DOI:
10.1039/C8QM00013A
(Chemistry Frontiers)
Mater. Chem. Front., 2018,
2, 830-834
The renaissance of nitric oxide: from improvement of stability to enhancement of endocytosis
Received
12th February 2018
, Accepted 9th March 2018
First published on 12th March 2018
Abstract
In this perspective article, we have reviewed the exploration of nitric oxide (NO) over the past 40 years and tried to catch a glimpse of the possible future direction of NO research. A lot of astounding achievements have already been made, from the discovery of the physiological mechanism of NO to the fabrication of a feasible NO delivery system. However, a large portion of NO action sites are inside cells, and indiscriminate distribution of NO donors would undoubtedly bring about a mismatch between the NO release and action sites. These mispairings not only reduce the efficacy of NO applications, but also cause undesirable side effects. Based on these arguments, we propose that the local generation and intracellular release of NO is no less vital than improving the stability of the carriers. Potential solutions to this problem have also been put forward. We hope our views and concepts will prove helpful to inspire new ideas for NO applications.

Fan Jia
| Fan Jia received his Bachelor degree from Zhejiang University. He is currently a PhD candidate in Professor Ji's group. His research is focused on synthesizing and fabricating novel NO releasing materials, and exploring their potential use in cardiovascular modulation, tumor inhibition and anti-microbial applications. |

Yongyan Deng
| Yongyan Deng received her Bachelor degree from Zhejiang University. She is currently a PhD candidate in Professor Ji's group. Her research is focused on employing NO and other molecules to modulate the micro-environment of tumors to improve the outcome of cancer therapy. |
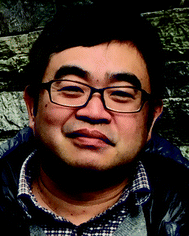
Jian Ji
| Prof. Ji Jian is the director of the Institute of Biomedical Macromolecules at Zhejiang University. He received the Distinguished Young Scholars Award of the National Science Foundation of China in 2010, Cheung Kong Scholars by the Ministry of Education in 2015, and became a Fellow of the Royal Society of Chemistry in 2016. His research is focused on interfacial phenomena for biomedical implants, tissue engineering and nanomedicine. Based on biomimetic strategies, several supramolecular self-assembly methods have been explored to develop biocompatible and biofunctional surfaces for biomedical applications. Several innovative techniques have been applied to biomedical devices including cardiovascular stents, catheters, etc. |
Nitric oxide (NO), a molecule that is never far from the limelight in the biomedical field, is considered to be both old and new. It is old because the medical application of its derivatives has a long history of more than 150 years.1 It is new because the veil that covers its mysterious effects has only begun to be lifted in the past 40 years.2 Since the 1980s, the rediscovery of NO has renewed our understanding of the immune response,3 neuronal signalling4 and vascular regulation.5 These advances in revealing underlying mechanisms marked the dawn of a new era in NO research, which led to the famed title of “Molecule of the Year” in 19926 and finally the 1998 Nobel Prize in Physiology or Medicine.7
As a simple diatomic molecule, NO has proven to be quite ‘mischievous’ and can readily undergo various kinds of reaction with diverse compounds, demonstrating its surprisingly active nature. Such a restless character originates from its unique molecular electron structure. Inside each NO molecule, the nitrogen and oxygen atoms are bonded together by an sp2 σ-bond and a π-bond, with lone pair electrons on both atoms and an unpaired electron from the N atom. These features mean that NO is both an active radical species and an excellent coordinating ligand, with a mild oxidation ability and a strong reductive property.8 Its versatility singles NO out as an important multifunctional biomolecule with a key role in many physiological and pathological processes (Fig. 1), including cardiovascular homeostasis,9 immune modulation,10 tissue repair11 and tumor inhibition.12
 |
| Fig. 1 Nitric oxide participates in various physiological and pathological processes, including, but not limited to, tumor inhibition, immune response, wound healing, neuronal signalling, cardiovascular regulation, and anti-bacterial and parasitism applications. | |
Hence, NO can inspire great hope for solving complicated medical problems with one simple strike. Lots of therapeutic approaches and medical materials have been developed by employing NO as the main active agent.13 Although NO may prove to be a promising all-rounder, its bright prospect is darkened, interestingly, by the very quality that gives rise to its glory. Its high reactivity allows NO to be easily scavenged by various chemicals via oxidation–reduction reactions and coordination interactions.14a Therefore, many chemicals, including reactive oxygen species (ROS), could influence the threshold of NO levels and modulate the signaling processes of NO.14b,c The clearance processes result in transitory half-lives and quite short diffusion radii of NO molecules.12b,15 As a consequence, the biological effects of NO are usually confined to the immediate area of the production source, seriously restricting its clinical application.
In order to break free from such an Ouroboros-like paradox, various compounds with an NO releasing function have been isolated and evaluated. They constitute the 16 different main categories of both natural and artificial NO donors16 and the list is being added to, becoming longer and longer even as we sit and discuss. Early practical NO carriers proved to be extremely sensitive to exogenous stimuli, such as light irradiation, protonic environments and heat.17 Hence, most of the designs focused on improving the overall stability, via chemical modification and physical encapsulation.21b,c,34,35 These upgraded NO delivery systems demonstrated great promise in medical applications, including for relieving acute angina,18 preventing coagulation on cardiovascular devices,19 reducing ischemia reperfusion injury20 and inhibiting bacteria and parasite proliferation.21 It seemed that the golden age of NO was just around the corner.
However, a large portion of NO action sites are inside cells.22 In the case of modulating smooth muscle cells (SMCs), NO generated from nearby endothelial cells (ECs) could easily diffuse through the cell membrane, binding to the reduced haem (Fe2+) of soluble guanylyl cyclase (sGC) inside the underlying SMCs.26c The closeness of the ECs and SMCs in space guarantees that NO is locally released and mainly distributed inside the targeted cells. On the contrary, most NO applicable donors are administrated orally, or by inhalation or blood injection. These unspecific delivery methods will undoubtedly cause an indiscriminate distribution of NO, which will further bring about mispairings between the NO release and action sites. Considering the transient life of NO, these mismatches may remarkably reduce its bio-utilization efficiency and dampen the overall outcome.23 As a consequence, extra NO is usually required to maintain a sufficient critical concentration and compensate for the weakened effects. This is usually achieved by increasing the number of times of administration or elevating the payload ratio, which may prove burdensome in both practice and economy. Also, an excessively high NO concentration may be accompanied by undesirable side effects. In the case of nitroglycerin, tolerance may develop after long-term usage.24 In addition, high levels of oxynitride may also increase the chance of generating carcinogenic nitrosamine, bringing its general safety into question.25 Such demerits may lead to even more challenges for NO applications.
After tens of thousands of years of evolution, the human body has built a genius system to overcome these problems. NO is almost exclusively generated by NO synthase enzymes (e.g. iNOS, eNOS and nNOS) in vivo, via the oxidation of L-arginine to L-citrulline.26 eNOS and nNOS are continuously expressed in endothelial cells and neuron cells, respectively. Their sustained presence is important in maintaining the crucial functions of the host cells and their effect on the immediate surroundings.27 As a complement to eNOS and nNOS, iNOS is usually present at locations where NO is required, far away from endothelial and neuron cells. Its occasional expression is mostly induced by certain internal triggers, as indicated by its name.28 Besides, many amino acids and proteins in the blood can act as media and long range NO carriers.29 Some can be recognized and captured by ligands on the cellular membrane, and sequentially internalized by the cells.30 Others may react with chemicals exposed at cell surfaces, converting the nitric species they carried to allow them to be further brought into the cells.31 Together, the two stage system guarantees that NO generation only occurs at target sites in a tunable manner and most NO is intracellularly delivered, thus confining its effects largely to areas of interest and minimizing the influence on irrelevant cells to the maximum degree.
So, the lessons we can learn from mother nature are that the local generation and intracellular release of NO is no less vital than improving the stability of the carriers. One potential option is to specifically induce NO generation in targeted cells. This may include methods such as the delivery of natural NOS and their artificial mimics into cells, or transfecting cells with genetic materials encoded with an NOS sequence.32 On the other hand, novel NO carriers with features such as fast cellular internalization and endogenous stimuli release also hold great promise. Enzymes or glutathione may act as excellent intracellular stimuli to initiate NO offloading. An NO delivery system that can utilize these typical cellular triggers is highly favored.33 Macromolecules with a glycosidase triggered NO releasing ability have been synthesized by attaching galactose conjugated diazeniumdiolates to biocompatible chitosan. NO was readily released in an enzymatic responsive manner and the materials could be easily processed into different forms, depending on the application scenario (Fig. 2).33c Besides, saccharides, peptides and other cell targeting ligands can also be attached to NO donors to enhance their endocytosis.34 It is even better if the conjugated species can stabilize the active NO derivatives (Fig. 3).35 These efforts and proposals may represent a new direction for future NO exploration. Meanwhile, smart vehicles with stimuli-responsiveness and intracellular delivery capacity have long been the focus in fields such as cancer therapy and the fighting of microbes.36 The mature concepts and approaches derived from these studies may also prove helpful to inspire new ideas and to overcome the aforementioned difficulties in the research of NO.
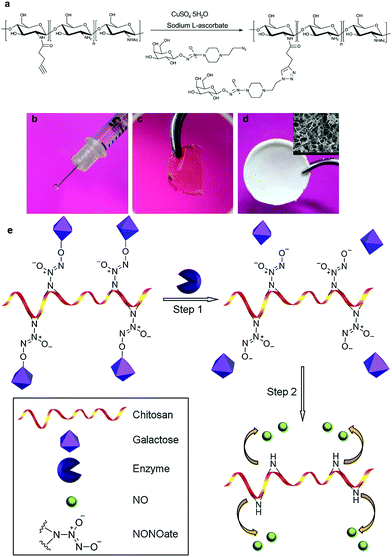 |
| Fig. 2 (a) Glycosidase responsive NO prodrugs were conjugated to chitosan. The material could be processed into different forms, including (b) an injectable gel, (c) a transparent membrane and (d) a porous scaffold. (e) NO could be freed via glycosidase activated cleavage of galactose residues. (modified from ref. 33c by permission of Elsevier). | |
 |
| Fig. 3 Diazeniumdiolates could be stabilized by (a) saccharides and (b) peptides, which might also enhance the cellular internalization of these NO prodrugs. NO could be released via enzymatic cleavage of the protecting groups (modified from ref. 35 by permission of Elsevier). | |
Although there may be many obstacles ahead, we remain optimistic and hold a strong belief in the application of NO in medical practice. Like a naughty youngster, NO has proven to be tough to discipline. But it is from these trials and errors that our creativity is motivated, skills tested and knowledge expanded. The endeavours of researchers all over the world will at last make the NO boy grow into a magnificent giant shouldering the health and well-being of man-kind.
Conflicts of interest
There are no conflicts to declare.
Acknowledgements
Financial support from the National Natural Science Foundation of China (51333005) and the National Key Research and Development Program of China (2017YFGX090043) is gratefully acknowledged.
Notes and references
-
N. Ringertz, Alfred Nobel's health and his interest in medicine, The Nobel Foundation, 1998 Search PubMed.
-
(a)
P. M. Vanhoutte and J. T. Shepherd, Vasodilatation: Vascular Smooth Muscle, Peptide, Autonomic Nerves and Endothelium, Raven Press, New York, 1988, p. 401 Search PubMed;
(b)
P. M. Vanhoutte and J. T. Shepherd, Vasodilatation: Vascular Smooth Muscle, Peptide, Autonomic Nerves and Endothelium, Raven Press, New York, 1988, p. 427 Search PubMed;
(c) R. F. Furchgott and J. V. Zawadzki, Nature, 1980, 288, 373 CrossRef CAS PubMed;
(d) A. R. Butler and C. Glidewell, Chem. Soc. Rev., 1987, 16, 361 RSC;
(e) R. J. P. Williams, Chem. Soc. Rev., 1996, 25, 77 RSC.
- J. B. Hibbs Jr., Res. Immunol., 1991, 142, 565 CrossRef CAS.
- J. Garthwaite, Trends Neurosci., 1991, 14, 60 CrossRef CAS PubMed.
-
(a) L. J. Ignarro, Semin. Hematol., 1989, 26, 63 CAS;
(b) L. J. Ignarro, J. Pharmacol. Res., 1989, 6, 651 CrossRef CAS;
(c) P. Ganz, P. F. Davies, J. A. Leopold, M. A. Gimbrone Jr. and R. W. Alexander, Proc. Natl. Acad. Sci. U. S. A., 1986, 83, 3552 CrossRef CAS PubMed.
- D. E. Koshland Jr., Science, 1992, 258, 1861 CAS.
-
(a) F. Murad, Angew. Chem., Int. Ed., 1999, 38, 1856 CrossRef CAS;
(b) R. F. Furchgott, Angew. Chem., Int. Ed. Engl., 1999, 38, 1870 CrossRef CAS;
(c) L. J. Ignarro, Angew. Chem., Int. Ed., 1999, 38, 1882 CrossRef CAS.
-
(a) G. M. Rosen, P. Tsai and S. Pou, Chem. Rev., 2002, 102, 1191 CrossRef CAS PubMed;
(b) P. C. Ford and I. M. Lorkovic, Chem. Rev., 2002, 102, 993 CrossRef CAS PubMed;
(c) J. Lee, L. Chen, A. H. West and G. B. Richter-Addo, Chem. Rev., 2002, 102, 1019 CrossRef CAS PubMed;
(d) I. M. Wasser, V. S. De, P. Moënne-Loccoz, I. Schröder and K. D. Karlin, Chem. Rev., 2002, 102, 1201 CrossRef CAS PubMed.
-
(a) T. M. Griffith, D. H. Edwards, R. L. Davies, T. J. Harrison and K. T. Evans, Nature, 1987, 329, 442 CrossRef CAS PubMed;
(b) T. M. Griffith, D. H. Edwards, M. J. Lewis, A. C. Newby and A. H. Henderson, Nature, 1984, 308, 645 CrossRef CAS PubMed;
(c) R. F. Furchgott, Annu. Rev. Pharmacol. Toxicol., 1984, 24, 175 CrossRef CAS PubMed;
(d) R. Busse, G. Trogisch and E. Bassenge, Basic Res. Cardiol., 1985, 80, 475 CrossRef CAS PubMed.
-
(a) N. S. Kwon, C. F. Nathan and D. J. Stuehr, J. Biol. Chem., 1989, 264, 20496 CAS;
(b) N. S. Kwon, C. F. Nathan, C. Gilker, O. W. Griffith, D. E. Matthews and D. J. Stuehr, J. Biol. Chem., 1990, 265, 13442 CAS;
(c) A. M. Leone, R. M. Palmer, R. G. Knowles, P. L. Francis, D. S. Ashton and S. Moncada, J. Biol. Chem., 1991, 266, 23790 CAS.
-
(a) D. E. Heck, D. L. Laskin, C. R. Gardner and J. D. Laskin, J. Biol. Chem., 1992, 267, 21277 CAS;
(b) L. Xiao, D. R. Pimentel, J. Wang, K. Singh, W. S. Colucci and D. B. Sawyer, Am. J. Physiol.: Cell Physiol., 2001, 281, 1971 CrossRef PubMed;
(c) L. L. Shears, M. R. Kibbe, A. D. Murdock, T. R. Billiar, A. Lizonova, I. Kovesdi, S. C. Watkins and E. Tzeng, J. Am. Coll. Surg., 1998, 187, 295 CrossRef PubMed;
(d) J. Luo and A. F. Chen, Acta Pharmacol. Sin., 2005, 26, 259 CAS.
-
(a) X. Zhang, G. Tian, W. Yin, L. Wang, X. Zheng, L. Yan, J. Li, H. Su, C. Chen, Z. Gu and Y. Zhao, Adv. Funct. Mater., 2015, 25, 3049 CrossRef CAS;
(b) J. Xu, F. Zeng, H. Wu, C. Hu, C. Yu and S. Wu, Small, 2014, 10, 3750 CrossRef CAS PubMed;
(c) J. Fan, N. He, Q. He, Y. Liu, Y. Ma, X. Fu, Y. Liu, P. Huang and X. Chen, Nanoscale, 2015, 7, 20055 RSC.
-
(a) A. B. Seabra and N. Durán, J. Mater. Chem., 2010, 20, 1624 RSC;
(b) A. W. Carpenter and M. H. Schoenfisch, Chem. Soc. Rev., 2012, 41, 3742 RSC.
-
(a) D. A. Riccio and M. H. Schoenfisch, Chem. Soc. Rev., 2012, 41, 3731 RSC;
(b) R. A. Khalil and J. P. Granger, Am. J. Physiol.: Regul., Integr. Comp. Physiol., 2002, 283, 29 CrossRef PubMed;
(c) L. A. Ridnour, D. D. Thomas, C. Switzer, W. Flores-Santana, J. S. Isenberg, S. Ambs, D. D. Roberts and D. A. Wink, Nitric Oxide, 2008, 19, 73 CrossRef CAS PubMed.
-
(a)
L. J. Ignarro, Nitric Oxide: Biology and Pathobiology, Academic Press, San Diego, CA, 2000 Search PubMed;
(b) S. Sortino, J. Mater. Chem., 2011, 22, 301 RSC.
- P. G. Wang, M. Xian, X. Tang, X. Wu, Z. Wen, T. Cai and A. J. Janczuk, Chem. Rev., 2002, 102, 1091 CrossRef CAS PubMed.
-
(a) D. J. Smith, D. Chakravarthy, S. Pulfer, M. L. Simmons, J. A. Hrabie, M. L. Citro, J. E. Saavedra, K. M. Davies, T. C. Hutsell, D. L. Mooradian, S. R. Hanson and L. K. Keefer, J. Am. Chem. Soc., 1960, 82, 96 CrossRef;
(b) V. R. Zhelyaskov and D. W. Godwin, Nitric Oxide, 1999, 3, 419 CrossRef CAS PubMed;
(c) J. Hwu, C. Yau, S. Tsay and T. Ho, Tetrahedron Lett., 1997, 38, 9001 CrossRef CAS.
- M. J. Peach, H. A. Singer, N. J. Izzo and A. L. Lobe, Am. J. Cardiol., 1987, 60, 1 CrossRef.
-
(a) J. Loscalzo, Circ. Res., 2001, 88, 756 CrossRef CAS PubMed;
(b) M. Mehta, R. C. Darling, S. P. Roddy, S. Fecteau, K. J. Ozsvath, P. B. Kreienberg, P. S. K. Paty, B. Chang and D. M. Shah, J. Vascular Surgery, 2005, 42, 1047 CrossRef PubMed.
-
(a) T. A. Johnson, N. A. Stasko, J. L. Matthews, W. E. Cascio, E. L. Holmuhamedov, C. B. Johnson and M. H. Schoenfisch, Nitric oxide, 2010, 22, 30 CrossRef CAS PubMed;
(b) R. Schulz, M. Kelm and G. Heusch, Cardiovasc. Res., 2004, 61, 402 CrossRef CAS PubMed;
(c) X. Liu, Y. Huang, P. Pokreisz, P. Vermeersch, G. Marsboom, M. Sweinnen, E. Verbeken, J. Santos, M. Pellens, H. Gillijns, F. Van de Werf, K. D. Bloch and S. Janssens, J. Am. Coll. Cardiol., 2007, 50, 808 CrossRef CAS PubMed;
(d) R. M. Bell, H. L. Maddock and D. M. Yellon, Cardiovasc. Res., 2003, 57, 405 CrossRef CAS PubMed;
(e) E. A. Konorev, M. M. Tarpey, J. Joseph, J. E. Baker and B. Kalyanaraman, J. Pharmacol. Exp. Ther., 1995, 274, 200 CAS.
-
(a) S. M. Marxer, A. R. Rothrock, B. J. Nablo, M. E. Robbins and M. H. Schoenfisch, Chem. Mater., 2003, 15, 4193 CrossRef CAS;
(b) P. N. Coneski, J. A. Nash and M. H. Schoenfisch, ACS Appl. Mater. Interfaces, 2011, 3, 426 CrossRef CAS PubMed;
(c) J. H. Shin, S. K. Metzger and M. H. Schoenfisch, J. Am. Chem. Soc., 2007, 129, 4612 CrossRef CAS PubMed;
(d) A. W. Carpenter, D. L. Slomberg, K. Rao and M. H. Schoenfisch, ACS Nano, 2011, 5, 7235 CrossRef CAS PubMed;
(e) A. R. Ketchum, M. P. Kappler, J. Wu, C. Xi and M. E. Meyerhoff, J. Mater. Chem. B, 2016, 4, 422 RSC;
(f) A. Rivero, Trends Parasitol., 2006, 5, 219 CrossRef PubMed;
(g) L. R. Brunet, Int. Immunopharmacol., 2001, 8, 1457 CrossRef;
(h) J. L. Stafford, N. F. Neumann and M. Belosevic, Crit. Rev. Microbiol., 2002, 3, 187 CrossRef PubMed;
(i) D. O. Schairer, J. S. Chouake, J. D. Nosanchuk and A. J. Friedman, Virulence, 2012, 3, 271 CrossRef PubMed.
-
(a) S. Moncada and E. A. Higgs, Eur. J. Clin. Invest., 1991, 21, 361 CrossRef CAS PubMed;
(b) S. H. Snyder, Science, 1992, 257, 494 CAS;
(c) U. Forstermann, E. I. Closs, J. S. Pollock, M. Nakane, P. Schwarz, I. Gath and H. Kleinert, Hypertension, 1994, 23, 1121 CrossRef CAS PubMed;
(d) P. Huang, T. M. Dawson, D. S. Bredt, S. H. Snyder and M. C. Fishman, Cell, 1993, 75, 1273 CrossRef CAS PubMed;
(e) P. Huang, Z. Huang, H. Mashimo, K. D. Moskowitz, J. A. Bevan and M. C. Fishman, Nature, 1995, 377, 239 CrossRef CAS PubMed;
(f) H. M. Lander, FASEB J., 1997, 11, 118 CrossRef CAS PubMed.
-
(a) L. K. Keefer, Curr. Top. Med. Chem., 2005, 5, 625 CrossRef CAS PubMed;
(b) A. A. Eroyreveles and P. K. Mascharak, Future Med. Chem., 2009, 1, 1497 CrossRef CAS.
- A. Chung, H. Yang and C. van Breemen, Br. J. Pharmacol., 2007, 151, 305 Search PubMed.
-
(a) S. Mocellin and V. D. Bronte, Med. Res. Rev., 2007, 27, 317 CrossRef CAS PubMed;
(b) D. D. Thomas, L. A. Ridnour, J. S. Isenberg, W. Flores-Santana, C. H. Switzer, S. Donzelli, P. Hussain, C. Vecoli, N. Paolocci, S. Ambs, C. A. Colton, C. C. Harris, D. D. Roberts and D. A. Wink, Free Radical Biol. Med., 2008, 45, 18 CrossRef CAS PubMed.
-
(a) D. J. Stuehr, N. S. Kwon, C. F. Nathan, O. W. Griffith, P. L. Feldman and J. Wiseman, J. Biol. Chem., 1991, 266, 6259 CAS;
(b) M. A. Marletta, A. R. Hurshman and K. M. Rusche, Curr. Opin. Chem. Biol., 1998, 2, 656 CrossRef CAS PubMed;
(c) J. O. Lundberg, M. T. Gladwin and E. Weitzberg, Nat. Rev. Drug Discovery, 2014, 623–641 Search PubMed.
-
(a) J. S. Pollock, U. Förstermann, J. A. Mitchell, T. D. Warner, H. H. Schmidt, M. Nakane and F. Murad, Proc. Natl. Acad. Sci. U. S. A., 1991, 88, 10480 CrossRef CAS PubMed;
(b) S. Lamas, P. A. Marsden, G. Li, P. Tempst and T. Michel, Proc. Natl. Acad. Sci. U. S. A., 1992, 89, 6348 CrossRef CAS PubMed;
(c) D. S. Bredt, P. M. Hwang, C. E. Glatt, C. Lowenstein, R. R. Reed and S. H. Snyder, Nature, 1991, 351, 714 CrossRef CAS PubMed;
(d) J. E. Brenman, D. S. Chao, S. H. Gee, A. W. McGee, S. E. Craven, D. R. Santillano, Z. Wu, F. Huang, H. Xia, M. F. Peters, S. C. Froehner and D. S. Bredt, Cell, 1996, 84, 757 CrossRef CAS PubMed.
-
(a)
S. Moncada, M. A. Marletta, J. B. Hibbs Jr. and E. A. Higgs, The Biology of Nitric Oxide: 2. Enzymology, Biochemistry and Immunology, Portland Press, London, 1992, p. 19 Search PubMed;
(b) D. J. Stuehr, H. J. Cho, N. S. Kwon, M. F. Weise and C. F. Nathan, Proc. Natl. Acad. Sci. U. S. A., 1991, 88, 7773 CrossRef CAS.
-
(a) J. S. Stamler, O. Jaraki, J. Osborne, D. I. Simon, J. Keaney, J. Vita, D. Singel, C. R. Valeri and J. Loscalzo, Proc. Natl. Acad. Sci. U. S. A., 1992, 89, 7674 CrossRef CAS PubMed;
(b) E. Nagababu and J. M. Rifkind, Methods Mol. Biol., 2011, 704, 27 CrossRef CAS PubMed;
(c) J. T. Mattila and A. C. Thomas, Front. Immunol., 2014, 5, 478 CrossRef PubMed.
-
(a) R. L. Davisson, M. D. Travis, J. N. Bates and S. J. Lewis, Circ. Res., 1996, 79, 256 CrossRef CAS PubMed;
(b) M. D. Travis, R. L. Davisson, J. N. Bates and S. J. Lewis, J. Am. Physiol., 1997, 273, 1493 Search PubMed;
(c) S. Satoh, T. Kimura, M. Toda, M. Maekawa, S. Ono, H. Narita, H. Miyazaki, T. Murayama and Y. Nomura, J. Neurochem., 1997, 69, 2197 CrossRef CAS PubMed.
-
(a) Y. Zhang and N. Hogg, Free Radical Biol. Med., 2005, 38, 831 CrossRef CAS PubMed;
(b) R. J. Mallis and J. A. Thomas, Arch. Biochem. Biophys., 2000, 383, 60 CrossRef CAS PubMed;
(c) Y. Zhang and N. Hogg, Proc. Natl. Acad. Sci. U. S. A., 2004, 101, 7891 CrossRef CAS PubMed.
- W. Fan, N. Lu, P. Huang, Y. Liu, Z. Yang, S. Wang, G. Yu, Y. Liu, J. Hu, Q. He, J. Qu, T. Wang and X. Chen, Angew. Chem., Int. Ed., 2017, 56, 1229 CrossRef CAS PubMed.
-
(a) W. Cai, J. Wu, C. Xi and M. E. Meyerhoff, Biomaterials, 2012, 33, 7933 CrossRef CAS PubMed;
(b) P. J. Shami, J. E. Saavedra, Y. Lai, C. L. Bonifant, B. A. Diwan, S. V. Singh, Y. Gu, S. D. Fox, G. S. Buzard, M. L. Citro, D. J. Waterhouse, K. M. Davies, X. Ji and L. K. Keefer, Mol. Cancer Ther., 2003, 2, 409 CAS;
(c) Q. Zhao, J. Zhang, L. Song, Q. Ji, Y. Yao, Y. Cui, J. Shen, P. G. Wang and D. Kong, Biomaterials, 2013, 34, 8450 CrossRef CAS PubMed.
-
(a) Y. Hou, J. Wang, P. R. Andreana, G. Cantauria, S. Tarasia, L. Sharp, P. G. Braunschweiger and P. G. Wang, Bioorg. Med. Chem. Lett., 1999, 9, 2255 CrossRef CAS PubMed;
(b) J. Ramirez, L. Yu, P. G. Braunschweiger and P. G. Wang, Bioorg. Med. Chem. Lett., 1996, 6, 2575 CrossRef CAS;
(c) N. Barnabé, R. A. Marusak and B. B. Hasinoff, Nitric oxide, 1997, 1, 211 CrossRef PubMed.
-
(a) X. Tang, M. Xian, M. Trikha, K. V. Honn and P. G. Wang, Tetrahedron Lett., 2001, 42, 2625 CrossRef CAS;
(b) X. Wu, X. Tang, M. Xian and P. G. Wang, Tetrahedron Lett., 2001, 42, 3779 CrossRef CAS.
-
(a) M. J. Webber and R. S. Langer, Chem. Soc. Rev., 2017, 46, 6600 RSC;
(b) M. Karimi, A. Ghasemi, P. S. Zangabad, R. Rahighi, S. M. Moosavi Basri, H. Mirshekari, M. Amiri, Z. S. Pishabad, A. Aslani, M. Bozorgomid, D. Ghosh, A. Beyzavi, A. Vaseghi, A. R. Aref, L. Haghani, S. Bahrami and M. R. Hamblin, Chem. Soc. Rev., 2016, 45, 1457 RSC;
(c) R. Tong, L. Tang, L. Ma, C. Tu, R. Baumgartner and J. Cheng, Chem. Soc. Rev., 2014, 43, 6982 RSC;
(d) Z. Ge and S. Liu, Chem. Soc. Rev., 2013, 42, 7289 RSC;
(e) Y. Zhang, J. Yu, N. H. Bomba, Y. Zhu and Z. Gu, Chem. Rev., 2016, 116, 12536 CrossRef CAS PubMed;
(f) P. T. Wong and S. K. Choi, Chem. Rev., 2015, 115, 3388 CrossRef CAS PubMed;
(g) K. E. Uhrich, S. M. Cannizzaro, R. S. Langer and K. M. Shakesheff, Chem. Rev., 1999, 99, 3181 CrossRef CAS PubMed;
(h) Y. Wang, V. Bansal, A. N. Zelikin and F. Caruso, Chem. Rev., 2016, 116, 3888 Search PubMed;
(i) X. Zhang, L. Han, M. Liu, K. Wang, L. Tao, Q. Wan and Y. Wei, Mater. Chem. Front., 2017, 807 RSC;
(j) A. Varela-Moreira, Y. Shi, M. H. A. M. Fens, T. Lammers, W. E. Hennink and R. M. Schiffelers, Mater. Chem. Front., 2017, 1, 1485 RSC.
|
This journal is © the Partner Organisations 2018 |