DOI:
10.1039/C8RA01648H
(Paper)
RSC Adv., 2018,
8, 14171-14176
One-pot, sequential four-component synthesis of novel heterocyclic [3.3.3] propellane derivatives at room temperature†
Received
24th February 2018
, Accepted 9th April 2018
First published on 17th April 2018
Abstract
An efficient, one-pot, two-step, four-component reaction for the synthesis of propellane derivatives is described by the condensation reaction between acenaphthenequinone, malono derivatives, primary amines and β-ketoester or β-diketone derivatives in the presence of triethylamine in ethanol at room temperature. Using this procedure, all the products were obtained in good to excellent yields.
Introduction
In modern organic chemistry, the improvement of reaction efficiency, the reduction of waste, the avoidance of toxic reagents and the responsible utilization of our resources have become critical objectives.1,2 Multi-component reactions, are useful tools for the synthesis of biologically and pharmacologically important compounds because of their environmentally friendly, green characteristics and atom efficient.3–6 Therefore, the design and application of multi-component reactions has attracted great attention from research groups working in areas such as biological, medicinal and organic chemistry.
Heterocyclic compounds occur commonly in nature and are essential to life.7 These compounds have received considerable attention in recent times due to their wide applications. Functionalized nitrogen and oxygen-containing heterocycles are of importance as they have various industrial, pharmaceutical and biological applications.8–20
Propellanes are tricyclic compounds in which the three rings are connected to a common C–C single bond.21 Nitrogen- and oxygen-containing propellanes and their analogues constitute the structural framework of many natural and non-natural products such as periglaucine A, hasubanone, sinoacutine and merrilactone A (Fig. 1).22,23 Propellanes due to their challenging framework and broad spectra of biological and pharmacological activities, constitute an interesting class of compounds in organic chemistry.24
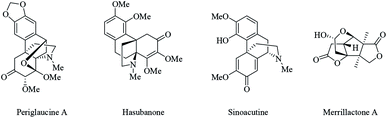 |
| Fig. 1 Typical natural and biological products containing propellane skeleton. | |
Recently, there have been some reports about the synthesis of propellane compounds from ninhydrin25–32 and acenaphthoquinone.32–34 In continuation of our research on the synthesis of biologically important heterocycles and green chemistry protocols,35–47 we report a simple and efficient method for the synthesis of novel propellane derivatives via a one-pot, sequential four-component condensation reaction between acenaphthoquinone (1), malono derivatives (2), primary amines (4) and β-ketoester or β-diketone (5) derivatives in the presence of triethylamine in ethanol at room temperature (Scheme 1).
 |
| Scheme 1 The synthesis of propellane derivatives via the reaction between acenaphthoquinone, malono derivatives, dicarbonyl compounds and primary amines in the presence of Et3N in EtOH at room temperature. | |
Results and discussion
In the first step, the reaction between acenaphthoquinone (1), malononitrile (2a), ethyl acetoacetate (5a) and benzyl amine (4c), as a model reaction, was investigated in the presence of different molar ratios of Et3N in some solvents (5 mL) at room temperature and the results are summarized in Table 1. For this propose to a stirred mixture of acenaphthoquinone 1 (1 mmol) and malononitrile 2a (1 mmol) in EtOH (5 mL) at room temperature different amount of Et3N were added to form the corresponding Knoevenagel adduct and then ethyl acetoacetate 5a (1 mmol) and benzyl amine 4c (1 mmol) were added to obtain the corresponding product 6a. As it is shown in Table 1, higher yield and shorter reaction time were obtained when the reaction was carried out in the presence of 1 mmol of the Et3N in ethanol at room temperature (Table 1, entry 4). It should be mention that simultaneity of this four-component condensation reaction was studied in the presence of 1 mmol Et3N in EtOH at room temperature and we observed that benzyl amine has remained intact in this condition and the corresponding propellane was not formed.
Table 1 Optimization of the reaction conditionsa
Entry |
Amount of Et3N (mmol) |
Solvent |
Time (h) |
Yieldb (%) |
Triethylamine (1.0 mmol) was added to a stirred mixture of acenaphthoquinone 1 (1 mmol), malononitrile 2a (1 mmol) in EtOH (5 mL) at room temperature and after about 10 min to form the corresponding Knoevenagel adduct, then ethyl acetoacetate 5a (1 mmol) and benzyl amine 4c (1 mmol) were added to obtain the corresponding product 6a. Isolated yields. |
1 |
— |
EtOH |
5 |
— |
2 |
0.1 |
EtOH |
5 |
45 |
3 |
0.5 |
EtOH |
5 |
87 |
4 |
1.0 |
EtOH |
1.2 |
91 |
5 |
1.5 |
EtOH |
1.2 |
91 |
6 |
1.0 |
H2O |
2.0 |
70 |
7 |
1.0 |
DMF |
4.0 |
60 |
8 |
1.0 |
MeOH |
1.5 |
85 |
9 |
1.0 |
CHCl3 |
4.0 |
40 |
10 |
1.0 |
THF |
4.0 |
55 |
11 |
1.0 |
CH3CN |
40 |
45 |
In the next step, the scope and efficiency of the process was explored under the optimized conditions. For this purpose, acenaphthoquinone was condensed with alkyl malonates, aliphatic and aromatic amines and dicarbonyl compounds in the presence of Et3N (1 mmol) to afford the corresponding products 6. The structural diversity of reactants is summarized in Fig. 2 and the results are displayed in Table 2.
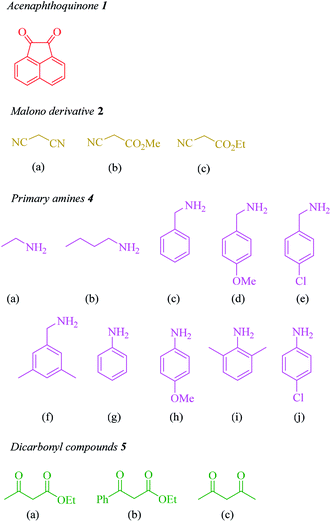 |
| Fig. 2 Diversity elements employed for synthesis of propellane derivatives. | |
Table 2 One-pot, sequential four component synthesis of propellane derivatives in the presence of Et3N (1 mmol) in EtOH at room temperature.a,b
Triethylamine (1.0 mmol) was added to a stirred mixture of acenaphthoquinone 1 (1 mmol), malono derivatives 2 (1 mmol) in EtOH (5 mL) at room temperature to form the corresponding Knoevenagel adduct (about 10–30 min), then dicarbonyl compound 5 (1 mmol) and primary amine 4 (1 mmol) were added to obtain the desired product 6. Isolated yields. |
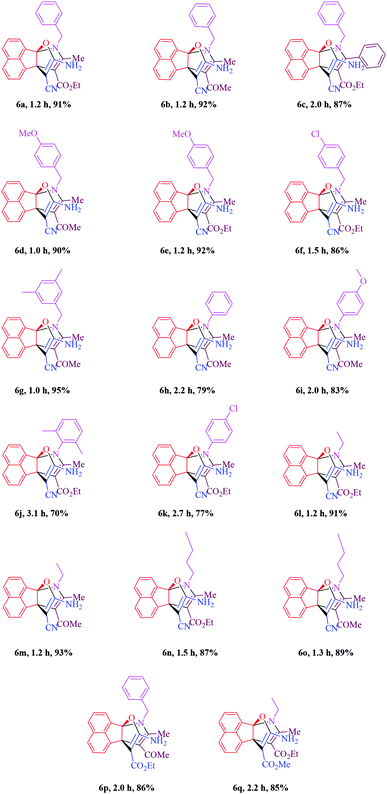 |
The synthetic pathway for the synthesis of titled compounds is consisting of two steps. At first, compound 3 are obtained from acenaphthoquinone 1 and alkyl malonates 2. Then, the resulting products are treated with primary amines 4 and β-dicarbonyl compounds 5 to afford the related propellane derivatives as the desired products. As Table 2 indicates, a variety of alkyl malonates, aliphatic and aromatic amines and β-dicarbonyl compounds were successfully applied in this process to afford the corresponding propellane derivatives as novel compounds in good to excellent yields.
To demonstrate the generality and scope of this method, malono derivatives, various acyclic β-dicarbonyl compounds such as methyl acetoacetate, ethyl acetoacetate and ethyl benzoylacetate were treated with a broad range of aliphatic and aromatic amines in the presence of Et3N (1 mmol) in EtOH at room temperature and the results are shown in Table 2. Use of malononitrile leads to shorter reaction times and higher yields compared to application of other alkylmalonates such as methyl cyanoacetate or ethyl cyanoacetate (compare 6b with 6p and 6l with 6q). As it is shown in Table 2, benzyl amines bearing electron-donating or -withdrawing substituents gave the desired propellanes in high yields and in short reaction times and it seems that when the methoxy group is placed on the aromatic ring, reactivity is better than when the chlorine group is on the ring. Moreover, the presence of 3,5-dimethyl group on the aromatic ring has the best efficiency. Some aromatic amines were also screened to carry out the four-component reaction by this method and the results are listed in Table 2 (entries 6h–6j). Generally, aliphatic amines are more reactive than aromatic amines. Ortho-substituted anilines, whatever the nature of the substituted groups, required a longer reaction period. For instance, reaction of acenaphthenequinone, malononitrile and 2,6-dimethylaniline with ethyl acetoacetate gave the desired product (6j) in 70% yield after 3.1 h. Moreover, aliphatic amines such as ethyl amine and butyl amine were used for the synthesis of the corresponding product in excellent yields (Table 2, entries 6l–6o). In the same way, Some cyclic and acyclic 1.3-dicarbonyl compounds were also screened to carry out the one pot four-component reaction by this method and the results are listed in Table 2. As it is shown in Table 2, aliphatic β-keto esters are more reactive than aromatic β-keto esters (compare 6a and 6c) and no products were obtained when cyclic 1.3-dicarbonyl compounds were involved in this one-pot room temperature reaction.
The proposed mechanism for the synthesis of propellane compounds 6 in the presence of Et3N is shown in Scheme 2. Based on this mechanism, Et3N is effective catalyst for the formation of olefin (3) which readily prepares in situ by Knoevenagel condensation of acenaphthoquinone (1) with the active methylene of compound (2). Next, the formation of enamine (7) occurs through condensation of amine (4) with dicarbonyl compound (5). Then, the enamine (7) attacks to olefin (3) in a Michael addition to produce intermediate (8). After proton transfer and tautomerization, intermediate (8) converts to intermediate (10), through intramolecular cyclization by nitrogen attack of enamine to carbonyl group. In this stage, nucleophilic addition of amino group to C
O bond afforded intermediate (10). Then, intramolecular O-cyclization and the tautomerization of imino group to amino group lead to propellanes (6).
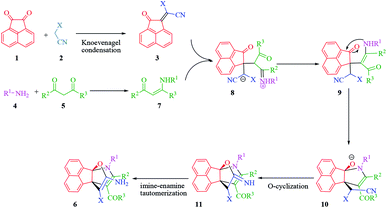 |
| Scheme 2 The proposed mechanism for the synthesis of propellane 6 in the presence of Et3N. | |
Experimental
All chemicals were purchased from Merck or Fluka chemical companies. The 1H NMR (400 MHz) and 13C NMR (100 MHz) were run on a Bruker Avance 400. Melting points were recorded on a Stuart Scientific Apparatus SMP3 (UK) in open capillary tubes. Elemental C, H and N analyses were performed using a Costech CHNS-O elemental analyzer.
General procedure for the synthesis of propellane derivatives 6
Triethylamine (1.0 mmol) was added to a stirred mixture of acenaphthoquinone 1 (1 mmol), malono derivatives 2 (1 mmol) in EtOH (5 mL) and the reaction mixture was stirred to complete the formation of related Knoevenagel adduct 3 (monitored by TLC). Subsequently, dicarbonyl compound 5 (1 mmol) and primary amine 4 (1 mmol) were added to this reaction mixture at once and dropwise over 10 min, respectively and reacted at room temperature for the appropriate amount of time (see Table 2). After completion of the reaction confirmed by TLC, the reaction mixture was filtered and washed with ethanol to afford the pure product 6a–l.
Ethyl-11-amino-7-benzyl-10-cyano-8-methyl-7H-6b,9a-(epoxyetheno)acenaphtho[1,2-b]pyrrole-9-carboxylate 6a. White powder, mp = 249–251 °C, 1H NMR (DMSO-d6, 400 MHz) δ (ppm): 1.45 (t, J = 6.6 Hz, 3H), 2.25 (s, 3H), 4.04 (q, J = 7.2 Hz, 2H), 4.54 (d, J = 16.0 Hz, 1H), 4.60 (d, J = 16.0 Hz, 1H), 7.57 (s, 2H), 7.66–7.71 (m, 3H), 7.73–7.81 (m, 4H), 7.89 (d, J = 8.0 Hz, 2H), 8.04 (d, J = 7.2 Hz, 2H). 13C NMR (DMSO-d6, 100 MHz) δ (ppm): 14.4, 14.6, 58.1, 60.5, 61.2, 70.7, 79.9, 110.4, 118.4, 120.6, 121.0, 124.7, 125.8, 127.8, 129.2, 129.6, 132.0, 135.8, 136.3, 142.7, 147.2, 147.6, 147.7, 162.4, 166.5, 166.9, 168.0, 168.1. Anal. calcd for C28H23N3O3: C, 74.82; H, 5.16; N, 9.35%. Found: C, 74.85; H, 5.14; N, 9.33%. IR (KBr) (νmax, cm−1): 3358 and 3192 (NH2), 2193 (CN), 1687 (C
O), 1652 (OC
C).
9-Acetyl-11-amino-7-benzyl-8-methyl-7H-6b,9a-(epoxyetheno)acenaphtho[1,2-b]pyrrole-10-carbonitrile 6b. White powder, mp = 250–252 °C, 1H NMR (DMSO-d6, 400 MHz) δ (ppm): 2.32 (s, 3H), 2.36 (s, 3H), 4.74 (d, J = 15.6 Hz, 1H), 4.99 (d, J = 15.6 Hz, 1H), 7.45 (s, 2H), 7.63 (t, J = 7.4 Hz, 2H), 7.69–7.71 (m, 2H), 7.73–7.77 (m, 3H), 7.85 (d, J = 7.6 Hz, 2H), 8.02 (d, J = 8.0 Hz, 2H). 13C NMR (DMSO-d6, 100 MHz) δ (ppm): 15.9, 30.3, 58.4, 59.1, 72.2, 79.8, 116.4, 116.6, 118.5, 120.4, 121.4, 121.6, 124.4, 124.9, 127.7, 128.9, 129.5, 131.9, 135.9, 136.3, 143.0, 148.0, 156.6, 157.1, 166.5, 167.2, 192.7. Anal. calcd for C27H21N3O2: C, 77.31; H, 5.05; N, 10.02%. Found: C, 77.32; H, 5.02; N, 10.05%.
Ethyl-11-amino-7-benzyl-10-cyano-8-phenyl-7H-6b,9a-(epoxyetheno)acenaphtho[1,2-b]pyrrole-9-carboxylate 6c. White powder, mp = 247–249 °C, 1H NMR (DMSO-d6, 400 MHz) δ (ppm): 1.38 (t, J = 7.0 Hz, 3H), (q, J = 6.8 Hz, 2H), 4.92 (d, J = 16.0 Hz, 1H), 4.98 (d, J = 16.0 Hz, 1H), 7.43 (t, J = 7.4 Hz, 3H), 7.51 (t, J = 7.0 Hz, 3H), 7.66 (s, 2H), 7.70–7.77 (m, 4H), 7.83 (d, J = 6.8 Hz, 1H), 7.87 (d, J = 7.2 Hz, 1H), 7.93 (d, J = 8.0 Hz, 2H), 8.07 (d, J = 8.0 Hz, 2H). 13C NMR (DMSO-d6, 100 MHz) δ (ppm): 15.3, 43.6, 47.1, 47.2, 72.2, 79.9, 113.0, 116.3, 117.3, 117.5, 121.2, 123.4, 125.4, 125.7, 127.0, 127.7, 127.9, 128.3, 130.3, 134.5, 134.7, 135.6, 139.3, 147.4, 152.6, 155.4, 158.5, 158.5, 159.1, 159.3, 162.2, 168.1. Anal. calcd for C33H25N3O3: C, 77.48; H, 4.93; N, 8.21%. Found: C, 77.51; H, 4.91; N, 8.19%.
9-Acetyl-11-amino-7-(4-methoxybenzyl)-8-methyl-7H-6b,9a-(epoxyetheno)acenaphtho[1,2-b]pyrrole-10-carbonitrile 6d. White powder, mp = 249–251 °C, 1H NMR (DMSO-d6, 400 MHz) δ (ppm): 2.32 (s, 3H), 2.37 (s, 3H), 3.75 (s, 3H), 4.67 (d, J = 16.0 Hz, 1H), 4.78 (d, J = 16.0 Hz, 1H), 7.35 (d, J = 6.8 Hz, 2H), 7.55–7.59 (m, 2H), 7.77 (t, J = 7.6 Hz, 2H), 7.84–7.89 (m, 2H), 8.09 (s, 2H), 8.17 (d, J = 7.6 Hz, 2H). 13C NMR (DMSO-d6, 100 MHz) δ (ppm): 15.9, 30.3, 57.6, 58.0, 58.4, 72.2, 79.9, 118.5, 120.4, 121.4, 121.7, 124.5, 124.9, 127.7, 128.9, 129.5, 131.9, 135.9, 136.3, 140.0, 140.1, 140.6, 140.8, 143.8, 166.6, 167.2, 168.6, 192.7. Anal. calcd for C28H23N3O3: C, 74.82; H, 5.16; N, 9.35%. Found: C, 74.79; H, 5.17; N, 9.38%. IR (KBr) (νmax, cm−1): 3361 and 3101 (NH2), 2195 (CN), 1711 (C
O), 1644 (OC
C).
Ethyl-11-amino-10-cyano-7-(4-methoxybenzyl)-8-methyl-7H-6b,9a-(epoxyetheno)acenaphtho[1,2-b]pyrrole-9-carboxylate 6e. White powder, mp = 251–253 °C, 1H NMR (DMSO-d6, 400 MHz) δ (ppm): 0.57 (t, J = 7.6 Hz, 3H), 1.90 (s, 3H), 3.10 (s, 3H), 3.45–3.49 (m, 1H), 3.79–3.82 (m, 1H), 4.77 (d, J = 16.0 Hz, 1H), 5.03 (d, J = 15.6 Hz, 1H), 7.57 (s, 2H), 7.66–7.71 (m, 2H), 7.73–7.76 (m, 2H), 7.79 (t, J = 6.0 Hz, 2H), 7.88 (d, J = 8.0 Hz, 2H), 8.03 (d, J = 7.6 Hz, 2H). 13C NMR (DMSO-d6, 100 MHz) δ (ppm): 14.4, 14.6, 47.5, 69.4, 70.0, 73.1, 73.3, 79.7, 106.1, 116.2, 117.6, 121.2, 125.1, 127.0, 127.7, 127.9, 128.2, 130.2, 134.7, 135.5, 139.3, 147.4, 158.7, 159.3, 159.8, 160.7, 162.3, 165.0, 168.8. Anal. calcd for C29H25N3O4: C, 72.64; H, 5.25; N, 8.76%. Found: C, 72.62; H, 5.23; N, 8.79%.
Ethyl-11-amino-7-(4-chlorobenzyl)-10-cyano-8-methyl-7H-6b,9a-(epoxyetheno)acenaphtho[1,2-b]pyrrole-9-carboxylate 6f. White powder, mp = 249–251 °C, 1H NMR (DMSO-d6, 400 MHz) δ (ppm): 1.44 (t, J = 7.0 Hz, 3H), 2.25 (s, 3H), 4.11–4.17 (m, 2H), 4.42 (d, J = 16.0 Hz, 1H), 4.48 (d, J = 16.0 Hz, 1H), 7.77 (s, 2H), 7.85–7.91 (m, 2H), 7.92–7.96 (m, 3H), 7.99 (t, J = 6.0 Hz, 1H), 8.08 (d, J = 8.4 Hz, 2H), 8.23 (d, J = 8.0 Hz, 2H). 13C NMR (DMSO-d6, 100 MHz) δ (ppm): 14.4, 14.6, 58.1, 60.6, 60.6, 70.7, 79.9, 110.4, 118.4, 120.6, 121.0, 124.7, 125.8, 127.8, 129.1, 129.6, 132.0, 135.8, 136.3, 142.7, 154.8, 155.1, 155.8, 157.5, 157.8, 166.6, 167.2, 167.3. Anal. calcd for C28H22ClN3O3: C, 69.49; H, 4.58; Cl, 7.33; N, 8.68%. Found: C, 69.50; H, 4.56; N, 8.70%.
9-Acetyl-11-amino-7-(3,5-dimethylbenzyl)-8-methyl-7H-6b,9a-(epoxyetheno)acenaphtho[1,2-b]pyrrole-10-carbonitrile 6g. White powder, mp = 251–253 °C, 1H NMR (DMSO-d6, 400 MHz) δ (ppm): 2.05 (s, 3H), 2.10 (s, 3H), 2.31 (s, 3H), 2.36 (s, 3H), 4.96 (d, J = 16.0 Hz, 1H), 5.02 (d, J = 16.0 Hz, 1H), 6.83 (d, J = 7.6 Hz, 1H), 7.02 (t, J = 7.4 Hz, 1H), 7.21–7.35 (m, 2H), 7.49 (d, J = 7.2 Hz, 2H), 7.58 (d, J = 9.2 Hz, 1H), 7.79 (s, 2H), 7.85 (dd, J = 2.4 Hz, 8.8 Hz, 1H), 8.02 (d, J = 2.4 Hz, 1H). 13C NMR (DMSO-d6, 100 MHz) δ (ppm): 14.1, 20.2, 20.5, 30.3, 47.4, 59.3, 71.9, 79.7, 103.9, 108.4, 112.9, 116.9, 122.4, 123.4, 123.6, 125.4, 127.7, 128.4, 128.7, 134.1, 134.5, 137.2, 145.4, 152.4, 154.5, 158.4, 159.2, 167.5, 198.2. Anal. calcd for C29H25N3O2: C, 77.83; H, 5.63; N, 9.39%. Found: C, 77.80; H, 5.65; N, 9.41%.
9-Acetyl-11-amino-8-methyl-7-phenyl-7H-6b,9a-(epoxyetheno)acenaphtho[1,2-b]pyrrole-10-carbonitrile 6h. White powder, mp = 247–249 °C, 1H NMR (DMSO-d6, 400 MHz) δ (ppm): 2.31 (s, 3H), 2.36 (s, 3H), 7.51–7.60 (m, 3H), 7.78–7.82 (m, 2H), 7.88 (s, 2H), 7.97 (dd, J = 1.4 Hz, 7.8 Hz, 2H), 8.23 (dd, J = 2.2 Hz, 8.6 Hz, 2H), 8.38 (d, J = 2.4 Hz, 2H). 13C NMR (DMSO-d6, 100 MHz) δ (ppm): 15.8, 30.2, 57.2, 71.4, 79.8, 101.7, 109.6, 112.9, 117.2, 117.5, 123.2, 123.4, 124.6, 125.5, 127.6, 127.8, 128.9, 129.5, 132.8, 134.3, 136.4, 143.2, 152.6, 155.8, 158.9, 199.0. Anal. calcd for C26H19N3O2: C, 77.02; H, 4.72; N, 10.36%. Found: C, 77.04; H, 4.75; N, 10.32%.
9-Acetyl-11-amino-7-(4-methoxyphenyl)-8-methyl-7H-6b,9a-(epoxyetheno)acenaphtho[1,2-b]pyrrole-10-carbonitrile 6i. White powder, mp = 252–254 °C, 1H NMR (DMSO-d6, 400 MHz) δ (ppm): 2.22 (s, 3H), 2.93 (s, 3H), 3.74 (s, 3H), 7.53 (d, J = 6.8 Hz, 2H), 7.67 (t, J = 7.6 Hz, 1H), 7.89 (t, J = 7.4 Hz, 1H), 7.99–8.04 (m, 4H), 8.08 (s, 2H), 8.33 8.02 (d, J = 8.4 Hz, 2H). 13C NMR (DMSO-d6, 100 MHz) δ (ppm): 15.4, 30.0, 48.1, 57.5, 72.1, 79.2, 101.9, 110.0, 112.9, 117.1, 117.5, 122.5, 123.1, 124.6, 125.5, 129.4, 133.5, 134.2, 142.7, 152.5, 155.6, 158.8, 158.9, 166.1, 167.6, 177.6, 195.0. Anal. calcd for C27H21N3O3: C, 74.47; H, 4.86; N, 9.65%. Found: C, 74.43; H, 4.89; N, 9.67%.
Ethyl-11-amino-10-cyano-7-(2,6-dimethylphenyl)-8-methyl-7H-6b,9a-(epoxyetheno)acenaphtho[1,2-b]pyrrole-9-carboxylate 6j. White powder, mp = 255–257 °C, 1H NMR (DMSO-d6, 400 MHz) δ (ppm): 1.35 (t, J = 7.2 Hz, 3H), 2.21 (s, 3H), 2.30 (s, 3H), 2.33 (s, 3H), 3.31–3.34 (m, 2H), 7.35 (d, J = 6.8 Hz, 2H), 7.55–7.59 (m, 2H), 7.77 (t, J = 7.6 Hz, 2H), 7.84–7.89 (m, 2H), 8.10 (s, 2H), 8.17 (d, J = 7.6 Hz, 1H). 13C NMR (DMSO-d6, 100 MHz) δ (ppm): 13.6, 14.6, 21.4, 21.7, 59.6, 60.2, 72.0, 79.8, 104.3, 108.9, 112.8, 112.9, 116.8, 117.0, 121.5, 123.7, 124.1, 125.3, 125.6, 128.4, 133.9, 134.3, 135.2, 144.6, 152.3, 154.2, 167.6, 170.9, 179.4. Anal. calcd for C29H25N3O3: C, 75.14; H, 5.44; N, 9.07%. Found: C, 75.10; H, 5.42; N, 9.12%.
Ethyl-11-amino-7-(4-chlorophenyl)-10-cyano-8-methyl-7H-6b,9a-(epoxyetheno)acenaphtho[1,2-b]pyrrole-9-carboxylate 6k. White powder, mp = 251–253 °C, 1H NMR (DMSO-d6, 400 MHz) δ (ppm): 1.19 (t, J = 7.2 Hz, 3H), 2.00 (s, 3H), 4.04 (q, J = 7.2 Hz, 2H), 7.56 (d, J = 8.8 Hz, 1H), 7.65 (d, J = 7.2 Hz, 1H), 7.72 (t, J = 7.6 Hz, 1H), 7.77 (s, 2H), 7.84 (dd, J = 2.4 Hz, 8.8 Hz, 1H), 7.90 (t, J = 7.6 Hz, 1H), 8.03–8.06 (m, 3H), 8.37 (d, J = 8.0 Hz, 2H). 13C NMR (DMSO-d6, 100 MHz) δ (ppm): 13.7, 14.6, 59.8, 60.2, 71.9, 79.2, 103.0, 108.9, 113.1, 116.9, 119.8, 123.5, 125.3, 126.1, 134.1, 136.2, 142.4, 151.2, 152.4, 155.1, 158.7, 159.3, 167.2, 167.7, 180.2. Anal. calcd for C27H20ClN3O3: C, 69.01; H, 4.29; Cl, 7.54; N, 8.94%. Found: C, 69.05; H, 4.26; N, 8.92%.
Ethyl-11-amino-10-cyano-7-ethyl-8-methyl-7H-6b,9a-(epoxyetheno)acenaphtho[1,2-b]pyrrole-9-carboxylate 6l. White powder, mp = 250–252 °C, 1H NMR (DMSO-d6, 400 MHz) δ (ppm): 0.76 (t, J = 7.2 Hz, 3H), 1.44 (t, J = 7.0 Hz, 3H), 2.25 (s, 3H), 2.81 (q, J = 7.0 Hz, 2H), 4.30 (q, J = 7.0 Hz, 2H), 7.57 (s, 2H), 7.66–7.71 (m, 1H), 7.72–7.76 (m, 2H), 7.79 (t, J = 6.0 Hz, 1H), 7.88 (d, J = 8.4 Hz, 1H), 8.03 (d, J = 8.0 Hz, 1H). 13C NMR (DMSO-d6, 100 MHz) δ (ppm): 7.9, 14.4, 14.6, 58.1, 58.6, 60.5, 70.7, 79.9, 110.4, 118.4, 120.6, 121.0, 124.7, 125.8, 127.8, 129.1, 129.6, 132.0, 135.8, 136.3, 142.7, 167.3, 168.1. Anal. calcd for C23H21N3O3: C, 71.30; H, 5.46; N, 10.85%. Found: C, 71.28; H, 5.44; N, 10.88%. IR (KBr) (νmax, cm−1): 3405 and 3278 (NH2), 2197 (CN), 1663 (C
O), 1639 (OC
C).
9-Acetyl-11-amino-7-ethyl-8-methyl-7H-6b,9a-(epoxyetheno)acenaphtho[1,2-b]pyrrole-10-carbonitrile 6m. White powder, mp = 251–253 °C, 1H NMR (DMSO-d6, 400 MHz) δ (ppm): 0.86 (t, J = 7.0 Hz, 3H), 2.32 (s, 3H), 2.36 (s, 3H), 3.81 (q, J = 7.1 Hz, 2H), 7.46 (s, 2H), 7.63 (t, J = 7.6 Hz, 1H), 7.70 (d, J = 7.2 Hz, 1H), 7.75 (t, J = 7.0 Hz, 1H), 7.85 (d, J = 7.6 Hz, 2H), 8.01 (d, J = 7.6 Hz, 1H). 13C NMR (DMSO-d6, 100 MHz) δ (ppm): 15.3, 15.9, 30.3, 58.4, 59.0, 72.2, 79.9, 118.5, 120.4, 121.4, 121.6, 124.4, 124.9, 127.7, 128.9, 129.5, 131.9, 135.9, 136.3, 143.0, 167.2, 192.7. Anal. calcd for C22H19N3O2: C, 73.93; H, 5.36; N, 11.76%. Found: C, 73.92; H, 5.39; N, 11.73%.
Ethyl-11-amino-7-butyl-10-cyano-8-methyl-7H-6b,9a-(epoxyetheno)acenaphtho[1,2-b]pyrrole-9-carboxylate 6n. White powder, mp = 247–249 °C, 1H NMR (DMSO-d6, 400 MHz) δ (ppm): 0.95 (t, J = 7.2 Hz, 3H), 1.44 (t, J = 7.2 Hz, 3H), 1.80–1.92 (m, 4H), 2.25 (s, 3H), 2.98 (t, J = 6.4 Hz, 2H), 4.32 (q, J = 7.2 Hz, 2H), 7.57 (s, 2H), 7.67 (t, J = 7.6 Hz, 1H), 7.71 (d, J = 7.6 Hz, 1H), 7.75 (d, J = 6.4 Hz, 1H), 7.79 (t, J = 6.4 Hz, 1H), 7.88 (d, J = 8.0 Hz, 1H), 8.03 (d, J = 8.0 Hz, 1H). 13C NMR (DMSO-d6, 100 MHz) δ (ppm): 12.7, 14.4, 14.6, 19.0, 26.2, 55.1, 58.1, 60.5, 70.7, 79.9, 110.4, 118.4, 120.6, 121.0, 124.7, 125.8, 127.8, 129.1, 129.6, 132.0, 135.8, 136.3, 142.7, 167.3, 168.1. Anal. calcd for C25H25N3O3: C, 72.27; H, 6.06; N, 10.11%. Found: C, 72.30; H, 6.05; N, 10.08%. IR (KBr) (νmax, cm−1): 3389 and 3179 (NH2), 2203 (CN), 1661 (C
O), 1635 (OC
C).
9-Acetyl-11-amino-7-butyl-8-methyl-7H-6b,9a-(epoxyetheno)acenaphtho[1,2-b]pyrrole-10-carbonitrile 6o. White powder, mp = 248–250 °C, 1H NMR (DMSO-d6, 400 MHz) δ (ppm): 0.84 (t, J = 7.2 Hz, 3H), 1.05–1.12 (m, 4H), 2.31 (s, 3H), 2.36 (s, 3H), 2.96 (q, J = 7.3 Hz, 2H), 7.46 (s, 2H), 7.63 (t, J = 7.6 Hz, 1H), 7.70 (d, J = 7.2 Hz, 1H), 7.75 (t, J = 7.0 Hz, 1H), 7.85 (d, J = 7.2 Hz, 2H), 8.01 (d, J = 8.0 Hz, 1H). 13C NMR (DMSO-d6, 100 MHz) δ (ppm): 15.2, 15.9, 23.7, 29.9, 30.3, 58.4, 59.5, 72.2, 79.9, 118.5, 120.4, 121.4, 121.6, 124.4, 124.9, 127.7, 128.9, 129.5, 131.9, 135.9, 136.3, 166.6, 167.2, 192.7. Anal. calcd for C24H23N3O2: C, 74.78; H, 6.01; N, 10.90%. Found: C, 74.75; H, 6.03; N, 10.92%.
Ethyl-9-acetyl-11-amino-7-benzyl-8-methyl-7H-6b,9a-(epoxyetheno)acenaphtho[1,2-b]pyrrole-10-carboxylate 6p. White powder, mp = 249–251 °C, 1H NMR (DMSO-d6, 400 MHz) δ (ppm): 0.83 (t, J = 7.0 Hz, 3H), 2.10 (s, 3H), 2.44 (s, 3H), 3.80 (q, J = 7.3 Hz, 2H), 4.84 (d, J = 16.0 Hz, 1H), 5.12 (d, J = 16.0 Hz, 1H), 7.42 (s, 2H), 7.64–7.67 (m, 2H), 7.70–7.74 (m, 3H), 7.84 (d, J = 6.4 Hz, 3H), 7.99–8.06 (m, 3H). 13C NMR (DMSO-d6, 100 MHz) δ (ppm): 15.0, 15.1, 31.9, 58.7, 59.4, 71.8, 72.5, 80.0, 118.2, 118.4, 120.1, 120.8, 124.0, 124.1, 125.6, 127.5, 128.6, 129.1, 131.8, 136.2, 136.6, 142.9, 163.4, 166.1, 166.7, 167.3, 167.9, 195.3. Anal. calcd for C29H26N2O4: C, 74.66; H, 5.62; N, 6.00%. Found: C, 74.68; H, 5.59; N, 6.02%. IR (KBr) (νmax, cm−1): 3359 and 3161 (NH2), 1738 (C
O), 1667 (C
O), 1639 (OC
C).
9-Ethyl-10-methyl-11-amino-7-ethyl-8-methyl-7H-6b,9a-(epoxyetheno)acenaphtho[1,2-b]pyrrole-9,10-dicarboxylate 6q. White powder, mp = 247–249 °C, 1H NMR (DMSO-d6, 400 MHz) δ (ppm): 0.86 (t, J = 7.0 Hz, 3H), 1.18 (t, J = 7.2 Hz, 3H), 2.00 (s, 3H), 3.22 (s, 3H), 3.81 (q, J = 7.1 Hz, 2H), 4.04 (q, J = 7.2 Hz, 2H), 7.38 (s, 2H), 7.59 (t, J = 7.6 Hz, 1H), 7.63 (d, J = 7.2 Hz, 1H), 7.69 (t, J = 6.6 Hz, 1H), 7.81 (d, J = 8.4 Hz, 1H), 7.99 (d, J = 7.6 Hz, 1H), 8.08 (d, J = 6.8 Hz, 1H). 13C NMR (DMSO-d6, 100 MHz) δ (ppm): 14.5, 14.6, 15.1, 58.6, 60.1, 60.9, 61.7, 70.8, 79.8, 120.1, 124.0, 124.2, 126.0, 127.6, 128.5, 129.0, 131.7, 136.1, 136.6, 142.7, 164.6, 165.0, 166.2, 166.9. Anal. calcd for C24H24N2O5: C, 68.56; H, 5.75; N, 6.66%. Found: C, 68.58; H, 5.77; N, 6.63%. IR (KBr) (νmax, cm−1): 3412 and 3249 (NH2), 1736 (C
O), 1675 (C
O), 1642 (OC
C).
Conclusions
In conclusion, we have reported a highly efficient method for the synthesis of biologically important propellane derivatives via a one-pot, sequential four-component condensation reaction presence of triethylamine in ethanol at room temperature. High atom-economy, simple procedure in the excellent yields, easy workup procedure, mild reaction conditions and metal-catalyst free are the main advantages of this method.
Conflicts of interest
There are no conflicts to declare.
Acknowledgements
The authors thank Persian Gulf University Research Councils for the financial support of this work.
References
- D. B. Ramachary and M. Kishor, J. Org. Chem., 2007, 72, 5056 CrossRef CAS PubMed.
-
(a) L. D. S. Yadav, S. Singh and V. K. Rai, Green Chem., 2009, 11, 878 RSC;
(b) W. Leitner, Green Chem., 2009, 11, 603 RSC.
- A. Hasaninejad, A. Zare and M. Shekouhy, Tetrahedron, 2011, 67, 390 CrossRef CAS.
- B. Maleki, G. Esmailian and R. Tayebee, Org. Prep. Proced. Int., 2015, 47, 461 CrossRef CAS.
- M. Dabiri, Z. N. Tisseh, M. Bahramnejad and A. Bazgir, Ultrason. Sonochem., 2011, 18, 1153 CrossRef CAS PubMed.
- J. Zhu and H. Bienayme, Multicomponent Reactions, John Wiley & Sons, 2006 Search PubMed.
- T. Amanpour, A. Bazgir, A. M. Ardekani and R. Ghahremanzadeh, Monatsh. Chem., 2014, 145, 627 CrossRef CAS.
- V. A. Chebanov, E. A. Muravyova, S. M. Desenko, V. I. Musatov, I. V. Knyazeva, S. V. Shishkina, O. V. Shishkin and C. O. Kappe, J. Comb. Chem., 2006, 8, 427 CrossRef CAS PubMed.
- J. M. Jamison, K. Krabill, A. Hatwalkar, E. Jamison and C. Tsai, Cell Biol. Int. Rep., 1990, 14, 1075 CrossRef CAS PubMed.
- S. M. Menchen, S. C. Benson, J. Y. L. Lam, W. Zhen, D. Sun, B. B. Rosenblum, S. H. Khan and M. Taing, US Pat., US 6583168, 2003transChem. Abstr. 2003, 139, 54287f Search PubMed.
- G. W. Rewcastle, G. J. Atwell, L. Zhuang, B. C. Baguley and W. A. Denny, J. Med. Chem., 1991, 34, 217 CrossRef CAS PubMed.
- R. J. Sarma and J. B. Baruah, Dyes Pigm., 2005, 64, 91 CrossRef CAS.
- J. B. Laursen and J. Nielsen, Chem. Rev., 2004, 104, 1663 CrossRef CAS PubMed.
- C. Neves-Pinto, V. Malta, M. Pinto, R. Santos, S. Castro and A. Pinto, J. Med. Chem., 2002, 45, 740 CrossRef.
- D. Cartwright, W. Chilton and D. Benson, Appl. Microbiol. Biotechnol., 1995, 43, 211 CrossRef CAS.
- M. Muller and T. Sorrell, Prostaglandins, 1995, 50, 301 CrossRef CAS.
- G. A. Kraus and I. A. Kim, J. Org. Chem., 2003, 68, 4517 CrossRef CAS PubMed.
- N. Vicker, L. Burgess, I. S. Chuckowree, R. Dodd, A. J. Folkes, D. J. Hardick, T. C. Hancox, W. Dangerfield, C. Liddle, P. Mistry, A. J. Stewart and W. A. Denny, J. Med. Chem., 2002, 45, 721 CrossRef CAS PubMed.
- Y. F. Qiao, T. Okazaki, T. Ando, K. Mizoue, K. Kondo, T. Eguchi and K. Kakinuma, J. Antibiot., 1998, 51, 282 CrossRef CAS PubMed.
- J. P. Poupelin, G. S. Ruf, O. F. Blanpin, G. Narcisse, G. U. Ernouf and R. Lacroix, Eur. J. Med. Chem., 1978, 13, 67 CAS.
-
(a) J. Altman, E. Babad, J. Itzchaki and D. Ginsburg, Tetrahedron, 1966, 279 CrossRef CAS;
(b) R. W. Weber and J. M. Cook, Can. J. Chem., 1977, 56, 189 CrossRef;
(c) K. B. Wiberg, J. Am. Chem. Soc., 1983, 105, 1227 CrossRef CAS;
(d) A. I. Ioffe, V. A. Svyatkin and O. M. Nefedov, Russ. Chem. Bull., 1988, 37, 1827 CrossRef;
(e) M. Shin, M. H. Kim, T. Ha, J. Jeon, K. H. J. S. Chung Kim and Y. G. Kim, Tetrahedron, 2014, 70, 1617 CrossRef CAS;
(f) D. Kinsberg, Acc. Chem. Res., 1974, 7, 286 CrossRef;
(g) K. B. Wiberg, Chem. Rev., 1989, 89, 975 CrossRef CAS;
(h) J. P. Ainoliisa and M. P. K. Ari, Tetrahedron, 2005, 61, 8769 CrossRef.
-
(a) T. Ibuka, K. Tanaka and Y. Inubushi, Chem. Pharm. Bull., 1974, 22, 782 CrossRef CAS;
(b) H.-Y. Lee, D.-I. Kim and S. Kim, J. Chem. Soc., Chem. Commun., 1996, 1539 RSC;
(c) L. Zu, B. W. Boal and N. K. Garg, J. Am. Chem. Soc., 2011, 133, 8877 CrossRef CAS PubMed;
(d) H. Jian Mei, Y. Ritsuko, Y. Chun-shu and Y. Fukuyama, Tetrahedron Lett., 2000, 41, 6111 CrossRef.
- A. Rezvanian and A. Alizadeh, Tetrahedron, 2012, 68, 10164 CrossRef CAS.
-
(a) K. Weniges, P. Guenther, W. Kasel, G. Hubertus and P. Gunther, Angew. Chem., Int. Ed. Engl., 1981, 20, 960 CrossRef;
(b) J.-M. Huang, C.-S. Yang, M. Tanaka and Y. Fukuyama, Tetrahedron, 2001, 57, 4691 CrossRef CAS;
(c) K. C. Nicolaou, S. A. Snyder, T. Montagnon and G. Vassilikogiannakis, Angew. Chem., Int. Ed., 2002, 41, 1668 CrossRef CAS;
(d) B. M. Trost and Y. Shi, J. Am. Chem. Soc., 1991, 113, 701 CrossRef CAS;
(e) K. Asahi and H. Nishino, Tetrahedron, 2008, 64, 1620 CrossRef CAS.
- A. Alizadeh, A. Rezvanian and L. G. Zhu, J. Org. Chem., 2012, 77, 4385 CrossRef CAS PubMed.
- A. Rezvanian and A. Alizadeh, Tetrahedron, 2012, 68, 10164 CrossRef CAS.
- A. Alizadeh, F. Bayat, L. Moafi and L. G. Zhu, Tetrahedron, 2015, 71, 8150 CrossRef CAS.
- I. Yavari and A. Khajeh-Khezri, Mol. Diversity, 2017, 21, 849 CrossRef CAS PubMed.
- A. A. Hassan, N. K. Mohamed, L. E. Abd El-Haleem, S. Brase and M. Nieger, Curr. Org. Synth., 2016, 13, 426 CrossRef CAS.
- M. Sirouspour and S. Souri, J. Heterocycl. Chem., 2016, 53, 147 CrossRef CAS.
- L. J. Zhang and C. G. Yan, Tetrahedron, 2013, 69, 4915 CrossRef CAS.
- I. Yavari, A. Khajeh-Khezri, S. Bahemmat and M. R. Halvagar, Synlett, 2017, 28, 1785 CrossRef CAS.
- I. Yavari, A. Khajeh-Khezri and M. R. Halvagar, Arabian J. Chem., 2018, 11, 188 CrossRef CAS.
- J. Wang, H. Liu, R. Wen, J. Li and S. Zhu, Chin. J. Chem., 2017, 35, 1463 CrossRef CAS.
- E. Safari, A. Maryamabadi and A. Hasaninejad, RSC Adv., 2017, 7, 39502 RSC.
- M. Beyrati and A. Hasaninejad, Tetrahedron Lett., 2017, 58, 1947 CrossRef CAS.
- A. Maryamabadi, A. Hasaninejad, N. Nowrouzi and G. Mohebbi, Bioorg. Med. Chem., 2017, 25, 2057 CrossRef CAS PubMed.
- M. Beyrati, M. Forutan, A. Hasaninejad, E. Rakovský, S. Babaei, A. Maryamabadi and G. Mohebbi, Tetrahedron, 2017, 73, 5144 CrossRef CAS.
- A. Hasaninejad and M. Beyrati, RSC Adv., 2018, 8, 1934 RSC.
- A. Maryamabadi, A. Hasaninejad, N. Nowrouzi, G. Mohebbi and B. Asghari, Bioorg. Med. Chem., 2016, 24, 1408 CrossRef CAS PubMed.
- M. Beyrati and A. Hasaninejad, Org. Prep. Proced. Int., 2016, 48, 393 CrossRef CAS.
- A. Hasaninejad, N. Golzar, M. Beyrati, A. Zare and M. M. Doroodmand, J. Mol. Catal. A: Chem., 2013, 372, 137 CrossRef CAS.
- A. Hasaninejad and S. Firoozi, Mol. Diversity, 2013, 17, 499 CrossRef CAS PubMed.
- A. Hasaninejad, A. Zare, M. Shekouhy and J. Ameri Rad, Green Chem., 2011, 13, 958 RSC.
- S. Mojikhalifeh and A. Hasaninejad, Tetrahedron Lett., 2017, 58, 2574 CrossRef CAS.
- S. Mojikhalifeh and A. Hasaninejad, Org. Chem. Front., 2018 10.1039/C8QO00210J.
- A. Hasaninejad, S. Mojikhalifeh and M. Beyrati, Appl. Organomet. Chem., 2018 DOI:10.1002/aoc.4380.
Footnote |
† Electronic supplementary information (ESI) available. See DOI: 10.1039/c8ra01648h |
|
This journal is © The Royal Society of Chemistry 2018 |