DOI:
10.1039/C7RA11259A
(Paper)
RSC Adv., 2018,
8, 170-175
A efficient protocol for the synthesis of thioamides in [DBUH][OAc] at room temperature†
Received
12th October 2017
, Accepted 8th December 2017
First published on 20th December 2017
Abstract
A novel, simple and eco-friendly method to synthesize thioamides from aryl nitriles and sodium sulfide (Na2S·9H2O) catalyzed by 1,8-diazabicyclo[5,4,0]undec-7-enium acetate ([DBUH][OAc]) ionic liquid (IL) at room temperature was developed in this paper. In this reaction, readily available inorganic salt (Na2S·9H2O) serves as the sulfur source, and various functional groups of aryl nitriles were well tolerated at room temperature. In addition, the products were easily separated from the IL which could be reused at least five times without considerable loss of its activity and applied in the green, concise synthesis of ethionamide.
Introduction
Thioamides and their derivatives are useful synthons and reaction partners in organic chemistry, and often found in vital biological and pharmaceutical molecules.1 Meanwhile, as important building blocks, they are applied broadly in the areas of synthesis of many significant sulfur-containing heterocycles, such as thiazolines, thiazoles, thiazolinones, thiadiazoles, tetrazoles, mesoionic rhodanine, betaines and other heterocyclic.2–10 Therefore, the synthesis of thioamides which have received extensive attention. Consequently, a number of synthesis methods have been developed for the thioamides using various reagents under diverse reaction conditions.11–18 Conventionally, alternative sulfur sources such as Lawesson's reagent and its analogues are applied to the synthesis of thioamides (Scheme 1a).19–21 Similarly, sulfur–phosphorus-type reagents have been developed for the synthesis of thioamides through nitriles or amides and carboxylic acids (Scheme 1a).22–31 However, these traditional methods often require harsh reaction conditions and the yields are typically low, such as high temperature, the use of toxic and high boiling solvents, and moreover, suffer from a narrow substrate scope. Following the guidance of green chemistry, find an environment-friendly way to synthesize thioamides is considered of high practical value.
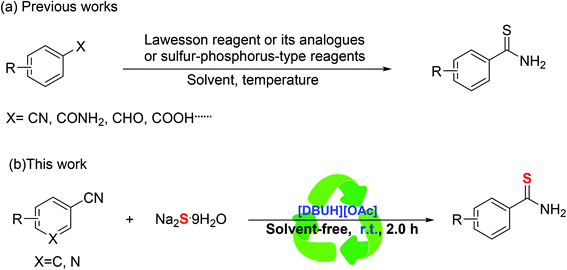 |
| Scheme 1 | |
Using ionic liquid as a solvent for organic reactions is a very interesting and attractive topic because it is both convenient and environmentally friendly. Ionic liquids becoming an effective medium in organic synthesis. In view of this, herein, we would like to report a simple green method for the synthesis of the thioamides based on the novel recyclable ionic liquid [DBUH][OAc] via reaction of cheap, easily available sodium sulfide (Na2S·9H2O) and nitriles at room temperature (Scheme 1b).
Result and discussion
Initially, we initiated the optimization of the reaction on simple benzonitrile (1a) (Table 1). Reaction of Benzonitrile 1a with S8 in the presence of bases (entries 1–7) in different solvents at room temperature, there are no the desired thiobenzamide 2a product generated. Similarly, when 1a with S8 in [DBUH][OAc] at room temperature also no reaction (entry 8). Treatment of benzonitrile with other S source, carbon disulfide (CS2), in [DBUH][OAc] at room temperature provided the desired thiobenzamide 2a in 31% yield (entry 1). When the same reaction was carried out at a higher temperature (40 °C), the yield (42%) was not improved significantly. Use of Na2S·9H2O as S source in solvents (entries 11–16) at room temperature, the product thiobenzamide 2a were not found. Use of Na2S·9H2O in the presence of [DBUH][OAc] was able to give he desired product (entry 19) in 88% yield; however, Na2S·9H2O in the presence of solvents provided thiobenzamide 2a in lower yield (entries 17 and 18). In order to examine the activity of different ionic liquids benzonitrile 1a was caused to react with Na2S·9H2O in the presence of each ionic liquid separately, as shown in Table 1 (entries 19–21), [DBUH][OAc] is the most effective in terms of yield of the corresponding thioamide (88%), the results show that the activities of the ILs depend on both their anions and cations, The [DBUH][OAc] and [BMim][OAc] with an Ac− anion can promote for the reaction, the major reason was that acetic acid is a weak acid and so the ILs are bases, and basic catalysis can promote the reaction. Meanwhile, the cations of the ILs also influenced reaction significantly, the excellent activity of the [DBUH][OAc] may result from their optimal basicity for the reaction.32 Treatment of benzonitrile 1a with Na2S in [DBUH][OAc] at room temperature for 2 h gave the desired thiobenzamide 2a product in a very trace amount, however, when no water was added, it furnished thiobenzamide 2a in 82% yield (entry 23), the result show that water is necessary. In general, the optimum conditions were 2 h at room temperature in the presence of [DBUH][OAc] for this reaction.
Table 1 Optimization of the reaction conditions
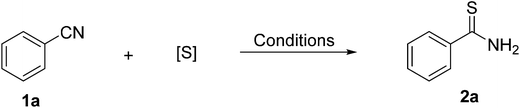
|
Entry |
S source |
Additive |
Solvent |
Yielde (%) |
Reaction conditions: 1a 1.0 mmol, S8 3.0 mmol, additive 3.0 mmol, solvent 0.6 mL, r.t., 2 h. Reaction conditions: 1a 1.0 mmol, S8 3.0 mmol, additive 3.0 mmol, r.t., 2 h. Reaction conditions: 1a 1.0 mmol, Na2S·9H2O 1.2 mmol, solvent 1 mL, r.t., 2 h. Reaction conditions: 1a 1.0 mmol, S source 1.2 mmol, additive 3.0 mmol, solvent 0.3 mL. Isolated yield. The reaction was run at 40 °C. |
1a |
S8 |
Na2CO3 |
H2O |
0 |
2a |
S8 |
K2CO3 |
H2O |
0 |
3a |
S8 |
KOtBu |
H2O |
0 |
4a |
S8 |
DBU |
H2O |
0 |
5a |
S8 |
DBU |
AcOH |
0 |
6a |
S8 |
DBU |
DMF |
0 |
7a |
S8 |
DBU |
DMSO |
0 |
8b |
S8 |
[DBUH][OAc] |
— |
0 |
9c |
CS2 |
[DBUH][OAc] |
— |
31 |
10c,f |
CS2 |
[DBUH][OAc] |
— |
42 |
11c |
Na2S·9H2O |
— |
H2O |
0 |
12c |
Na2S·9H2O |
— |
DMSO |
0 |
13c |
Na2S·9H2O |
— |
DMF |
0 |
14c |
Na2S·9H2O |
— |
NMP |
0 |
15c |
Na2S·9H2O |
— |
DMAC |
0 |
16c |
Na2S·9H2O |
— |
PEG-400 |
0 |
17d |
Na2S·9H2O |
[DBUH][OAc] |
H2O |
44 |
18d |
Na2S·9H2O |
[DBUH][OAc] |
DMF |
78 |
19d |
Na2S·9H2O |
[DBUH][OAc] |
— |
88 |
20d |
Na2S·9H2O |
[BMIm][OAc] |
— |
38 |
21d |
Na2S·9H2O |
[BMIm][PF6] |
— |
Trace |
22d |
Na2S |
[DBUH][OAc] |
— |
Trace |
23d |
Na2S |
[DBUH][OAc] |
H2O |
82° |
In order to expand the scope of this system, various aromatic or heterocyclic nitriles were then examined (Table 2). The experimental results disclosed that the substrates bearing electron donating or electron withdrawing groups underwent this reaction to produce thioamides in moderate to high yields (70–91%). The substrate bearing the electron-donating group CH3 reacted smoothly with yields of up to 88% (Table 2, 2b, 2c). The substrate bearing the electron-withdrawing group CN and CF3 also reacted well with yields of up to 75% (Table 2, 2l, 2m). The coupling of heteroaryl nitriles with Na2S·9H2O also performed well (Table 2, 2t, 2u, 2v and 2w). Letrozole is an orally active nonsteroidal aromatase inhibitor for the treatment of hormonally-responsive breast cancer after surgery, and it has two “CN” functional groups, the product 2y (64%) was obtained when 1.2 equiv. of Na2S·9H2O was used. However, when the amount of Na2S·9H2O was increased to 2.4 equiv., the product 2z was obtained with 73% yield. In general, it can be concluded from the results that the IL ([DBUH][OAc]) had broad applicability to substrates in this reaction.
Table 2 Substrate scope for the thioamidesa,b
Reaction conditions: 1 (1 mmol), Na2S·9H2O (1.2 mmol), [DBUH][OAc] (3 mmol), r.t. 2 h. Isolated yield. Yield of the product (runs: 2). Yield of the product (runs: 3). Yield of the product (runs: 4). Yield of the product (runs: 5). Reaction conditions: 1 (0.5 mmol), Na2S·9H2O (0.6 mmol). Reaction conditions: 1 (0.5 mmol), Na2S·9H2O (1.2 mmol). |
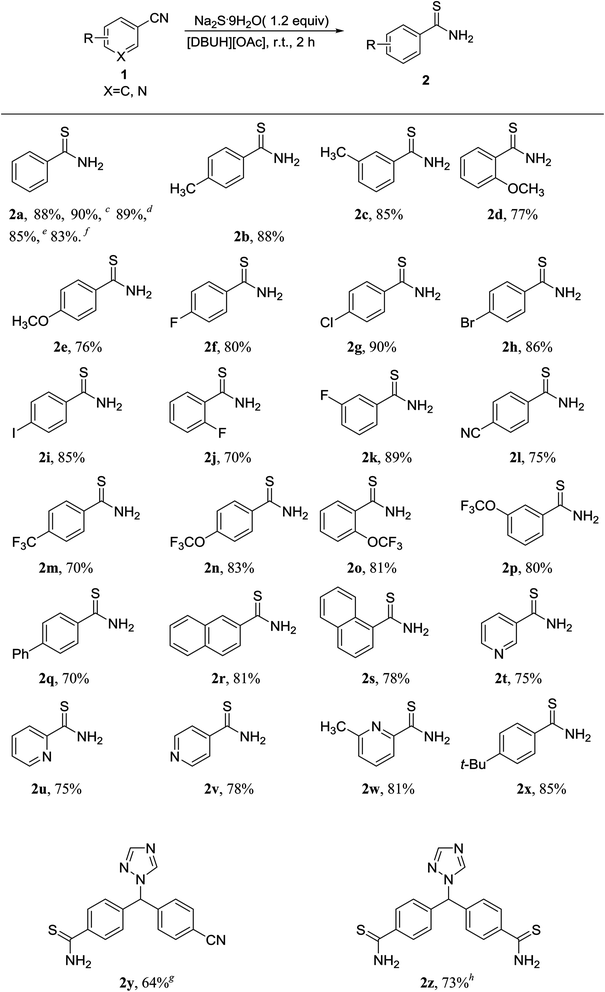 |
Significantly, the IL ([DBUH][OAc]) used in this reaction was found to remain catalytic ability after being three times. Yet the product yields only decline slightly (reducing from 88% in the first run to 83% in the five run). This result indicated that this system was recyclable for catalyzing the reaction of Na2S·9H2O with aryl nitriles.
So as to embody the application value of the method, we performed a gram-scale reaction (Scheme 2), the product 2a was easily obtained in 79% yield under the [DBUH][OAc] ionic-liquid at room temperature.
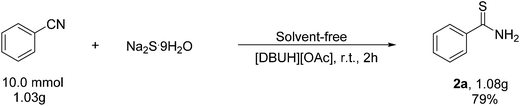 |
| Scheme 2 A gram-scale reaction. | |
With the effective method of thioamides in hand, we next inspected the applicability of this method to prepare ethionamide which is an antibiotic used to treat tuberculosis.
Fortunately, the synthesis of compound ethionamide was successful proceed under optimum conditions, and the target product was acquired in 80% yield (Scheme 4).
Importantly, the products were easily afford pure thioamides. The present reaction provides a simple and straightforward access from readily available materials (nitriles, and Na2S·9H2O) to thioamides, which permits rapid access to various important compounds. For instance, the 1,2,4-thiadiazole 3a was isolated in 95% yield (Scheme 3a),33 Moreover, styrene with NBS and subsequent addition of thiobenzamide 2a in water provided 2,4-diarylsubstituted thiazole 4a in 72% yield (Scheme 3b).34 Furthermore, thiobenzamide 2a with iodobenzene to provide 5a in 57% yield, (Scheme 3c).35
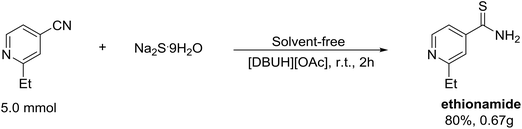 |
| Scheme 3 Application of the reaction. | |
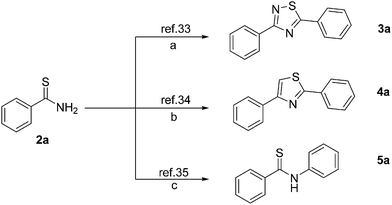 |
| Scheme 4 Applications to thioamides. | |
Reaction mechanism
A plausible pathway was outlined in Scheme 5. The [DBUH]+ made the cyanide activated, the S2− with the nitrile to yield intermediate A. Finally, the final product thioamides are obtained from the intermediate A.
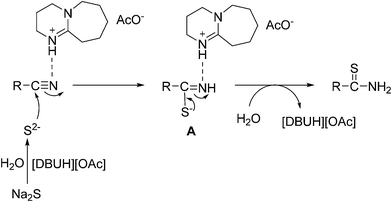 |
| Scheme 5 The possible reaction pathway. | |
Conclusion
In summary, we have developed an efficient [DBUH][OAc]-promoted the reaction of different aryl nitriles with Na2S·9H2O as an inexpensive, conveniently available and easy-to-handle sulfide surrogate under solvent-free conditions at room temperature. The generated products were easily separated from the ILs which could be reclaimed at least five times. The operational environmental friendliness and simplicity of this method highlights its potential application in organic synthesis and pharmaceutical industry.
Experimental
General procedure for preparation of ionic liquid [DBUH][OAc]: to a 250 mL three-necked flask was added 40 mmol of DBU. Acetic acid (40 mmol) was then added slowly dropwise in ice bath. After dropwise addition, the ice bath was removed and the reaction mixture was stirred at room temperature for 24 h. The oil residue was dried in vacuo at 60 °C for 24 h to afford [DBU][OAc] as a light yellow, viscous liquid. The characteristic data is accord with the literature.36
The general procedure of preparing all products is similar. Taking the model reaction as an example: benzonitrile 1a (1 mmol) and [DBUH][OAc] (3 mmol) were added into a 10 mL bottle. After 2.0 h, by adding about 4 mL H2O after the reaction to disperse the solid product, the reaction mixture was extracted with ethyl acetate (3 × 4 mL) followed by H2O (5 mL), and the mixture was purified by column chromatography.
Conflicts of interest
There are no conflicts to declare.
Acknowledgements
We are grateful to the Major scientific and technological innovation projects of Hangzhou City (No. 20162011A036) and the Program for Changjiang Scholars and Innovative Research Team in Chinese University (IRT 1231) for providing financial support.
References
- F. Wang, R. Langley, G. Gulten, L. G. Dover, G. S. Besra, W. R. Jacobs Jr and J. C. Sacchettini, J. Exp. Med., 2007, 204, 73–78 CrossRef CAS PubMed.
- T. S. Jagodzinski, Chem. Rev., 2003, 103, 197–228 CrossRef CAS PubMed.
- P. Wipf and S. A. Venkatraman, J. Org. Chem., 1996, 61, 8004–8005 CrossRef CAS PubMed.
- D. Kumar, N. M. Kumar, K. H. Chang, R. Gupta and K. Shah, Bioorg. Med. Chem. Lett., 2011, 21, 5897–5900 CrossRef CAS PubMed.
- L. M. T. Frija, A. J. L. Pombeiro and M. N. Kopylovich, Eur. J. Org. Chem., 2017, 2017, 2670–2682 CrossRef CAS.
- O. A. Attanasi, S. Berretta, L. D. Crescentini, G. Favi, P. Filippone, G. Giorgi, S. Lillini and F. Mantellini, Tetrahedron Lett., 2007, 48, 2449–2451 CrossRef CAS.
- A. S. Gurjar, V. Andrisano, A. D. Simone and V. S. Velingkar, Bioorg. Chem., 2014, 57, 90–98 CrossRef CAS PubMed.
- G. L. Perlovich, A. N. Proshin, T. V. Volkova, L. N. Petrova and S. O. Bachurin, Mol. Pharmaceutics, 2012, 9, 2156–2167 CrossRef CAS PubMed.
- A. S. Mayhoub, L. Marler, T. P. Kondratyuk, E. J. Park, J. M. Pezzuto and M. Cushman, Bioorg. Med. Chem., 2012, 20, 510–520 CrossRef CAS PubMed.
- Y. Sun, W. Wu and H. Jiang, Eur. J. Org. Chem., 2014, 2014, 4239–4243 CrossRef CAS.
- H. Xu, H. Deng, Z. Li, H. Xiang and X. Zhou, Eur. J. Org. Chem., 2013, 7054–7057 CrossRef CAS.
- K. A. Mahammed, V. P. Jayashankar, N. Premsai Rai, K. Mohana Raju and P. N. Arunachalam, Synlett, 2009, 14, 2338–2340 Search PubMed.
- M. L. Boys and V. L. Downs, Synth. Commun., 2006, 36, 295–298 CrossRef CAS.
- T. Kanbara, K. Okamoto and T. Yamamoto, Synlett, 2007, 2007, 2687–2690 CrossRef.
- C. K. Khatri, A. S. Mali and G. U. Chaturbhuj, Monatsh. Chem., 2017, 148, 1463–1468 CrossRef CAS.
- A. Manaka and M. Sato, Synth. Commun., 2005, 35, 761–764 CrossRef CAS.
- S. Ray, A. Bhaumik, A. Dutta, R. J. Butcher and C. Mukhopadhyay, Tetrahedron Lett., 2013, 54, 2164–2170 CrossRef CAS.
- Z. Yin and B. Zheng, J. Sulfur Chem., 2013, 34, 527–531 CrossRef CAS.
- Z. Kaleta, G. Tárkányi, A. Görmory, F. Kálmán, T. Nagy and T. Soós, Org. Lett., 2006, 8, 1093–1095 CrossRef CAS PubMed.
- U. Pathak, L. Pandey, S. Mathur and M. Suryanarayana, Synthesis, 2011, 44, 377–379 CrossRef.
- C.-H. Yang, G.-J. Li, C.-J. Gong and Y.-M. Li, Tetrahedron, 2015, 71, 637–642 CrossRef CAS.
- T. J. Curphe, J. Org. Chem., 2002, 67, 6461–6473 CrossRef.
- J. Bergman, B. Pettersson, V. Hasimbegovic and P. H. Svensson, J. Org. Chem., 2011, 76, 1546–1553 CrossRef CAS PubMed.
- D. Cho, J. Ahn, K. A. De Castro, H. Ahn and H. Rhee, Tetrahedron, 2010, 66, 5583–5588 CrossRef.
- L. Doszczak and J. Rachon, Chem. Commun., 2000, 2093–2094 RSC.
- S. Goswami, A. C. Maity and N. K. Das, J. Sulfur Chem., 2007, 28, 233–237 CrossRef CAS.
- B. Kaboudin and D. Elhamifar, Synthesis, 2006, 224–226 CrossRef CAS.
- B. Kaboudin and L. Malekzadeh, Synlett, 2011, 2011, 2807–2810 CrossRef.
- B. Kaboudin, V. Yarahmadi, J.-y. Kato and T. Yokomatsu, RSC Adv., 2013, 3, 6435 RSC.
- H. R. Lagiakos, A. Walker, M.-I. Aguilar and P. Perlmutter, Tetrahedron Lett., 2011, 52, 5131–5132 CAS.
- A. K. Yadav, V. P. Srivastava and L. D. S. Yadav, Synth. Commun., 2013, 44, 408–416 CrossRef.
- W. Lu, J. Ma, J. Hu, J. Song, Z. Zhang, G. Yang and B. Han, Green Chem., 2014, 16, 221–225 RSC.
- M. H. Shinde and U. A. Kshirsagar, Green Chem., 2016, 18, 1455–1458 RSC.
- K. Swapna, S. N. Murthy and Y. V. D. Nageswar, Eur. J. Org. Chem., 2010, 2010, 6678–6684 CrossRef.
- G. Vanajatha and V. P. Reddy, Tetrahedron Lett., 2016, 57, 2356–2359 CrossRef CAS.
- A. Ying, L. Liu, G. Wu, G. Chen, X. Chen and W. Ye, Tetrahedron Lett., 2009, 50, 1653–1657 CrossRef CAS.
Footnote |
† Electronic supplementary information (ESI) available. See DOI: 10.1039/c7ra11259a |
|
This journal is © The Royal Society of Chemistry 2018 |
Click here to see how this site uses Cookies. View our privacy policy here.