DOI:
10.1039/C8RA02138D
(Paper)
RSC Adv., 2018,
8, 13388-13392
A simple two-photon turn-on fluorescent probe for the selective detection of cysteine based on a dual PeT/ICT mechanism†
Received
10th March 2018
, Accepted 29th March 2018
First published on 10th April 2018
Abstract
Herein, a simple two-photon turn-on fluorescent probe, N-(6-acyl-2-naphthayl)-maleimide (1), based on a dual PeT/ICT quenching mechanism is reported for the highly sensitive and selective detection of cysteine (Cys) over other biothiols. The probe was applied in the two-photon imaging of Cys in cultured HeLa cells, excited by a near-infrared laser at 690 nm.
Cysteine (Cys), homocysteine (Hcy), and glutathione (GSH) are structurally similar biothiols, but their biological functions are quite different from one another.1–6 Among these biothiols, Cys functions as one of the twenty-one amino acids for peptide and protein synthesis, and Cys deficiency is also associated with certain disease symptoms.7–10 Methods for the selective detection and differentiation of Cys among different biothiols, including high performance liquid chromatography (HPLC),11 capillary electrophoresis,12 electrochemical assay,13 UV-vis spectroscopy,14 and fluorescence-based methods,15–17 are important for its biological studies. Recently, fluorescent probes have attracted much attention as vital chemical biology tools due to their high sensitivity, convenient operation, and real-time imaging capabilities.18–20 A number of Cys-selective fluorescent probes have been reported,21 which utilize Cys-selective recognition groups such as aldehydes,11,22 acrylates,23 thioesters,24 and electron-deficient aromatic halides25–27 in their structures. However, many of them have relatively long response times and low sensitivity due to a slow cyclization process. In addition, fluorescent probes with high selectivity for Cys over Hcy are difficult to achieve because they differ by only one methylene group.28 Recently, we reported that N-(N′-butyl-1,8-naphthalimide-4-yl)-maleimide, containing a single maleimide group as the recognition group, is a fast, sensitive, and selective fluorescent probe for Cys based on a dual photo-induced electron transfer (PeT) and photo-induced intramolecular charge transfer (ICT) quenching mechanism.28 Different from many other maleimide-based fluorescent probes that only undergo a PeT mechanism,15 the additional ICT quenching mechanism keeps the 1,8-naphthalimide (NAP) fluorophore in the thiol-Michael adduct in a low fluorescence emission state due to the strong electron-withdrawing effects of the succinimide group at its 4-position. Then, a subsequent transcyclization step, involving the formation of a six-membered thiomorpholinone ring and cleavage of a five-membered succinimide ring, converts the non-fluorescent thiol-Michael adduct into the major fluorescent product, in which the ICT quenching is removed, resulting in a drastic fluorescence turn-on response.28 A similar transcyclization process and the simultaneous removal of ICT quenching allowed us to design a NAP-based turn-on fluorescent probe for γ-glutamyltranspeptidase29 and a coumarin-based turn-on fluorescent probe with dual recognition groups and dual cyclization for the selective detection of Cys.30 In addition, another NAP-based dual PeT/ICT probe was recently reported by Meka and Heagy for the detection of hydrogen sulfide, although two recognition groups instead of one were adopted in their probe to achieve the dual quenching mechanism.31
Our previous work and that of other groups has demonstrated that the combination of PeT and ICT mechanisms is particularly suitable for the design of fluorescent probes with a significant fluorescence turn-on response.30–33 However, many of these probes have a short excitation wavelength in the UV or visible range, which is not optimal for biological applications due to enhanced phototoxicity and/or autofluorescence.34,35 Considering that two-photon fluorescence imaging has advantages such as the excitation process being carried out by a near-infrared (NIR) laser that has a reduced cell toxicity and low fluorescence background,36 in this work, we aimed to introduce a similar dual PeT/ICT quenching mechanism to the known two-photon fluorophore 6-acyl-2-naphthylamine37–39 in order to design a simple maleimide-based two-photon fluorescent probe, 1, for the selective detection of Cys over Hcy and GSH. It was also tested to determine whether it is a turn-on fluorescent probe with high sensitivity and selectivity, which reacts with Cys via a fast two-step thiol-Michael addition and transcyclization cascade reaction.28 The structure of probe 1 is shown in Fig. 1. It has a maleimide group at its 2-position, which promotes the PeT quenching effect. It also has an additional electron-withdrawing methylcarbonyl group at its 6-position to ensure a pull–pull ICT quenching effect.
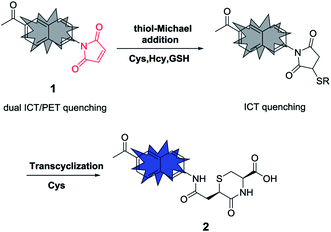 |
| Fig. 1 Design rationale of the fluorescent probe 1 for the selective turn-on detection of Cys over Hcy and GSH. | |
Probe 1 was conveniently synthesized from 6-acyl-2-naphthylamine (3)39 in a two-step process with a total yield of 38% (see Scheme S1 in the ESI†). First, the amine 3 was reacted with maleic anhydride to form the maleic amide acid 4. Then, the amide acid 4 was cyclized to afford the maleimide 1 in the presence of acetic anhydride (see the ESI† for more details).
We then investigated the absorption and fluorescence emission response of the probe towards just 1 equiv. of Cys. The time-dependent absorption spectra upon the addition of 1 equiv. of Cys are shown in Fig. 2a. Probe 1 has a maximum absorption peak at 292 nm. Upon addition of Cys, the maximum absorption peak shifts to 314 nm, a red-shift of 22 nm. Notably, an isosbestic point can be seen at 295 nm after 2 min, indicating the formation of an intermediate within 2 min, which is then converted into the final product. The UV spectral changes supported the presence of a proposed cascade reaction sequence for the fast formation of a thiol-Michael adduct intermediate, which then underwent a relatively slow intramolecular transcyclization process to give the final product. From time-dependent fluorescence emission studies (Fig. 2b), probe 1 was found to have almost no fluorescence emission due to dual PeT and ICT quenching effects. Upon the addition of 1 equiv. of Cys, a drastic turn-on fluorescence response (a >3000 fold increase) was observed at 446 nm (see Fig. S1b in the ESI†). The fluorescence intensity at 446 nm reached its maximum value after around 30 min indicating that the cascade reaction finished in about 30 min (Fig. 2b, and S2a in the ESI†). The pseudo-first-order reaction kinetic constant based on the fluorescence enhancement was calculated as 0.123 min−1 (half-time = 5.64 min, Fig. S2b in the ESI†), indicating an overall fast cascade reaction. Fluorescence titration experiments using an increasing amount of Cys from 0 to 4.0 equiv. over 30 min showed a steady increase in the fluorescence intensity and the maximum intensity was reached at exactly 1.0 equiv. of Cys. Further Cys addition did not increase the fluorescence intensity, indicating that probe 1 reacts with Cys in a 1
:
1 molar ratio (Fig. 2c and S3 in the ESI†), which was also supported by the Job plot (see Fig. S4 in the ESI†). From the linear relationship of the fluorescence intensity at 446 nm versus the Cys concentrations, the detection limit of probe 1 (2 μM) for Cys was calculated as 1.4 nM (S/N = 3, Fig. 3d), indicating that 1 is a highly sensitive probe for Cys. Moreover, the probe showed excellent selectivity for the detection of Cys over many other species (Fig. 2e, and S5 in the ESI†), including the structurally similar thiols Hcy, GSH, and N-acetylcysteine (NAC). The fluorescence intensity at 446 nm for 1 equiv. of Cys was significantly higher (12.2-fold, 9.1-fold, and 17.7-fold, respectively) than that of 10 equiv. of Hcy, GSH, or NAC. To further confirm the reaction mechanism, the reaction product, 2, from the reaction of probe 1 with Cys, was isolated and its structure was confirmed using 1H nuclear magnetic resonance (NMR) spectroscopy, 13C NMR spectroscopy, 2D-rotating-frame nuclear Overhauser effect spectroscopy (ROESY), and high-resolution mass spectrometry (HRMS) (see the ESI for more details†). The fluorescence quantum yields of probe 1 and product 2 were measured as 0.002 and 0.782, respectively (see the ESI for more details†). Therefore, the formation of the transcyclization product 2 was determined to be responsible for the observed fluorescence turn-on response. For the other thiols, the transcyclization steps of the thiol-Michael adducts were much slower, resulting in the observed high selectivity. Overall, we have shown here that probe 1 is a highly sensitive and selective turn-on fluorescent probe for Cys.
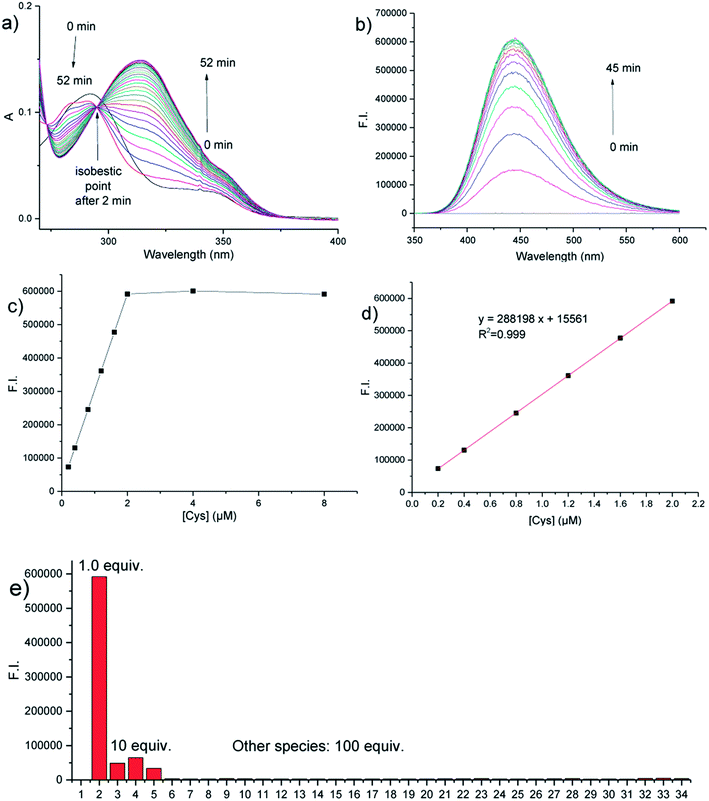 |
| Fig. 2 (a) Time-dependent UV-vis spectra of probe 1 (10 μM) upon the addition of 1 equiv. of Cys (a spectrum was recorded every 2 minutes); (b) time-dependent fluorescence emission spectra of probe 1 (2 μM) upon the addition of 1 equiv. of Cys (a spectrum was recorded every 3 minutes); (c) time-dependent fluorescence emission intensity at 446 nm of probe 1 (2 μM) upon addition of Cys (0 to 4 equiv.); (d) a linear relationship of the fluorescence intensity at 446 nm versus the Cys concentration (0.2–2.0 μM); (e) fluorescence response of probe 1 (2 μM) at 446 nm toward various species in PBS buffer (10 mM, pH 7.4): (1) blank; (2) Cys; (3) Hcy; (4) GSH; (5) NAC; (6) valine; (7) glycine; (8) isoleucine; (9) lysine; (10) leucine; (11) histidine; (12) asparagine; (13) methionine; (14) proline; (15) serine; (16) alanine; (17) threonine; (18) arginine; (19) glutamine; (20) aspartic acid; (21) glutamic acid; (22) tyrosine; (23) tryptophan; (24) phenylalanine; (25) glucose; (26) H2O2; (27) Na+; (28) K+; (29) Ca2+; (30) Mg2+; (31) Fe3+; (32) Fe2+; (33); Cu2+; (34) Zn2+ (All measurements were made in 10 mM PBS buffer, pH 7.4, 25 °C, and λex = 314 nm). | |
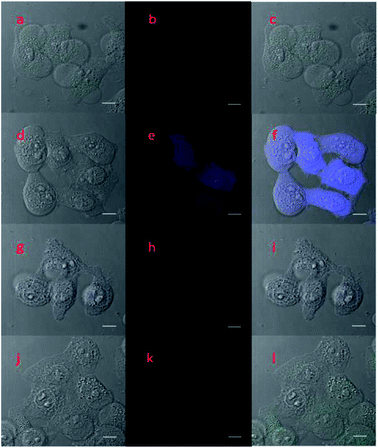 |
| Fig. 3 Two-photon fluorescence images (b, e, h, k) of HeLa cells collected at 410–510 nm (blue to cyan-blue, λex = 690 nm), the corresponding bright field view (a, d, g, j), and overlap of the fluorescence channel and the bright field view (c, f, i, l) after different treatments: (a–c) the cells were pretreated with 0.5 mM of N-ethylmaleimide (NEM) for 30 min and then incubated with 10 μM of probe 1 for 30 min; (d–f) cells were first pretreated with 0.5 mM of NEM for 30 min, then after addition of 1 mM of Cys were incubated for 30 min, and finally, incubated with 10 μM of probe 1 for 30 min (scale bar = 10 μm); the conditions for (g–i) and (j–l) were similar to those of (d–f), except that 10 μM of Hcy and 10 μM of GSH were used instead of 10 μM of Cys. | |
Encouraged by the fast, selective, and sensitive in vitro fluorescence response of probe 1 for the detection of Cys, we further evaluated its potential use as a two-photon imaging agent for Cys in biological systems, such as in living cells. The fluorescence response of probe 1 towards Cys at different pH values was evaluated and a suitable pH range for Cys detection was determined to be 7.0 to 10.0, which is a good range for cell imaging applications because physiological conditions have a pH of around 7.4 (see Fig. S7 in the ESI†). HeLa cells were then pretreated with N-ethylmaleimide (NEM, 0.5 mM) for 30 min to remove the endogenous cellular thiols, and incubated with Cys (1 mM), Hcy (1 mM), or GSH (1 mM), respectively for 30 min to increase the specific thiol levels. The samples were then further incubated with probe 1 (10 μM) for 30 min and were then washed with PBS buffer before two-photon fluorescence cell images and the corresponding bright-field view images were taken (Fig. 3(d–l)). Control images were also taken for samples pretreated with NEM (0.5 mM) and then incubated with probe 1 (10 μM) (Fig. 3a–c). Only cells pretreated with NEM and then Cys showed a distinctive blue fluorescence (Fig. 3e). The above cell imaging studies clearly demonstrated that probe 1 is capable of the selective detection and imaging of intracellular Cys over Hcy and GSH in living cells by two-photon fluorescence imaging with low background fluorescence interference.
Conclusions
We have demonstrated that N-(6-acyl-2-naphthayl)-maleimide (1) is a highly sensitive and selective simple fluorescent probe for the turn-on detection of Cys over other biothiols, including Hcy and GSH, with a detection limit of as low as 1.4 nM. The probe has very low fluorescence background due to a dual quenching mechanism of PeT and ICT. Upon reaction with Cys via a thiol-Michael addition and intramolecular transcyclization cascade reaction, the maleimide group at the 2-position was replaced by a better electron-donating succinamide group, resulting in more than a 3000-fold fluorescence enhancement due to the removal of dual quenching effects. The probe provided additional evidence of the rational design of a Cys-selective fluorescent probe by tuning the fluorescence turn-on response only at the Cys-selective transcyclization step in a two-step thiol-Michael-addition-transcyclization cascade reaction via a dual PeT/ICT mechanism, suggesting that the design of a N-fluorophore-substituted maleimide with dual PeT/ICT quenching, initially demonstrated on the 1,8-naphthalimide fluorophore platform,28 is a rather general approach for obtaining a Cys-selective turn-on fluorescent probe with high selectivity and sensitivity. Moreover, the probe was successfully applied in the two-photon imaging of intracellular Cys in cultured HeLa cells excited by a near-infrared 690 nm laser.
Conflicts of interest
There are no conflicts to declare.
Acknowledgements
This work was supported by the National Natural Science Foundation of China (Grant No. 21577037, K. L.) and the East China University of Science and Technology (start-up funds, W. W.).
Notes and references
- R. O. Ball, G. Courtney-Martin and P. B. Pencharz, J. Nutr., 2006, 136, 1682S CrossRef CAS PubMed.
- K. G. Reddie and K. S. Carroll, Curr. Opin. Chem. Biol., 2008, 12, 746 CrossRef CAS PubMed.
- X. F. Wang and M. S. Cynader, J. Neurosci., 2001, 21, 3322 CrossRef CAS PubMed.
- D. H. Baker and G. L. Czarnecki-Maulden, J. Nutr., 1987, 117, 1003 CrossRef CAS PubMed.
- J. Selhub, Annu. Rev. Nutr., 1999, 19, 217 CrossRef CAS PubMed.
- H. Sies, Free Radical Biol. Med., 1999, 27, 916 CrossRef CAS PubMed.
- S. Shahrokhian, Anal. Chem., 2001, 73, 5972 CrossRef CAS PubMed.
- S. Seshadri, A. Beiser, J. Selhub, P. F. Jacques, I. H. Rosenberg, R. B. D'Agostino, P. W. F. Wilson and P. A. Wolf, N. Engl. J. Med., 2002, 346, 476 CrossRef CAS PubMed.
- A. A. Mangoni and S. H. D. Jackson, Am. J. Med., 2002, 112, 556 CrossRef CAS PubMed.
- D. M. Townsend, K. D. Tew and H. Tapiero, Biomed. Pharmacother., 2003, 57, 145 CrossRef CAS.
- W. H. Wang, O. Rusin, X. Y. Xu, K. K. Kim, J. O. Escobedo, S. O. Fakayode, K. A. Fletcher, M. Lowry, C. M. Schowalter, C. M. Lawrence, F. R. Fronczek, I. M. Warner and R. M. Strongin, J. Am. Chem. Soc., 2005, 127, 15949 CrossRef CAS PubMed.
- T. Inoue and J. R. Kirchhoff, Anal. Chem., 2002, 74, 1349 CrossRef CAS PubMed.
- W. Wang, L. Li, S. Liu, C. Ma and S. Zhang, J. Am. Chem. Soc., 2008, 130, 10846 CrossRef CAS PubMed.
- F.-J. Huo, Y.-Q. Sun, J. Su, J.-B. Chao, H.-J. Zhi and C.-X. Yin, Org. Lett., 2009, 11, 4918 CrossRef CAS PubMed.
- X. Chen, Y. Zhou, X. Peng and J. Yoon, Chem. Soc. Rev., 2010, 39, 2120 RSC.
- H. S. Jung, X. Chen, J. S. Kim and J. Yoon, Chem. Soc. Rev., 2013, 42, 6019 RSC.
- H. Chen, Y. Tang and W. Lin, TrAC, Trends Anal. Chem., 2016, 76, 166 CrossRef CAS.
- Y. Yang, Q. Zhao, W. Feng and F. Li, Chem. Rev., 2013, 113, 192 CrossRef CAS PubMed.
- X. Qian, Y. Xiao, Y. Xu, X. Guo, J. Qian and W. Zhu, Chem. Commun., 2010, 46, 6418 RSC.
- Y. Tang, D. Lee, J. Wang, G. Li, J. Yu, W. Lin and J. Yoon, Chem. Soc. Rev., 2015, 44, 5003 RSC.
- L.-Y. Niu, Y.-Z. Chen, H.-R. Zheng, L.-Z. Wu, C.-H. Tung and Q.-Z. Yang, Chem. Soc. Rev., 2015, 44, 6143 RSC.
- O. Rusin, N. N. St Luce, R. A. Agbaria, J. O. Escobedo, S. Jiang, I. M. Warner, F. B. Dawan, K. Lian and R. M. Strongin, J. Am. Chem. Soc., 2004, 126, 438 CrossRef CAS PubMed.
- X. Yang, Y. Guo and R. M. Strongin, Angew. Chem., Int. Ed., 2011, 50, 10690 CrossRef CAS PubMed.
- L. Long, W. Lin, B. Chen, W. Gao and L. Yuan, Chem. Commun., 2011, 47, 893 RSC.
- L.-Y. Niu, Y.-S. Guan, Y.-Z. Chen, L.-Z. Wu, C.-H. Tung and Q.-Z. Yang, J. Am. Chem. Soc., 2012, 134, 18928 CrossRef CAS PubMed.
- L.-Y. Niu, Y.-S. Guan, Y.-Z. Chen, L.-Z. Wu, C.-H. Tung and Q.-Z. Yang, Chem. Commun., 2013, 49, 1294 RSC.
- J. Liu, Y.-Q. Sun, H. Zhang, Y. Huo, Y. Shi and W. Guo, Chem. Sci., 2014, 5, 3183 RSC.
- X. Li, Y. Zheng, H. Tong, R. Qian, L. Zhou, G. Liu, Y. Tang, H. Li, K. Lou and W. Wang, Chem.–Eur. J., 2016, 22, 9247 CrossRef CAS PubMed.
- H. Tong, Y. Zheng, L. Zhou, X. Li, R. Qian, R. Wang, J. Zhao, K. Lou and W. Wang, Anal. Chem., 2016, 88, 10816 CrossRef PubMed.
- H. Tong, J. Zhao, X. Li, Y. Zhang, S. Ma, K. Lou and W. Wang, Chem. Commun., 2017, 53, 3583 RSC.
- R. K. Meka and M. D. Heagy, J. Org. Chem., 2017, 82, 12153 CrossRef CAS PubMed.
- L. He, B. Dong, Y. Liu and W. Lin, Chem. Soc. Rev., 2016, 45, 6449 RSC.
- H. Zhang, C. Zhang, R. Liu, L. Yi and H. Sun, Chem. Commun., 2015, 51, 2029 RSC.
- Y. Matsumura and H. N. Ananthaswamy, Toxicol. Appl. Pharmacol., 2004, 195, 298 CrossRef CAS PubMed.
- M. Monici, Biotechnol. Annu. Rev., 2005, 11, 227 CAS.
- H. M. Kim and B. R. Cho, Chem. Rev., 2015, 115, 5014 CrossRef CAS PubMed.
- Z. Xiang and L. Wang, J. Org. Chem., 2011, 76, 6367 CrossRef CAS PubMed.
- S. Singha, D. Kim, B. Roy, S. Sambasivan, H. Moon, A. S. Rao, J. Y. Kim, T. Joo, J. W. Park, Y. M. Rhee, T. Wang, K. H. Kim, Y. H. Shin, J. Jung and K. H. Ahn, Chem. Sci., 2015, 6, 4335 RSC.
- M. Wen, X. Guo, P. Sun, L. Xiao, J. Li, Y. Xiong, J. Bao, T. Xue, L. Zhang and C. Tian, Chem. Commun., 2015, 51, 8153 RSC.
Footnotes |
† Electronic supplementary information (ESI) available: Details of the synthetic procedures, additional fluorescence spectra, and NMR and MS spectra. See DOI: 10.1039/c8ra02138d |
‡ These two authors contributed equally. |
|
This journal is © The Royal Society of Chemistry 2018 |