DOI:
10.1039/C8RA01018H
(Paper)
RSC Adv., 2018,
8, 9334-9343
Controllable thermal and pH responsive behavior of PEG based hydrogels and applications for dye adsorption and release
Received
2nd February 2018
, Accepted 27th February 2018
First published on 5th March 2018
Abstract
A series of controllable thermal and pH dual-responsive copolymeric hydrogels (PMA) were prepared by a one-pot reaction with poly(ethylene glycol) methyl ether acrylate (PEGA), 2-methoxyethyl acrylate (MEA) and acroleic acid (AA). The hydrogels exhibited good mechanical properties and a sensitive response to pH and temperature. Besides, the Lower Critical Solution Temperature (LCST) of the hydrogels can be adjusted from 37 °C to 58 °C by changing the content of AA. The hydrogels also showed excellent selective adsorption properties. The maximum adsorption quantity of organic cationic dye brilliant green and methylene blue were 0.49 mg mg−1 and 0.42 mg mg−1 respectively, much better than previous reports. Furthermore, using the thermal and pH responsibility, the PMA hydrogels can release the adsorbed molecules with control. Nearly 95% of carriers could be released at pH 4.01 and 65 °C over 8 h. The regeneration ability makes the materials easy to reuse many times. Due to these properties, these dual-responsive hydrogels have great potential applications in various fields for adsorption, drug delivery, release and tissue engineering.
Introduction
Polymer hydrogels are cross linked three-dimensional polymeric networks.1–3 They can absorb a great quantity of water and remain mechanically stable without dissolving. In recent years, hydrogels show good performance in developing functional materials such as shape memory polymers,4–7 self-healing materials,8 sensor units,9 super capacitors10 and drug delivery vectors.11–13 Thus, the applications of polymer hydrogels have spread to many fields. One of the most studied forms is stimuli-response hydrogels,14 which can respond to environmental conditions like pH, temperature, magnetic field, light and so on. Among them, the thermal-response property is the most widely studied. Different kinds of temperature responsive materials have been developed. Poly(N-isopropylacrylamide) (PNIPAM) is one of the representatives for the LCST around 32 °C, closing to the human body temperature. Thus, PNIPAM has been introduced to various hydrogels as the unit for offering the temperature responsiveness.15,16 However, most of the PNIPAM composite hydrogels show poor mechanical property, delayed or slow response, which limited their application as functional and biological materials. Hence, it is important to develop alternative structure which could have the excellent thermal-sensitiveness, good mechanical property and reduced hydrogen-bond. In 2012, Liu's group17 reported the poly(2-methoxyethyl acrylate-co-poly(ethylene glycol) methyl ether acrylate) (P(MEA-co-PEGA)) by RAFT dispersion polymerization in water which showed good thermal stimuli-response. They successfully synthesized copolymer hydrogels with narrow range temperature response and linear relationship between LCST and composition through adjusting the contents of monomers, although the mechanical property of such hydrogels was still low. Besides, RAFT requires specify chain transfer agent and initiator, which play important roles in LCST controlling.
In this work, a simple one-pot method was used to prepare a series of dual-responsive hydrogels based on tri-copolymer of PEGA, MEA and AA by conventional free radical polymerization. The polymer hydrogels showed excellent pH-sensitive and temperature-sensitive ability simultaneously. By changing the content of AA, the LCST of the hydrogels can be adjusted from 37 °C to 58 °C. Due to the functional group and structure, the PMA hydrogels showed excellent selective adsorption property. Moreover, by adjusting the pH and temperature, the adsorptions can be released almost completely. These properties ensure the PMA hydrogels have great potential applications in various aspects as adsorption, drug delivery, release and tissue engineering.
Experimental sections
Materials
2-Methoxyethyl acrylate (MEA, 98%), poly(ethylene glycol) methyl ether acrylate (PEGA, Mn = 480), acrylic acid (AA, 98%) were purchased from Sigma-Aldrich. Ammonium persulphate (APS) was purchased from Shanghai-Aladdin.
Synthesis
Poly(2-methoxyethyl acrylate-poly(ethylene glycol) methyl ether acrylate) hydrogels (PM). 1.504 g PEGA was dissolved in 5.186 g water, and then 0.592 g MEA was added. The mixture was stirred for 30 min until completely dissolved. 6% APS was added to the solution and the reaction was carried out at 50 °C for 4 hours under stirring. Then the hydrogel was formed and cooled to the room temperature.
Poly(2-methoxyethyl acrylate-poly(ethylene glycol) methyl ether acrylate-acrylic acid) hydrogels (PMA-1). 1.504 g PEGA was dissolved in water, and then 0.592 g MEA was added. The mixture was stirred for 30 min until completely dissolved. Then, different content of AA and a small amount of APS were added to the solution. The reaction was carried out at 50–60 °C for 6–8 h under stirring. Then the hydrogel was formed and cooled to the room temperature. In this paper, the PMA hydrogels with different content of AA were named as PMA-1 and PMA-2 as shown in Table 1.
Table 1 Different content of PMA hydrogels
Sample |
PEGA (g) |
MEA (g) |
AA (g) |
H2O (g) |
APS (%) |
Time (h) |
PM |
1.504 |
0.592 |
— |
5.186 |
6% |
4 |
PMA-1 |
1.504 |
0.592 |
0.301 |
5.186 |
6% |
6 |
PMA-2 |
1.504 |
0.592 |
0.575 |
5.186 |
6% |
6 |
Characterization
FTIR spectra. The experiments were performed on Nicolet-Nexus 670 spectrophotometer at room temperature. The dried gels were triturated with KBr at the ratio of 1
:
100.
NMR spectra experiments. High resolution solution 13C NMR spectrum was performed on a Varian 700 MHz at room temperature. Solid-state 13C cross-polarization/MAS NMR spectrum were performed at room temperature on Bruker 300 M (spinning rate set as 5 kHz, cross polarization time = 1.0 ms).
Lower critical solution temperature. The experiments were studied by turbidimetry on a Hitachi U-3010 UV-Vis spectrometer. The temperature was detected from 25 °C to 75 °C. The temperature at 50% transmittance of thermal transition was taken as the LCST.
Mechanical property of hydrogels. Hydrogels were prepared as expected shape (15 mm-thick cylindrical molds). The linear ramp force was designed as 1 N min−1 to test the hydrogels. The compressive modulus was calculated by the slope of the stress–strain curve in the linear region.
Swelling property. In order to obtain the hydrogels' swelling properties, the dried hydrogels were immersed into water at 25 °C, till reaching equilibrium of swelling. The swollen hydrogels were weighed after the excess of water on the surfaces absorbed by filter paper. The swelling radio (g g−1) was calculated using eqn (1).Ws and Wd are the weights of the hydrogels at swelling and dried, respectively.
pH responsiveness. The swelling ratio of hydrogels in PBS with pH 4.01, pH 7.4 and pH 9.18 were measured, respectively. The swelling ratio of hydrogels at different pH solutions were measured using the same method as follows: the dried samples were immersed into pH = 4.01 solution to reach equilibrium. Then the swollen hydrogel was transferred into pH = 9.18 solution to reach new equilibrium. The processes were repeated for 5 times and the swelling equilibrium was calculated each time.
Temperature responsiveness. The swelling ratio of hydrogels at different temperature was measured respectively using the same method as follows: the dried samples were immersed into 25 °C aqueous solution to reach equilibrium. Then the swollen hydrogel was transferred into 80 °C aqueous solution to reach new equilibrium. The processes were repeated for 5 times and the swelling equilibrium was calculated each time.
Adsorption
To investigate the adsorption property, 10 mg dried PMA-2 hydrogel was put into organic pigment (brilliant green and methylene blue) solutions with different concentration from 0.0498 mg mL−1 to 1.7778 mg mL−1, at room temperature for 72 h. The concentration of brilliant green and methylene blue in the solution were determined by UV at 623 nm and 664 nm, respectively. The adsorption capacity (Qe) (mg mg−1) was calculated as eqn (2). |
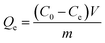 | (2) |
Qe is the adsorption capacity of organic pigment. C0 and Ce are initial and equilibrium concentrations of organic pigment. V is the volume of solution, and m is the weight of the dried hydrogels.
The adsorption kinetics were investigated as follows: 10 mg dried PMA-2 hydrogel was added into 5 mL organic pigment solution (1.7778 mg mL−1) at room temperature and then the samples were taken out at predetermined time ranging from 5 min to 72 h. The adsorption capacity Qt (mg mg−1) was calculated according to eqn (3).
|
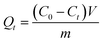 | (3) |
Qt is the adsorption capacity of organic pigment at different time.
Ct and
C0 are initial and equilibrium concentrations of organic pigment, respectively.
V is the volume of solution, and
m is the weight of the dried hydrogels.
Release and regeneration
The release behaviour of hydrogels was studied at different pH and temperature. The PMA-2 hydrogel was put into the brilliant green solution and kept 72 h till adsorption equilibrium. Then the loaded hydrogel was put into 30 mL PBS solutions with different pH (pH = 4.01, pH = 7.4 and pH = 9.81)18 at 25 °C. Periodically, 5 mL of solution was collected and the same volume of fresh PBS solution was added to keep the volume of the solution. The collected solution was detected by UV at 623 nm to measure the concentration of releasing brilliant green. The effect of temperature on release was researched from 25 °C to 80 °C with pH 4.01 PBS solution. The process was repeated to calculate the content of releasing brilliant green.
Regeneration is an attractive property for adsorption materials. To research the regeneration properties, PMA-2 hydrogel was used to adsorb and release brilliant green in pH 4.01 PBS solution at 65 °C. The adsorption and releasing process was repeated 5 times and the concentration was calculated.
All the adsorption and releasing experiments were performed in three repetitions to ensure the accuracy of data and the error bars were shown in the corresponding figures.
Results and discussion
Characterization
A series of tri-copolymer hydrogels was synthesized by one-pot reaction with 2-methoxyethyl acrylate, poly(ethylene glycol) methyl ether acrylate and acroleic acid. The AA was introduced into the system to gain a controllable thermal and pH dual-responsive copolymeric hydrogels. The proposed processes for PMA-1 and PMA-2 were shown in Scheme 1.
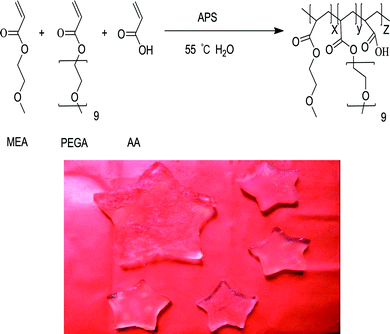 |
| Scheme 1 Schematic produce of hydrogels preparation. | |
The FTIR spectra of AA, MEA, PEGA, PM, PMA-1 and PMA-2 were displayed in Fig. 1. The characteristic peaks of AA were observed, –OH stretching at 3450 cm−1, –C
O stretching at 1700 cm−1 and –C
C stretching at 1600 cm−1. The characteristic peaks of MEA were at 2850 cm−1, 1700 cm−1 and 1100 cm−1, which attributed to the stretching vibrations of –CH2, –C
O and –C–O–C–, respectively. The FTIR spectrum of pure PEGA showed –CH2 stretching at 2800 cm−1, –C
O stretching at 1700 cm−1 and –C–O–C– stretching at 1200 cm−1. In the spectrum of PM, both MEA and PEGA characteristic peaks were observed, which indicating the PM hydrogel was synthesized. In the spectrum of PMA-1, the characteristic –OH peak shifted to 3300 cm−1 and became broader. Due to the polymerization, the –CH2 stretching band at 2800 cm−1 and the –C
C stretching band at 1480 cm−1 disappeared. The peaks at 1700 cm−1 and 1150 cm−1 were –C
O stretching and –C–O–C–, respectively, which proved the PMA-1 hydrogels was obtained. For PMA-2, similar spectrum was observed, which suggested the PMA-2 hydrogel was synthesized successfully.
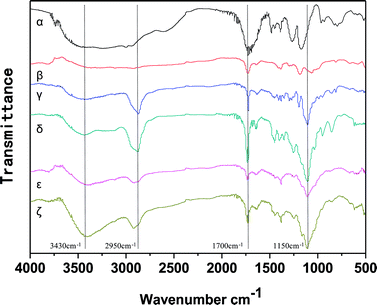 |
| Fig. 1 FTIR spectra of AA (α), MEA (β), PEGA (γ), PM (δ), PMA-1 (ε) and PMA-2 (ζ). | |
The 13C NMR spectra (α-γ) of AA, MEA and PEGA were shown in Fig. 2A. The signals at 175, 136, 128 ppm were assigned to AA (–C
O, C
C). The signals at 168, 133, 128, 60–80 ppm were assigned to MEA (–C
O, C
C, –CH2). The signals at 168, 133, 128, 60–80 ppm were assigned to PEGA (–C
O, C
C, –CH2). In order to quantitative analyse the content of AA, solid-state 13C cross-polarization/MAS single pulse excitation/MAS NMR experiments were performed on freeze-dried hydrogels samples, and the spectra were shown in Fig. 2B (δ–η). The signals of monomer could be distinguished in Fig. 2A. All hydrogels had been washed 3 times to remove the unreacted monomer. With the increase of the content of AA, the integral area of –C
O peaks increased and the –CH3 peaks reduced, which suggested that there were more AA combining with MEA and PEGA. Through titration experiment,19 the contents of AA were obtained as 12% (PMA-1) and 21% (PMA-2). The results are consistent with the 13C CP/MAS NMR results.
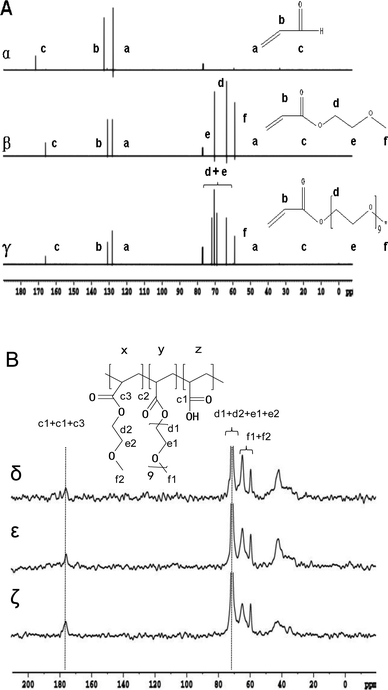 |
| Fig. 2 (A) 13C NMR spectra in CDCl3 (700 MHz) of AA (α), MEA (β), PEGA (γ). (B) 13C CP/MAS NMR spectra (300 MHz) of PM (δ), PMA-1 (ε), and PMA-2 (ζ). | |
The morphology of hydrogels
The morphology of all hydrogels were observed by SEM and shown in Fig. 3. PM hydrogel showed a rough construct and no pore structure (Fig. 3a). With the introducing of AA, PMA hydrogels became porous morphology, as shown in Fig. 3b–c. With the increasing of AA, the porous became denser and the pore size became smaller.
 |
| Fig. 3 The SEM image of hydrogels: PM (A), PMA-1 (B) and PMA-2 (C). All the samples were prepared by saturated swelling and freeze-dried. | |
Mechanical properties
Both PM and PMA hydrogels are transparent and soft. PM hydrogel was relatively brittle and easy to break, while PMA hydrogels showed better elasticity, one can pinch and malaxate it without crumble. To study the mechanical properties of hydrogels, the stress in 50% strain was chosen to evaluate the mechanical properties of the samples. Fig. 4 shows the compressive stress–strain curves of PM, PMA-1 and PMA-2. It can be observed that the stress–strain curves of PM and PMA hydrogels were significant different. The stress in 50% compressive strain of PM hydrogel was only 8.61 kPa, while that of PMA hydrogels ranged from 52.74 kPa to 79.84 kPa, improved at least 6 times. With the increasing of AA content, the stress in 50% strain increased. Due to the weak mechanical properties, the application of PM hydrogel is quite limited, and similar hydrogels were normally used as micro hydrogels.17 With 50–80 kPa elastic modulus, the applications of PMA hydrogels are more extensive, such as adsorption material and drug delivery.20,21
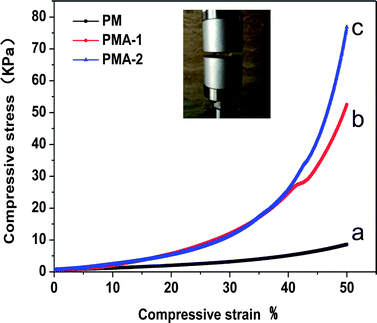 |
| Fig. 4 Compressive stress–strain curve of hydrogels PM (a), PMA-1 (b) and PMA-2 (c). The insetted image was the compressing experiment of PMA-2. | |
Temperature responsiveness
All the PM and PMA hydrogels showed obvious thermal sensitivity. The temperature responsiveness of the samples was shown in Fig. 5, and the parameters were collected in Table 2. In Fig. 5A, the LCST of PM hydrogel was 63 °C. By introducing AA into the copolymer, the LCST decreased to 58 °C for PMA-1. With the increase of AA content, the LCST of PMA-2 decreased to 37 °C. As an example, the images of thermal transitions on PMA-2 were exhibited in Fig. 5B from 35 °C to 45 °C. Through changing the monomers ratio, it provided a convenience way to adjust the temperature responsiveness. For PM hydrogel, it took 8 degrees for transmittance decrease from 100% to 0% (from 60 °C to 68 °C). While for PMA-1, the corresponding temperature range is 55–61 °C which showed shaper responsiveness. Especially for the PMA-2, the temperature range is only 37–40 °C, much less than the PNIPAM hydrogels.22 This character makes them can be used in conditions requiring higher sensitivity.
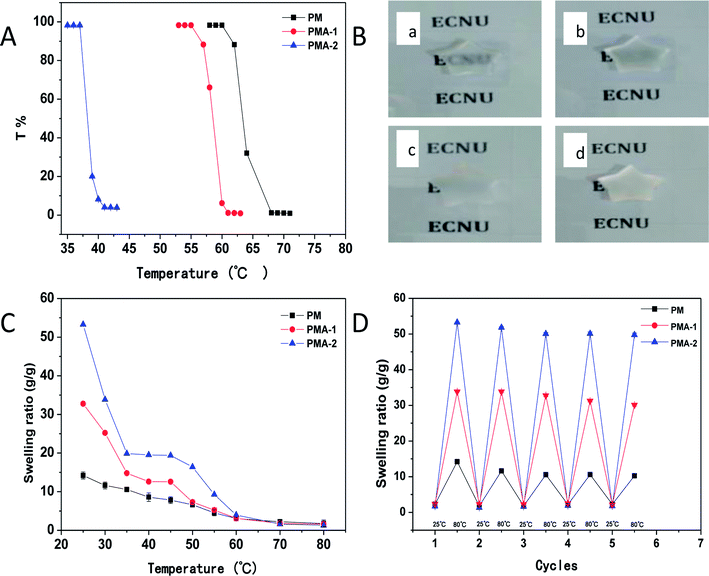 |
| Fig. 5 (A) Temperature response property of hydrogels PM, PMA-1 and PMA-2. (B) The images of hydrogels PMA-2's thermal transitions at 35 °C (a), 37 °C (b), 39 °C (c) and 41 °C (d), respectively. (C) Swelling property of hydrogels PM, PMA-1 and PMA-2 with temperature. (D) Temperature responsiveness property of PM, PMA-1 and PMA-2 with five cycles. | |
Table 2 Properties of PM and PMA samples
Sample |
Stress (kPa) |
LCST (°C) |
SR (g g−1), (pH 4.01) |
SR (g g−1), (pH 7.4) |
SR (g g−1), (pH 9.18) |
PM |
8.61 |
63 |
12.4 |
14.2 |
14.6 |
PMA-1 |
52.47 |
58 |
21.1 |
30.9 |
51.2 |
PMA-2 |
79.84 |
37 |
17.3 |
57.4 |
95.3 |
When the temperature increased through upon hydrogels' LCST, the swelling ratio of PM and PMAs also exhibited corresponding changes (Fig. 5C). For PM hydrogel, with temperature increased from 25 °C to 80 °C, a continuous deswelling was observed. While for PMA hydrogels, different trends were shown. With the temperature increasing, the swelling ratio decreased like a two-step exponential. The swelling ratio reduced quickly at first and then came to a plateau. This is the first step. After the short plateau, the swelling ratio decreased sharply again, and then slowed down until reached another plateau. This is the second step.
As we know, water has two different states in swelling hydrogels. One is freedom water and the other is bonded water, according to the different interactions between polymer chains and water molecules.23,24 When temperature increased, free water was lost easily. Therefore, the swelling ratio decreased sharply. Meanwhile, there was a great amount of bonded water that was not easy to lose, which led to the first plateau. With the temperature increased close to LCST, polymer chains aggregated, interactions between polymer chains and water molecules weakened. It made much of the bonded water transfer to free water.25,26 Thus, with the hydrogel shrinking, those free water molecules were extruded from hydrogel quickly. The swelling ratio decreased sharply again. After that, only strongly bonded water was left in the hydrogel, which presented as the final plateau at the curves.
Regeneration experiments were also performed to study the application of hydrogels. The hydrogels were heated to 80 °C then cooled to 25 °C. This process was repeated 5 times and the swelling ratio was measured each time. The results were shown in Fig. 5D. After five cycles, the swelling–deswelling properties were maintained more than 90% and kept stable. These results indicated that PMA hydrogels are good reusable materials.
pH responsiveness of hydrogels
As our anticipation, by introducing the AA content, the hydrogels may gain pH responsiveness. Due to the hydrophilic content and protonation of carboxyl group, the volume of hydrogels could extend or shrink following the solution pH value. The SEM images of PMA-2 hydrogels at different pH solution were shown in Fig. 6a–c were SEM images of PAM-2 hydrogels with same original volume and shape treated by pH 4.01, pH 7.4 and pH 9.18 PBS at 25 °C, respectively. At lower pH, the acid solutions caused the dissociation of ionic bonds because of protonation of carboxyl group. At higher pH, due to ionization and hydration, the carboxyl group were COO– forms at an alkaline solution.27
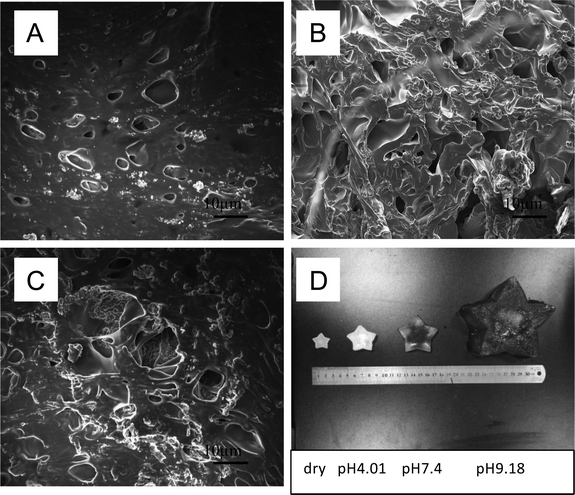 |
| Fig. 6 The SEM images of PMA-2 hydrogels in pH = 4.01 solution (A), pH = 7.4 solution (B) and pH = 9.18 solution (C) at 25 °C by saturated swelling and then freeze dried. (D) The images of PMA-2 hydrogel after swelling in PBS with different pH. | |
The relationship between pH and swelling ratio of hydrogels was studied. The equilibrium swelling ratio of dried PM, PMA-1 and PMA-2 hydrogels were measured in pH = 4.01, pH = 7.4 and pH = 9.18 solutions at 25 °C and collected in Table 2.
Fig. 7 showed the swelling behaviours of PM and PMA hydrogels. In Fig. 7A, all samples showed low swelling ratio in acidic condition. In neutral and alkali conditions, the swelling ratio of PMA hydrogels highly increased (see Fig. 7B and C), while PM hydrogel remained constant. All the samples remained their shape integrally (see Fig. 7D).
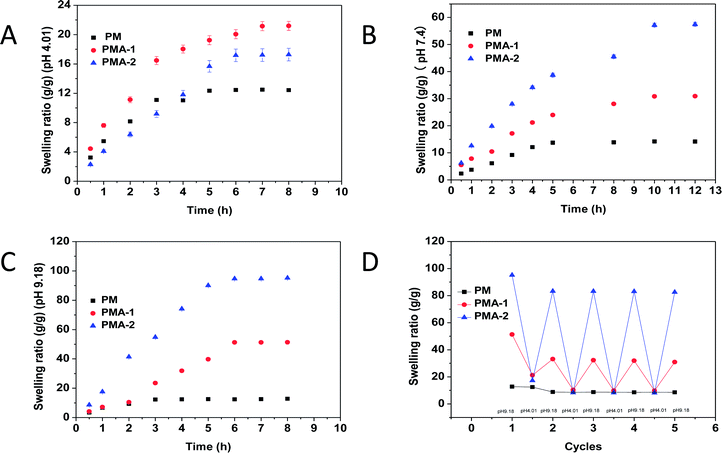 |
| Fig. 7 Swelling property of hydrogels PM, PMA-1 and PMA-2 in pH = 4.01 solution (A), pH = 7.4 solution (B) and pH = 9.18 solution (C). (D) pH responsiveness property of PM, PMA-1 and PMA-2 with five cycles. | |
In neutral condition, the swelling ratios of PMA-1 and PMA-2 were 30.9 and 57.4 g g−1, respectively, much more than that of PM (14.2). Both PEGA and MEA were not strong hydrophilic molecules. When strong hydrophilic carboxyl group was introduced into the copolymer, the hydrophilic ability of the whole system improved greatly and produced a tight and ionic bonds structure, introducing a large number of water-binding sites. Hence, the swelling ratio was enhanced.
To evaluate the regeneration ability, the hydrogels were alternately put into pH = 9.18 and pH = 4.01 PBS solutions repeatedly and measured the swelling ratio for 5 times. The results were shown in Fig. 7D. After five cycles, the swelling–deswelling property was still more than 90% and kept stable. This result indicated that PMA hydrogels were stable and reusable materials.
Adsorption
Due to the carboxyl groups, the hydrogels are also good special adsorption materials for cationic dyes. The adsorption behaviour of brilliant green was investigated at 25 °C. The adsorption kinetic curves of brilliant green were shown in Fig. 8. Both PMAs and PM samples showed adsorption ability. All PMA samples showed better adsorption ability than PM sample. For the PMA, the adsorption amount was increased with the increasing brilliant green. Through the adsorption equation, the equilibrium adsorption ability of PMA-2 was 0.492 ± 0.008 mg mg−1, much more than that of PM 0.051 ± 0.003 mg mg−1. It was also much higher than previous reports (around 0.135 mg mg−1).28
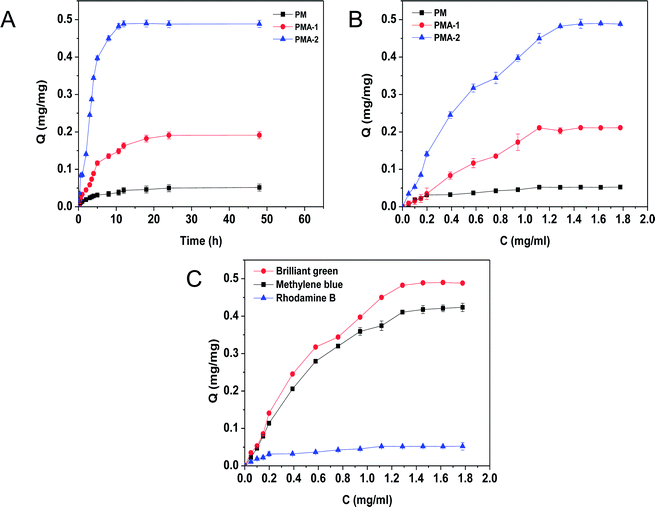 |
| Fig. 8 (A) Adsorption kinetic of brilliant green on PM, PMA-1 and PMA-2. (B) Static adsorption isotherms of brilliant green on PM, PMA-1 and PMA-2. (C) Static adsorption isotherms of brilliant green, methylene blue and rhodamine B on PMA-2. | |
The outstanding adsorption property of PMA hydrogels were attributed to the negative charged carboxyl group which can combine with the positive charged amino-group. And the porous structure could provide channels for dye molecules freely moving into the hydrogels.29,30 With the increasing of AA content, the adsorption capacity for brilliant green was improved from PMA-1 to PMA-2. It was attributed to the increasing combination sites by AA.
As the adsorption kinetic curves of PMA-2 and PM were shown in Fig. 9a. In the first 10 h, the PMA-2 exhibited rapidly adsorption of brilliant green and reached 93% of the equilibrium adsorption. During the next 5 h, the adsorption amount increased slowly and reached adsorption equilibrium. Compared with PMA-2, the adsorption kinetic of PM reached equilibrium slowly. The rapidly adsorption ability was attribute to the porous structure and the strong interaction between carboxyl group and amino-group. When dried hydrogels were put into the brilliant green solution, through the swelling process, brilliant green easily diffused into PMA and be caught by carboxyl group. The porous structure of hydrogel improved the dynamics rate.
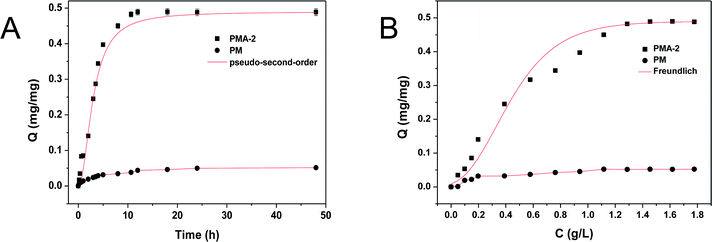 |
| Fig. 9 (A) Static adsorption isotherms for the adsorption process of brilliant green by PMA-2. (B) Adsorption kinetics for the adsorption process of brilliant green by PMA-2. | |
To further study the adsorption kinetics of brilliant green on PMA-2, the adsorption rate was fitted by kinetic adsorption rate equation as eqn (4) and (5) and the kinetic parameters were listed in Table 3.
|
 | (5) |
Qe (mg mg
−1) is equilibrium.
Qt (mg mg
−1) is the amount of brilliant green adsorbed on PMA-2 at time
t.
k1 is the rate constant of pseudo-first-order model.
k2 is the rate constant of pseudo-second-order model.
Table 3 Kinetics data for the pseudo-first-order and pseudo-second-order rate equation at 25 °C
|
Qe (mg mg−1) |
Pseudo-first-order |
Pseudo-second-order |
Qe1 (mg mg−1) |
k1 (h−1) |
R2 |
Qe2 (mg mg−1) |
k2 (h−1) |
R2 |
PMA-2 |
0.492 ± 0.008 |
0.489 |
0.0187 |
0.948 |
0.491 |
0.0085 |
0.991 |
PM |
0.051 ± 0.003 |
0.049 |
0.0013 |
0.937 |
0.048 |
0.0011 |
0.985 |
Compared with pseudo-first-order, the adsorption process of PMA-2 was in accord with the characteristics of the pseudo-second-order. It indicated that the adsorption process could be a chemical adsorption process with a fast adsorption beginning.
Fig. 9b was the isothermal adsorption curve for brilliant green on PMA and PM. Compared Langmuir and Freundlich adsorption equilibrium as following eqn (6) and (7), the results of Langmuir and Freundlich adsorption were shown in Table 4.
|
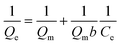 | (6) |
|
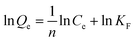 | (7) |
Ce is the equilibrium concentration of brilliant green in solution (mg mL
−1).
Qm is the maximum adsorption capacity of the adsorbent (mg mg
−1).
b is the Langmuir adsorption equilibrium constant (mL mg
−1).
KF and 1/
n are the Freundlich characteristic constants.
Table 4 Langmuir and Freundlich data for the adsorption of brilliant green on the PMA-2 at 25 °C
Adsorption |
Langmuir |
Freundlich |
Qm (mg mg−1) |
b |
R2 |
1/n |
KF |
R2 |
PMA-2 |
0.494 ± 0.006 |
0.865 |
0.964 |
0.253 |
0.324 |
0.994 |
The correlation coefficient R2 of Langmuir and Freundlich were close to 1, which suggested that the adsorption process on PMA-2 was the interaction of physical adsorption and chemical adsorption.31 It indicated that the adsorption was not a monolayer adsorption and there was strong interaction between the brilliant green and PMA-2. The adsorption intensity (1/n)32 of Freundlich was the adsorption intensity and surface heterogeneity. The 1/n was 0.253. It suggested that the brilliant green could be adsorbed easily by PMA-2.
We also studied the adsorption ability of PMA-2 hydrogel on methylene blue with amino-group and rhodamine B with carboxyl group (see Fig. 8C). Compared with 0.42 mg mg−1 of methylene blue,33,34 the adsorption of rhodamine B was quite low, which attributed to the weak interaction between PMA and rhodamine B. The excellent adsorption ability indicated that PMA hydrogels had a wide use for selective adsorption of cationic organic dyestuff.
Release and reutilization
For the organic dyestuff adsorption materials, the desorption and regeneration were attractive properties. Due to the stimuli-responsive properties of the PMA hydrogels, we assumed that the adsorbed dye molecules may be released under fitful conditions. Thus, the release behaviour was studied and the results were shown in Fig. 10. Using brilliant green@PMA-2 as an example, the releasing properties at different pH and different temperature were studied. From Fig. 10A we could see that the releasing capacity at pH 4.01 was more than that at pH 7.4 and pH 9.18 at room temperature. At pH 4.01, almost 80% of brilliant green was released within 50 h at room temperature. While only 18% and 30% were released at pH 7.4 and pH 9.18, respectively. It was because the brilliant green located on the surface could release quickly into the solution in the first 10 h. According to the pH swelling experiments, the lower pH could improve the deswelling and lower the electrostatic attraction between PMA-2 and brilliant green, which drove more brilliant green molecules to release from hydrogels. Therefore, the brilliant green could be controlled release from hydrogels by pH.
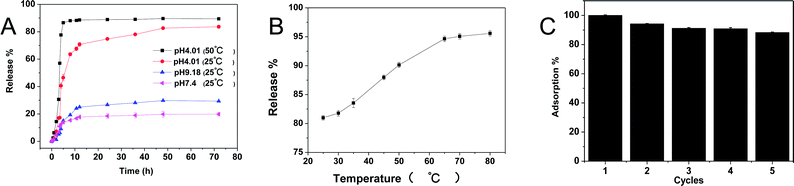 |
| Fig. 10 (A) Time dependence of SBC release from PMA-2 hydrogels in PBS solution (pH = 4.01, 7.4, 9.18) at different temperature. (B) Effect of temperature on release. (C) The regeneration of PMA-2 on brilliant green during five cycles (pH 4.01 PBS solution and 50 °C). | |
In consideration of thermal sensitive, the release behaviour at different temperature was also researched. In Fig. 10B, it could be found the elevated temperature improved the released content from 80% to 95% at pH 4.01. As we know, the PMA hydrogels have LCST, above this temperature, polymer chains aggregate and the hydrogels shrink. Moreover, increasing temperature also accelerated the molecular motion and reduced the releasing time. For example, at 50 °C (pH 4.01), it took only 8 h to release 90% brilliant green, while at 25 °C, it took 50 h to release 80%. (Fig. 10A). Hence, after adsorption of the dyestuff, the PMA hydrogels could be easily reused by desorption.
As we can see from Fig. 10B, the releasing rates are almost the same at temperature higher than 65 °C, thus we choose pH 4.01 and 65 °C as desorption conditions. Then, the adsorption–desorption behavior of brilliant green on PMA-2 was studied. Adsorption was performed at neutral condition room temperature. As shown in Fig. 10C, after five cycles, the adsorption ability remained 90% of the original and kept stable. These results indicated that the PMA-2 hydrogels had good regeneration.
Conclusions
In this work, a serious of dual-responsive copolymeric PMA hydrogels were successfully synthesized. The PMA hydrogels not only provided intelligent temperature and pH responsiveness but also had good mechanical properties. With the stimulation of pH and temperature, the hydrogels showed the excellent repeatable swelling and deswelling properties. The LCST of hydrogels was controllable (from 37 °C to 58 °C) and the mechanical property was quite good (79.84 kPa in 50% compressive strain). Because of the carboxyl group, the hydrogels were applied in adsorbing cationic organic dyes like brilliant green (0.49 mg mg−1) and methylene blue (0.42 mg mg−1). The PMA-2 hydrogel also could easily release nearly 95% brilliant green at pH 4.01 and 65 °C during 8 h. Moreover, it also had good regeneration ability and kept 90% adsorption property after five cycles. In a word, PMA hydrogels are good adsorption materials with high capacity, controllable releasing and good reusability. Besides, with these features, these PMA hydrogels would have wild potential as multifunctional materials in different areas.
Conflicts of interest
There are no conflicts to declare.
Acknowledgements
This study was supported by National Natural Science Foundation of China (No. 51273067, 21544003)
Notes and references
- Y. Tang, Y. Du, X. Hu, X. Shi and J. F. Kennedy, Carbohydr. Polym., 2007, 4, 491–499 CrossRef.
- Y. Luo, Y. Guo, X. Gao, B. Li and T. Xie, Adv. Mater., 2013, 5, 743–748 CrossRef PubMed.
- A. Sannino, C. Demitri and M. Madaghiele, Materials, 2009, 2, 353 CrossRef CAS.
- M. Behl, M. Y. Razzaq and A. Lendlein, Adv. Mater., 2010, 31, 3388–3410 CrossRef PubMed.
- I. Bellin, S. Kelch and A. Lendlein, J. Mater. Chem., 2007, 28, 2885–2891 RSC.
- Y. Wu, J. Hu, C. Zhang, J. Han, Y. Wang and B. Kumar, J. Mater. Chem. A, 2015, 1, 97–100 Search PubMed.
- Q. Zhao, H. J. Qi and T. Xie, Prog. Polym. Sci., 2015, 49, 79–120 CrossRef.
- H. Jia, Z. Huang, Z. Fei, P. J. Dyson, Z. Zheng and X. Wang, ACS Appl. Mater. Interfaces, 2016, 45, 31339–31347 Search PubMed.
- C. Ohm, M. Brehmer and R. Zentel, Adv. Mater., 2010, 31, 3366–3387 CrossRef PubMed.
- K. Shi, Z. Liu, Y. Wei, W. Wang, X. J. Ju, R. Xie and L. Y. Chu, ACS Appl. Mater. Interfaces, 2015, 49, 27289–27298 Search PubMed.
- L. Zha, B. Banik and F. Alexis, Soft Matter, 2011, 13, 5908–5916 RSC.
- P. S. K. Murthy, M. Y. Murali, K. Varaprasad, B. Sreedhar and R. K. Mohana, J. Colloid Interface Sci., 2008, 2, 217–224 CrossRef PubMed.
- X. Zhang, Y. Yang and T. Chung, J. Colloid Interface Sci., 2002, 1, 105–111 CrossRef PubMed.
- Y. Xiao, X. Gong, Y. Kang, Z. Jiang and S. Zhang, Chem. Commun., 2016, 70, 10609–10612 RSC.
- H. Zhang, Y. Zhang, L. He, B. Yang, S. Zhu and M. Yao, Colloid Polym. Sci., 2016, 12, 1959–1967 Search PubMed.
- Z. M. O. Rzaev, S. Dinçer and E. Pişkin, Prog. Polym. Sci., 2007, 5, 534–595 CrossRef.
- G. Liu, Q. Qiu and Z. An, Polym. Chem., 2012, 2, 504–513 RSC.
- H. L. Huang, X. H. Wang, H. Ge and M. Xu, ACS Sustainable Chem. Eng., 2016, 6, 3334–3343 CrossRef.
- H. Ge, H. L. Huang, M. Xu and Q. Chen, Cellulose, 2016, 4, 2527–2537 CrossRef.
- N. S. Johari, I. Ahmad and N. Halib, Chem. Biochem. Eng. Q., 2012, 26, 399–404 CAS.
- K. Haraguchi and T. Takehisa, Adv. Mater., 2002, 16, 1120–1124 CrossRef.
- X. Feng, K. Zhang, P. Chen and X. Sui, Macromol. Rapid Commun., 2016, 13, 1939–1944 CrossRef PubMed.
- W. B. Li, F. Xue and R. S. Cheng, Acta Polym. Sin., 2006, 5, 671–675 Search PubMed.
- G. X. Tan and Y. D. Cui, Polym. Mater. Sci. Eng., 2005, 2, 253–256 Search PubMed.
- Y. Zhao, H. Su, L. Fang and T. Tan, Polymer, 2005, 14, 5368–5376 CrossRef.
- G. R. Mahdavinia, A. Pourjavadi, H. Hosseinzadeh and M. J. Zohuriaan, Eur. Polym. J., 2004, 7, 1399–1407 CrossRef.
- R. R. Haikal, A. M. Elmansi, P. Ali, Y. S. Hassan and M. H. Alkordi, RSC Adv., 2016, 48, 42307–42312 RSC.
- L. Y. Kong, F. X. Qiu, Z. Y. Zhao, X. Y. Zhang, T. Zhang, J. M. Pan and D. Y. Yang, J. Cleaner Prod., 2016, 137, 51–59 CrossRef CAS.
- M. P. Tavlieva, S. D. Genieva, V. G. Georgieva and L. T. Vlaev, J. Colloid Interface Sci., 2013, 409, 112–122 CrossRef CAS PubMed.
- M. S. U. Rehman, M. Munir, M. Ashfaq, M. Rashid, M. F. Nazar, M. Danish and J. I. Han, Chem. Eng. J., 2013, 228, 54–6231 CrossRef CAS.
- L. Xie, J. Guo, Y. Zhang and S. Shi, J. Agric. Food Chem., 2014, 62, 8221–8228 CrossRef CAS PubMed.
- M. Zhang, R. Wang, Z. Shi, X. Huang, W. Zhao and C. Zhao, J. Hazard. Mater., 2017, 322, 499–507 CrossRef CAS PubMed.
- R. Han, J. Zhang, P. Han, Y. Wang, Z. Zhao and M. Tang, Chem. Eng. J., 2009, 3, 496–504 CrossRef.
- D. Kavitha and C. Namasivayam, Bioresour. Technol., 2007, 1, 14–21 CrossRef PubMed.
|
This journal is © The Royal Society of Chemistry 2018 |
Click here to see how this site uses Cookies. View our privacy policy here.