DOI:
10.1039/C7RA12100H
(Paper)
RSC Adv., 2018,
8, 5058-5062
Transition-metal-free regioselective C–H halogenation of imidazo[1,2-a]pyridines: sodium chlorite/bromite as the halogen source†
Received
3rd November 2017
, Accepted 23rd January 2018
First published on 1st February 2018
Abstract
A facile transition-metal-free regioselective halogenation of imidazo[1,2-a]pyridines using sodium chlorite/bromite as the halogen source is presented. The reaction has provided an efficient method for the formation of C–Cl or C–Br bonds to synthesize 3-chloro or 3-bromo-imidazo[1,2-a]pyridines which were then efficiently transformed into imidazo[1,2-a]pyridine core π-systems by Suzuki–Miyaura reactions.
Aryl halides as a structural skeleton are present in a large number of natural products, pharmaceuticals, and biologically active compounds.1 Significant drugs such as Aripiprazole, Chlortrimeton, Plavix and Zoloft all included the aryl chlorides motif (Scheme 1). Apart from this, aryl bromides have always been extremely important synthetic intermediates and building blocks in organic chemistry2 for the construction of diverse and highly functionalized compounds. In the past few years, aryl halides have been used as substrates to form carbon–carbon and carbon–heteroatom bonds via transition-metal-catalyzed cross-coupling reactions. There are some classical methods: Heck, Suzuki, Negishi, Stille, Sonogashira, Ullmann, Buchwald–Hartwig, Kumada, etc.3–10 Consequently, the development of a new route for the construction of these scaffolds is highly desirable, especially those based on assembling structures directly from readily available raw materials. In the last few years, several transition-metal-catalyzed halogenation transformations11,12 have been developed, employing Pd, Rh or Cu as the catalysts and carboxylic acid, amide, nitrile, or pyridine as the directing groups (Scheme 1a). Recently, NH4X, NaX and HX have been also employed as the halogen sources13 in transition-metal-free conditions for the halogenation of several arenes and heteroarenes (Scheme 1b). Nevertheless, directing groups and additional oxidants were usually needed in these transformations and the halogen sources were very limited.
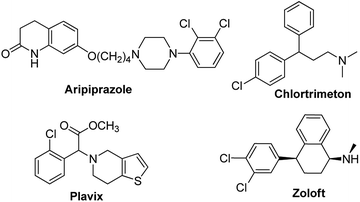 |
| Scheme 1 Chlorine containing drugs. | |
In order to expand the richness of green synthetic methods, we tried to hunt for other atom-economical and easy-to-obtain halogen sources. As we know, sodium chlorite or bromite are commodity chemicals that are widely used as main effective components of bleaches or desizing agents.14 On the other hand, imidazo[1,2-a]pyridines represent an important class of molecules and show unique bioactivities and chemical properties15 that lead them to broad applications in organic synthesis and pharmaceutical chemistry.16 Recent significant advances17 have been achieved in this field. We have also developed novel strategies for the construction of imidazo[1,2-a]pyridines.18 Herein, our current interest is focused on developing an efficient transition-metal-free selective halogenation of imidazo[1,2-a]pyridines without direct group, in which process sodium chlorite or bromite were used as both halogen sources and oxidants (Scheme 2c).
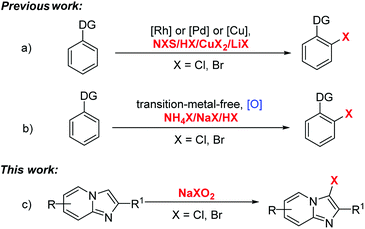 |
| Scheme 2 Selective halogenation reactions. | |
Our initial investigation focused on the halogenation of imidazo[1,2-a]pyridine 1a. The results of the optimized reaction conditions are summarized in Table 1. The reaction was conducted in the presence of NaClO2 (1 equiv.), AcOH (2 mmol), in toluene at 60 °C for 10 h (Table 1, entry 1). To our delight, the desired product 2a was formed in 64% yield. No regioisomeric products were observed by GC-MS and 1H NMR spectroscopy. Being delighted by this result, we then carried out the different optimization experiments to obtain the optimal reaction conditions. Various amounts of NaClO2 were next survey for this transformation (Table 1, entries 2–3). A similar yield was obtained after increasing the amount of NaClO2 to 3 equiv., while a decreased yield was observed after decreasing the amount from 2 to 1 equiv. Among the set of additives examined, AcOH gave the desired product 2a in good yield, while CF3COOH, PivOH and TsOH were found to be less effective in affording the corresponding product 2a in 29–40% yield (Table 1, entries 4–6). It was worth noting that if acid was not added, only trace amount of target product was generated (Table 1, entry 7). The effect of solvents was further tested. It was found that DMF was the best choice in comparison to toluene, 1,4-dioxane, NMP, CH3CN, DMSO and DCE (Table 1, entries 8–13). We then screened the reaction temperature and found the reaction performed at 40 °C or 80 °C gave a lower yield of the product 2a (Table 1, entries 14–15). The control experiment indicated that product 2a was not generated in the absence of NaClO2 (Table 1, entry 16).
Table 1 Optimization of the reaction conditionsa
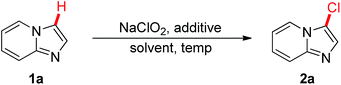
|
Entry |
NaClO2 (equiv.) |
Additive |
Solvent |
Temp (°C) |
Yieldb (%) |
Reaction conditions: 1a (0.5 mmol), NaClO2 (1–3 mmol), AcOH (2 mmol), solvent (2 mL), 40–80 °C for 10 h. Determined by GC analysis. Without NaClO2. |
1 |
2 |
AcOH |
Toluene |
60 |
64 |
2 |
3 |
AcOH |
Toluene |
60 |
62 |
3 |
1 |
AcOH |
Toluene |
60 |
43 |
4 |
2 |
CF3COOH |
Toluene |
60 |
40 |
5 |
2 |
PivOH |
Toluene |
60 |
29 |
6 |
2 |
TsOH |
Toluene |
60 |
31 |
7 |
2 |
— |
Toluene |
60 |
Trace |
8 |
2 |
AcOH |
Dioxane |
60 |
69 |
9 |
2 |
AcOH |
NMP |
60 |
45 |
10 |
2 |
AcOH |
CH3CN |
60 |
37 |
11 |
2 |
AcOH |
DMSO |
60 |
63 |
12 |
2 |
AcOH |
DMF |
60 |
87 |
13 |
2 |
AcOH |
DCE |
60 |
14 |
14 |
2 |
AcOH |
DMF |
40 |
74 |
15 |
2 |
AcOH |
DMF |
80 |
85 |
16c |
2 |
AcOH |
DMF |
60 |
n.r. |
With the establishment of the optimal conditions, the scope of this transition-metal-free chlorination reaction was next investigated. And the results have been described in Scheme 3. A variety of 2-unsubstituted imidazo[1,2-a]pyridines were first employed under the optimized conditions. Different position substituted groups on the pyridine ring of imidazo[1,2-a]pyridine, having 6-CH3, 6-Cl, 6-I, 7-CH3, 8-CH3 substitution, were well-tolerated under the optimized conditions. The results indicated that selective C-3 chlorination products 2a–2f were formed in good to excellent yields. This catalytic system was further found to be successfully applied to catalyze the chlorination of 2-CH3, 2-C(CH3)3, and 2-Ph substituted imidazo[1,2-a]pyridines, generating the desired products in moderate to good yields (2g–2p). It was worth noting that when imidazo[1,2-a]pyridines substituted with sterically hindered 2-C(CH3)3 were employed as substrates, the transformation worked well and led to a beneficial effect on the reaction outcome.
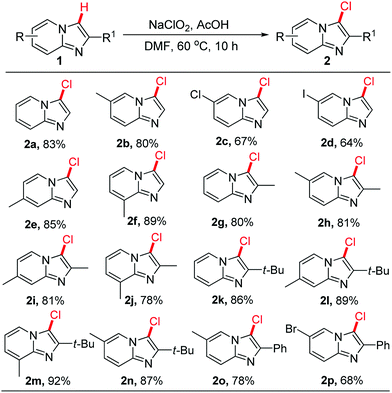 |
| Scheme 3 Chlorination of imidazo[1,2-a]pyridines. | |
We next examined the bromination of imidazo[1,2-a]pyridines derivatives in the presence of NaBrO2 and AcOH in DMF at 60 °C for 10 h. The results were summarized in Scheme 4. As we expected, the optimal conditions could also be applied to bromination of imidazo[1,2-a]pyridines and afforded the brominated products 3a–3f in 70–88% yields. It was found that the reaction was also with great regioselective in the case of 2-unsubstituted imidazo[1,2-a]pyridines.
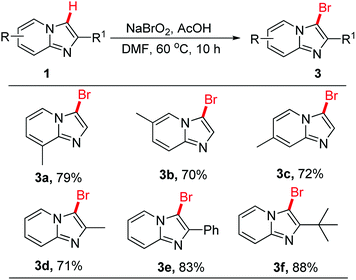 |
| Scheme 4 Bromination of imidazo[1,2-a]pyridines. | |
The reactions of 2a or 3d with phenylboronic acid were conducted in the presence of Pd-catalyst (Scheme 5). The Suzuki–Miyaura reactions were performed very well, affording the product 5a or 6a in 74% or 79% yields, respectively.
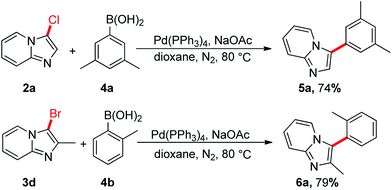 |
| Scheme 5 Suzuki–Miyaura reactions of 2a or 3d with phenylboronic acid. | |
In order to text whether this method is compatible with other aromatic species or not, we have tried to use indoles, 1-methyl-1H-indoles, benzofurans, N,N-dimethylaniline, N-phenylacetamide and 1,3,5-trimethoxybenzene to perform under the present reaction conditions, while no target products were obtained. We supposed that the specificity of this reported method is because of the rich electronic ethene-1,2-diamine moiety of imidazo[1,2-a]pyridine (Scheme 6).
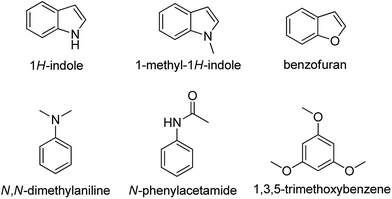 |
| Scheme 6 Applications for other aromatic species. | |
Gaining insight into the mechanism, control experiments were carried out for this transition-metal-free halogenation reaction. To prove a radical species involved in transformation, the reactions were conducted by adding radical-trapping reagent (TEMPO) or radical inhibitor (BHT) (Scheme 7, eqn (1)) in the reaction, and only trace amount of product 2a was observed. The result clearly showed that this reaction had been inhibited and a radical process was involved for this transformation, which was consistent with previous reported.17 To further investigated the chlorine source, NaClO and NaClO3 were employed to react with imidazo[1,2-a]pyridine 2a under the standard conditions. The results showed that the chlorination products were obtained in yields of 47% or 63% respectively, which means chlorine ions having a charge of 1+ or 5+ can also proceed this transformation, albeit with low yields (Scheme 7, eqn (2)).
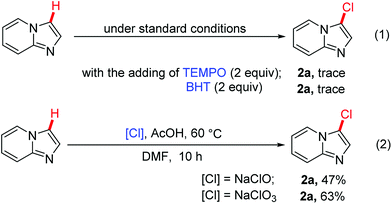 |
| Scheme 7 Control experiments for investigation of the mechanism. | |
Base on the above results and previous works,19 a possible mechanism was proposed to account for this transition-metal-free regioselective halogenation reaction (Scheme 8). Firstly, oxidation–reduction reaction of sodium chlorite happened in the presence of AcOH to produce chlorine, NaOAc and H2O. Subsequently, the chlorine radical was easily formed via homolysis of chlorine, which then attack the double bond between C2 and C3 of imidazo[1,2-a]pyridine, resulting in free radical intermediate I (more stable than II because of the p–π conjugation). Finally, the free radical intermediate I underwent an aromatization with chlorine free radical to give the target product 2a.
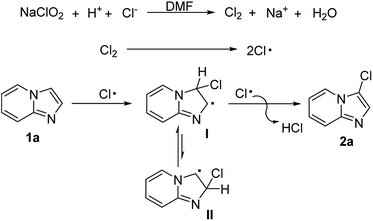 |
| Scheme 8 Possible mechanism. | |
Conclusions
In conclusion, we have developed an efficient transition-metal-free regioselective C–H functionalization approach. The reaction provides a new selective route to synthesize 3-Cl or 3-Br-imidazo[1,2-a]pyridines without directing groups. The use of cheap sodium chlorite or bromite as both halogenic sources and oxidants is a major advantage. The 3-Cl or 3-Br-imidazo[1,2-a]pyridines as the substrates can successful apply to Suzuki–Miyaura reactions.
Conflicts of interest
There are no conflicts to declare.
Acknowledgements
This work was supported by the Innovation and Strong School Project of Guangdong Pharmaceutical University (2015cxqx212), and the Project of Innovation for Enhancing Guangdong Pharmaceutical University, Provincial Experimental Teaching Demonstration Center of Chemistry & Chemical Engineering.
Notes and references
-
(a) D. G. Fujimori and C. T. Walsh, Curr. Opin. Chem. Biol., 2007, 11, 553–560 CrossRef CAS PubMed;
(b) A. Butler and J. V. Walker, Chem. Rev., 1993, 93, 1937–1944 CrossRef CAS.
-
(a) K. C. Nicolaou, P. G. Bulger and D. Sarlah, Angew. Chem., Int. Ed., 2005, 44, 4442–4489 CrossRef CAS PubMed;
(b) L. Rout, T. K. Sen and T. Punniyamurthy, Angew. Chem., Int. Ed., 2007, 46, 5583–5586 CrossRef CAS PubMed.
-
(a) I. P. Beletskaya and A. V. Cheprakov, Chem. Rev., 2000, 100, 3009–3066 CrossRef CAS PubMed;
(b) J. L. Bras and J. Muzart, Chem. Rev., 2011, 111, 1170–1214 CrossRef PubMed.
-
(a) T. Kawashima, T. Takao and H. Suzuki, J. Am. Chem. Soc., 2007, 129, 11006–11007 CrossRef CAS PubMed;
(b) T. Kawakami and H. Suzuki, Tetrahedron Lett., 2000, 41, 7093–7096 CrossRef CAS;
(c) A. R. Katritzky, K. Suzuki, S. K. Singh and H. Y. He, J. Org. Chem., 2003, 68, 5720–5723 CrossRef CAS PubMed.
-
(a) E. Negishi and L. Anastasia, Chem. Rev., 2003, 103, 1979–2018 CrossRef CAS PubMed;
(b) E.-i. Negishi, Z. Huang, G. Wang, S. Mohan, C. Wang and H. Hattori, Acc. Chem. Res., 2008, 41, 1474–1485 CrossRef CAS PubMed.
-
(a) J. K. Stille, Angew. Chem., Int. Ed., 1986, 25, 508–524 CrossRef;
(b) V. Farina, V. Krishnamurthy and W. J. Scott, Org. React., 1998, 50, 1–652 Search PubMed.
-
(a) R. Chinchilla and C. Nájera, Chem. Soc. Rev., 2011, 40, 5084–5121 RSC;
(b) R. Chinchilla and C. Nájera, Chem. Rev., 2007, 107, 874–922 CrossRef CAS PubMed.
- J. Hassan, M. Sevignon, C. Gozzi, E. Schulz and M. Lemaire, Chem. Rev., 2002, 102, 1359–1470 CrossRef CAS PubMed.
-
(a) D. S. Surry and S. L. Buchwald, Chem. Sci., 2011, 2, 27–50 RSC;
(b) J. F. Hartwig, Acc. Chem. Res., 1998, 31, 852–860 CrossRef CAS;
(c) J. P. Wolfe, S. Wagaw, J. F. Marcoux and S. L. Buchwald, Acc. Chem. Res., 1998, 31, 805–818 CrossRef CAS.
- M. S. Eno, A. Lu and J. P. Morken, J. Am. Chem. Soc., 2016, 138, 7824–7827 CrossRef CAS PubMed.
- Selected examples:
(a) F. Kakiuchi, T. Kochi, H. Mutsutani, N. Kobayashi, S. Urano, M. Sato, S. Nishiyama and T. Tanabe, J. Am. Chem. Soc., 2009, 131, 11310–11311 CrossRef CAS PubMed;
(b) P. Sadhu, S. K. Alla and T. Punniyamurthy, J. Org. Chem., 2013, 78, 6104–6111 CrossRef CAS PubMed;
(c) B. Du, X. Jiang and P. Sun, J. Org. Chem., 2013, 78, 2786–2791 CrossRef CAS PubMed;
(d) T. S. Mei, R. Giri, N. Maugel and J. Q. Yu, Angew. Chem., Int. Ed., 2008, 47, 5215–5219 CrossRef CAS PubMed;
(e) B. R. Song, X. J. Zheng, J. Mo and B. Xu, Adv. Synth. Catal., 2010, 352, 329–335 CrossRef CAS;
(f) R. B. Bedford, M. F. Haddow, C. J. Mitchell and R. L. Webster, Angew. Chem., Int. Ed., 2011, 50, 5524–5528 CrossRef CAS PubMed;
(g) R. Das and M. Kapur, J. Org. Chem., 2017, 82, 1114–1126 CrossRef CAS PubMed;
(h) M. Dabiri, N. F. Lehi, S. Kazemi Movahed and H. R. Khavasi, Org. Biomol. Chem., 2017, 15, 6264–6268 RSC;
(i) C. Testa, É. Gigot, S. Genc, R. Decréau, J. Roger and J.-C. Hierso, Angew. Chem., Int. Ed., 2016, 55, 5555–5559 CrossRef CAS PubMed.
- Selected examples:
(a) X. Zhou, C. Yu, Z. Feng, Y. Yu, J. Wang, E. Hao, Y. Wei, X. Mu and L. Jiao, Org. Lett., 2015, 17, 4632–4635 CrossRef CAS PubMed;
(b) P. P. Singh, T. Thatikonda, K. A. A. Kumar, S. D. Sawant, B. Singh, A. K. Sharma, P. R. Sharma, D. Singh and R. A. Vishwakarma, J. Org. Chem., 2012, 77, 5823–5828 CrossRef CAS PubMed;
(c) G. Qian, X. Hong, B. Liu, H. Mao and B. Xu, Org. Lett., 2014, 16, 5294–5297 CrossRef CAS PubMed;
(d) J. Wang, W. Wang and J. H. Li, Green Chem., 2010, 12, 2124–2126 RSC;
(e) N. Schroder, J. Wencel-Delord and F. Glorius, J. Am. Chem. Soc., 2012, 134, 8298–8301 CrossRef PubMed;
(f) S. Mo, Y. Zhu and Z. Shen, Org. Biomol. Chem., 2013, 11, 2756–2760 RSC;
(g) J. Xu, X. Zhu, G. Zhou, B. Ying, P. Ye, L. Su, C. Shen and P. Zhang, Org. Biomol. Chem., 2016, 14, 3016–3021 RSC;
(h) P. Zhang, L. Hong, G. Li and R. Wang, Adv. Synth. Catal., 2015, 357, 345–349 CrossRef CAS.
-
(a) S. Song, X. Sun, X. Li, Y. Yuan and N. Jiao, Org. Lett., 2015, 17, 2886–2889 CrossRef CAS PubMed;
(b) L. Gu, T. Lu, M. Zhang, L. Tou and Y. Zhang, Adv. Synth. Catal., 2013, 355, 1077–1082 CrossRef CAS;
(c) G.-W. Wang and J. Gao, Green Chem., 2012, 14, 1125–1131 RSC;
(d) Y. Wang, Y. Wang, K. Jiang, Q. Zhang and D. Li, Org. Biomol. Chem., 2016, 14, 10180–10184 RSC.
- S. Adimurthy, S. Ghosh, P. U. Patoliya, G. Ramachandraiah, M. Agrawal, M. R. Gandhi, S. C. Upadhyay, P. K. Ghosh and B. C. Ranu, Green Chem., 2008, 10, 232–237 RSC.
-
(a) G. Puerstinger, J. Paeshuyse, E. Declercq and J. Neyts, Bioorg. Med. Chem. Lett., 2007, 17, 390–393 CrossRef CAS PubMed;
(b) M. A. Ismail, R. K. Arafa, T. Wenzler, R. Brun, F. A. Tanious, W. D. Wilson and D. W. Boykin, Bioorg. Med. Chem., 2008, 16, 681–685 Search PubMed.
-
(a) S. Husinec, R. Markovic, M. Petkovic, V. Nasufovic and V. Savic, Org. Lett., 2011, 13, 2286–2289 CrossRef CAS PubMed;
(b) H. W. Huang, X. C. Ji, X. D. Tang, M. Zhang, X. W. Li and H. F. Jiang, Org. Lett., 2013, 15, 6254–6257 CrossRef CAS PubMed;
(c) Y. Gao, M. Z. Yin, W. Q. Wu, H. W. Huang and H. F. Jiang, Adv. Synth. Catal., 2013, 355, 2263–2273 CrossRef CAS.
-
(a) K. Pericherla, P. Khedar, B. Khungara and A. Kumar, Chem. Commun., 2013, 49, 2924–2926 RSC;
(b) H. Wang, Y. Wang, C. Peng, J. Zhang and Q. Zhu, J. Am. Chem. Soc., 2010, 132, 13217–13219 CrossRef CAS PubMed;
(c) L. Albrecht, A. Albrecht, L. K. Ransborg and K. A. Jørgensen, Chem. Sci., 2011, 2, 1273–1277 RSC;
(d) A. K. Bagdi, S. Santra, K. Monir and A. Hajra, Chem. Commun., 2015, 51, 1555–1575 RSC;
(e) A. K. Bagdi, M. Rahman, S. Santra, A. Majee and A. Hajra, Adv. Synth. Catal., 2013, 355, 1741–1747 CrossRef CAS;
(f) S. Santra, A. K. Bagdi, A. Majee and A. Hajra, Adv. Synth. Catal., 2013, 355, 1065–1070 CrossRef CAS;
(g) J. Zeng, Y. J. Tan, M. L. Leow and X. W. Liu, Org. Lett., 2012, 14, 4386–4389 CrossRef CAS PubMed;
(h) H. Wang, Y. Wang, D. Liang, L. Liu, J. Zhang and Q. Zhu, Angew. Chem., Int. Ed., 2011, 50, 5678–5681 CrossRef CAS PubMed;
(i) K. S. Masters, T. R. M. Rauws, A. K. Yasav, W. A. Herrebout, B. V. Veken and B. U. W. Mases, Chem.–Eur. J., 2011, 17, 6315–6320 CrossRef CAS PubMed;
(j) A. J. Stasyuk, M. Banasiewicz, M. K. Cyrański and D. T. Gryko, J. Org. Chem., 2012, 77, 5552–5558 CrossRef CAS PubMed;
(k) P. R. Adiyala, G. S. Mani, J. B. Nanubolu, K. C. Shekar and R. A. Maurya, Org. Lett., 2015, 17, 4308–4311 CrossRef CAS PubMed;
(l) J. Liu, W. Wei, T. Zhao, X. Liu, J. Wu, W. Yu and J. Chang, J. Org. Chem., 2016, 81, 9326–9336 CrossRef CAS PubMed;
(m) C. Rao, S. Mai and Q. Song, Org. Lett., 2017, 19, 4726–4729 CrossRef CAS PubMed.
-
(a) H. Cao, L. Chen, J. Liu, H. Cai, H. Deng, G. Chen, C. Yan and Y. Chen, RSC Adv., 2015, 5, 22356–22360 RSC;
(b) H. Cao, S. Lei, N. Li, L. Chen, J. Liu, H. Cai, S. Qiu and J. Tan, Chem. Commun., 2015, 51, 1823–1825 RSC;
(c) S. Lei, G. Chen, Y. Mai, L. Chen, H. Cai, J. Tan and H. Cao, Adv. Synth. Catal., 2016, 358, 67–73 CrossRef CAS;
(d) S. Lei, Y. Mai, C. Yan, J. Mao and H. Cao, Org. Lett., 2016, 18, 3582–3585 CrossRef CAS PubMed;
(e) H. Zhan, L. Zhao, J. Liao, N. Li, Q. Chen, S. Qiu and H. Cao, Adv. Synth. Catal., 2015, 357, 46–50 CrossRef CAS.
-
(a) X. Chen, X.-S. Hao, C. E. Goodhue and J.-Q. Yu, J. Am. Chem. Soc., 2006, 128, 6790–6791 CrossRef CAS PubMed;
(b) J.-B. Xia and S.-L. You, Org. Lett., 2009, 11, 1187–1190 CrossRef CAS PubMed.
Footnote |
† Electronic supplementary information (ESI) available. See DOI: 10.1039/c7ra12100h |
|
This journal is © The Royal Society of Chemistry 2018 |
Click here to see how this site uses Cookies. View our privacy policy here.