubMed.
- Y. L. Lin, Y. L. Wang, J. G. Ma and W. P. Li, J. Exp. Clin. Cancer Res., 2014, 33, 1–6 CrossRef PubMed.
- Z. Zong, H. Pang, R. Yu and Y. Jiao, Oncol. Lett., 2017, 14, 3357–3362 CrossRef PubMed.
- K. J. Livak and T. D. Schmittgen, Methods, 2001, 25, 402–408 CrossRef PubMed.
- Y. L. Lin, J. H. Ma, X. L. Luo, T. Y. Guan and Z. G. Li, J. Int. Med. Res., 2013, 41, 48–54 CrossRef PubMed.
- Y. L. Lin, Y. L. Wang, X. L. Fu and J. G. Ma, Med. Sci. Monit., 2014, 20, 2380–2385 CrossRef PubMed.
- D. C. Radisky, Cancer Res., 2008, 68, 9574–9577 CrossRef PubMed.
- M. H. Lu, M. F. Fan and X. D. Yu, Eur. Rev. Med. Pharmacol. Sci., 2017, 21, 928–936 Search PubMed.
- R.-C. Simbulan, D. Rosenthal, R. Luo and M. Smulson, Cancer Res., 1999, 59, 2190–2194 Search PubMed.
- A. G. Porter and R. U. Jänicke, Cell Death Differ., 1999, 6, 99–104 CrossRef PubMed.
- D. Zhang, W. Zhao, X. Liao, T. Bi, H. Li and X. Che, Oncol. Rep., 2012, 28, 1785–1791 CrossRef PubMed.
- J. Moscat and M. T. Diazmeco, Cell, 2009, 137, 1001–1004 CrossRef PubMed.
- L. Ouyang, Z. Shi, S. Zhao, F. T. Wang, T. T. Zhou, B. Liu and J. K. Bao, Cell Proliferation, 2012, 45, 487–498 CrossRef PubMed.
- X. L. Guo, D. Li, F. Hu, J. R. Song, S. S. Zhang, W. J. Deng, K. Sun, Q. D. Zhao, X. Q. Xie and Y. J. Song, Cancer Lett., 2012, 320, 171–179 CrossRef PubMed.
- Z. J. Yang, C. E. Chee, S. Huang and F. A. Sinicrope, Mol. Cancer Ther., 2011, 10, 1533–1541 CrossRef PubMed.
- X. Sui, R. Chen, Z. Wang, Z. Huang, N. Kong, M. Zhang, W. Han, F. Lou, J. Yang and Q. Zhang, Cell Death Dis., 2013, 4, e838 CrossRef PubMed.
- H. H. Luu, R. Zhang, R. C. Haydon, E. Rayburn, Q. Kang, W. Si, J. K. Park, H. Wang, Y. Peng and W. Jiang, Curr. Cancer Drug Targets, 2004, 4, 653–671 CrossRef PubMed.
- C. Li, X. Shi, G. Zhou, X. Liu, S. Wu and J. Zhao, Front. Biosci., 2013, 18, 1384–1391 CrossRef.
- C. H. Lin, T. Ji, C. F. Chen and B. H. Hoang, Wnt signaling in osteosarcoma, Adv. Exp. Med. Biol., 2014, 804, 33–45 CrossRef PubMed.
- R. Li and L. Wang, Biochem. Biophys. Res. Commun., 2016, 474, 730–735 CrossRef PubMed.
- R. C. Haydon, A. Deyrup, A. Ishikawa, R. Heck, W. Jiang, L. Zhou, T. Feng, D. King, H. Cheng and B. Breyer, Int. J. Cancer, 2002, 102, 338–342 CrossRef PubMed.
- H. Clevers, Cell, 2006, 127, 469–480 CrossRef PubMed.
- Y. Liu, W. Wang, J. Xu, L. Li, Q. Dong, Q. Shi, G. Zuo, L. Zhou, Y. Weng and M. Tang, Oncol. Rep., 2013, 30, 1723–1730 CrossRef PubMed.
- Y. Liu, Y. Z. Liu, R. X. Zhang, X. Wang, Z. J. Meng, J. Huang, K. Wu, J. Y. Luo, G. W. Zuo and L. Chen, Int. J. Oncol., 2014, 45, 795–803 CrossRef PubMed.
- Z. Zong, H. Pang, R. Yu and Y. Jiao, Oncol. Lett., 2017, 14, 3357 CrossRef PubMed.
Footnote |
† These authors contributed equally to this work. |
|
This journal is © The Royal Society of Chemistry 2018 |