Impact of infrared radiation on UVB-induced skin tumourigenesis in wild type C57BL/6 mice†
Received
20th March 2018
, Accepted 6th October 2018
First published on 8th October 2018
Abstract
Although infrared radiation (IR) represents more than 50% of the solar radiation reaching the Earth's surface, this waveband has been hardly investigated in terms of tumourigenesis. The objective of the present study was to investigate the influence of IR on ultraviolet B (UVB)-induced carcinogenesis in male and female wild type mice. For this purpose, male and female C57BL/6N mice were subjected to a long-term irradiation protocol. Mice were irradiated once neonatally and from the age of eight weeks for 36 weeks with a cumulative dose of 576 kJ m−2 UVB and/or 78
895 kJ m−2 IR. In order to resemble natural sun irradiation, exposure to physiological doses of UVB and IR was performed simultaneously. Mice were screened for arising lesions twice a week. Lesions were excised and histologically diagnosed. Kaplan–Meier analyses were carried out and lesion counts and cumulated hazard rates for the development of lesions in the UVB and IR + UVB-exposed groups in male and female mice were compared. We found that IR-exposure did not change the number of epithelial malignant tumours in UVB-exposed wild type mice. In combination with IR there was a tendency of more tumours with increased malignancy: 23 vs. seven spindle cell shaped sarcomas and seven vs. two MelanA+/S100+ tumours in groups of 35 C57BL/6 mice. IR did not influence UVB-induced carcinogenesis differently in male and female mice. However, comparing UVB and sham irradiated animals irrespective of IR exposure, UVB-induced non-epithelial tumours arose significantly earlier in male mice than in female mice.
Introduction
Besides visible light and ultraviolet radiation (UVR), terrestrial optical solar radiation comprises in large part (∼50%) infrared radiation (IR, 760 nm–1
000
000 nm).1 Infrared A radiation (IRA, 760 nm–1400 nm) is the most abundant part within the IR waveband.2 In contrast to IRB (1400 nm–3000 nm) and IRC (3000 nm–1
000
000 nm), which are absorbed within the uppermost layers of the skin, IRA can penetrate up to several centimetres into tissues.3 There is increasing evidence that IR exerts waveband specific effects in tissues and cells which are independent of heat. It was reported that IR impacts several cellular mechanisms, ranging from apoptosis and calcium signalling, over skin aging to proposed contributions to skin carcinogenesis.4–8 The widespread use of sunscreens protecting from UVR enables people to stay in the sun for a longer time which results in a higher cumulative IR dose.1
Since IR is abundantly present in natural sunlight, epidemiologic studies investigating the impact of UVR on skin carcinogenesis always imply also a contribution of IR. Nevertheless, IR has been hardly considered to play a role in photocarcinogenesis. Moreover, little is known about the interactions of IR with other solar wavebands for example UVR. However, there are studies indicating that IR modifies the impact of UVR on tissues and cells. It has been shown that the combination of UVR and IR leads to enhanced elastic fibre deposition in the dermis.9 On the other hand IR protects fibroblasts from UVR-induced toxicity.10 Moreover, IRA decreases UVB-induced apoptosis while increasing the repair of UVB-induced DNA damage in primary murine keratinocytes.11 In contrast, it was recently demonstrated that IRA does not affect the repair of UVB-induced DNA damage while it decreases UVB-induced apoptosis in primary human melanocytes. Hence it was speculated that IRA might contribute to melanomagenesis as it promotes survival of UVB-damaged melanocytes.8,12 A murine in vivo study revealed that IRA influences the growth behaviour of UVB-induced skin tumours in terms of enhancing their proliferative capacity, particularly of non-epithelial tumours. Noteworthily, in this study two melanomas were diagnosed in mice exposed to both IRA and UVB, while none was observed in only UVB irradiated animals.8,13
In the present study gender specific aspects were also investigated. In humans, there are differences between men and women in melanoma and non-melanoma skin cancer (NMSC) incidence as they seem to be more prevalent in men than in women. Moreover, melanomas in men have a higher mutational burden and male patients have worse prognoses. In contrast, trends of a higher incidence of melanomas in women under 50 were recently observed.14–16 The higher skin tumour burden of men might be partly related to a less careful behaviour of males concerning sun protection and dermatological examination whereas the elevated risk for young women for melanomas might be attributed to indoor tanning demographics.14,17
However, also in hairless albino Skh-1 mice it was shown that male animals develop UVB-induced epithelial tumours earlier than their female counterparts and the tumours are more aggressive. It is believed that the more aggressive behaviour is attributed to a lower anti-oxidative capacity of the male skin.18 In a haired albino mouse model it was shown that oestrogen has a protective effect against the development of NMSC.19 In addition, it has been demonstrated that androgens partially inhibit UVB-induced vitamin D synthesis in the skin of male C57BL/6 mice.20 To our knowledge, the influence of gender on non-epithelial solar radiation-induced skin cancers in pigmented wild type mice has not yet been investigated.
The aim of the present study was to investigate the effect of IRA on skin photocarcinogenesis in a wild type mouse strain. As compared to previous studies investigating similar problems,13,21 (1) we employed a wild type mouse model, (2) we used a higher number of mice per group, (3) in order to study the influence of gender on photocarcinogenesis mice of both sexes were exposed, (4) simultaneous exposure to IRA and UVB mimicked natural sun exposure, and (5) an additional neonatal exposure of the pups to UVB and/or IRA was introduced. The neonatal exposure on postnatal day three was performed as at this stage of development a considerable number of melanocytes are located in the epidermis of the pups.22,23 To our knowledge, neonatal exposure has never been included in this kind of study. The proposed irradiation protocol unifies two epidemiologically supported hypotheses that either high dose early childhood exposure to solar radiation and/or intermittent cumulative lifetime exposure might promote different types of skin cancers.24–26
Materials and methods
Mice
Wild type C57BL/6N mice for breeding were purchased from Charles River. Mice for the experiment were bred in-house. Animal care was provided by professional animal caretakers and supervised by veterinarians of the Centre for Biomedical Research. Animals were housed under local standards with a day/night cycle of twelve hours and received a mouse diet (ssniff Spezialdiäten GmbH, Soest, Germany) and drinking water ad libitum. All mouse experiments were approved by the Austrian Federal Ministry of Science, Research and Economy (BMWFW-66.009/0341-II/3b/2013).
Lamps and dosimetry
IRA was delivered using a Hydrosun®750 water-filtered IRA lamp (Hydrosun, Mühlheim, Germany) equipped with a 760 nm cut-off filter, emitting between 760 and 1440 nm. IRA dosimetry was performed using an HBM1 infrared exposure measurement device (Hydrosun). The IRA flux ranged from 0.50–0.60 kW m−2, and mice were exposed for 30 minutes per session. In the present mouse study the IRA flux was reduced from 1350 kJ m−2 per session in previous studies to 1000 kJ m−2 per session to reduce thermal effects (especially in combination with UVB) and to adjust the flux to a more natural value.13
UVB exposure was carried out using a custom-made Waldmann TP-4 lamp (Herbert Waldmann GmbH & Co. KG, Villingen-Schwenningen, Germany) equipped with two Philips TL/12 broadband UVB tubes, emitting light almost exclusively in the UVB region, peaking at ∼313 nm. UVB dosimetry was carried out using an IL1700 research radiometer (International light, Newburyport, MA) with an SED240#3143 detector/NS313#12178 filter measuring the flux at 313 nm. In order to keep the exposure times as constant as possible, the distance between the lamp and mice was adjusted. This procedure resulted in a UVB flux ranging from 2.3 to 5.6 W m−2 and exposure times of 18–30 minutes.
Irradiation protocol
Mice were subjected to an irradiation regimen consisting of a combination of two established photocarcinogenesis protocols.13,27 To ensure equally distributed irradiation intensity, during irradiation mice of one group were put into Type II cages (floor area: 375 cm2), capped with a transparent net (included in irradiation intensity calculations). During irradiation cages were cooled using a transportable air conditioner and a cooling plate. The experimental setup was unchanged throughout the duration of the whole irradiation period. Immediately after birth mice were randomly allocated to four groups: (1) IRA + UVB, (2) UVB, (3) IRA, and (4) control group (sham irradiated). For practical reasons littermates were assigned to the same group. Litter of one mouse was assigned randomly to one of the four groups, until the number of 35 mice per group was reached: 20 females and 15 males. Mice were kept in groups of ten (females), seven and eight (males) in Makrolon Type IV cages in order to reduce the agonistic behaviour of the mice towards each other. In accordance with the irradiation protocol by Noonan et al., neonatal mice were exposed to single irradiation at day three with 1000 kJ m−2 IRA and/or 6.0 kJ m−2 UVB.27 At this age C57BL/6N mice were not yet pigmented. Between the age of eight and 45 weeks mice were exposed one to three times per week to 1000 kJ m−2 of IRA and/or increasing doses of UVB (2.5 kJ m−2 to 10 kJ m−2), as described in ref. 13. Mice were exposed three times per week for 24 weeks according to an established protocol.13 If one of the mice suffered from intermediate stress (rating 1 according to ESI, Table 1†) single exposures were postponed to the end of the exposure period for all mice. Prior to each exposure backs of the mice (and backs of the control mice) were shaved. Through the irradiation protocol mice were exposed to a cumulative dose of 78
895 kJ m−2 IRA and/or 576 kJ m−2 UVB.
Development of tumours
Mice were monitored at least twice per week and their physical and mental health was graded according to a criteria catalogue given in ESI, Table 1.† All lesions were documented. Tumour volumes were calculated according to the US National Cancer Institute formula:
, where V is the volume of the tumour, L is the largest diameter of the tumour and l is the smallest diameter of the tumour. If lesions were observed but vanished at a later time point (and never reached the size of V = 200 mm3), lesions’ photographic records were kept, but no histological examination of the respective lesions was possible and therefore they were excluded from the evaluation. We compared the lesion-free survival curves of mice including the non-histologically confirmed lesions and were not able to see significant differences in the curves without these lesions. The size of every lesion was measured in three dimensions using a caliper, twice a week. A lesion was excised at a volume V of ≥200 mm3 or if it was ulcerating. If a mouse had more than one lesion at the time of surgery, all existing lesions of the respective mouse were excised in order to avoid more surgery than necessary. Mice were anaesthetized using 0.30 mg per kg body weight medetomidine, 1.00 mg per kg body weight midazolam, 0.03 mg per kg body weight fentanyl and 10.00 mg per kg body weight ketamine, injected intraperitoneally. After surgery medetomidine and midazolam were antagonized using 0.5 mg per kg body weight atipamezole and 0.1 mg per kg body weight flumazenil, respectively. Mice were kept extra in Type II cages, which were located in the Type IV cage of their cage mates in order to keep contact with their fellows and at the same time guaranteeing an uncomplicated wound closure. After wound closure mice were put back with their cage mates. Overall, a single mouse underwent zero to five surgical interventions.
Processing of lesions and tissues
After surgery or sacrifice of mice the bulk of the lesions were formalin fixed and paraffin embedded, whereas a part of each lesion was cryo-preserved for further usage, both using standard histology protocols. At sacrifice, mice were killed by cervical dislocation.
Evaluation of lesions
Tumours were diagnosed and graded independently by two dermato-histopathologists according to criteria stated below.
Macroscopically: endophytic or exophytic growth, erosion, colour (red, skin-coloured, dark), hair growth on tumours, consistency hard or soft.
Microscopically: evaluation of haematoxylin/eosin (H&E) stained paraffin sections according to standard histopathological parameters. Lesions were diagnosed as non-neoplastic, benign and malignant. Immunohistochemistry was performed using antibodies against S100, MelanA, pan-cytokeratin, vimentin and SMA.
All histologically diagnosed lesions were counted and used for analysis. We analysed survival curves including non-histologically confirmed lesions (counted as proliferative epithelial lesions) and did not observe significant differences in the curves without these lesions.
Immunohistochemistry protocol
Immunohistochemistry (IHC) was performed using a Polink-2 HRP Plus Rabbit AEC Detection System for immunohistochemistry supersensitive to AEC Chromogen (GBI Labs, Bothell, WA) according to the manufacturer's instruction. In short, paraffin sections were fixed on coated slides. They were dewaxed using NeoClear® (Merck, Darmstadt, Germany) and decreasing ethanol dilutions. Endogenous peroxidase activity was blocked using 0.6% H2O2 in 80% methanol. Antigen retrieval was carried out by incubating cells in citrate buffer pH 6, at 80 °C for 20 minutes. Slides were stained with antibodies (Rabbit S100 (Dako Z0311), 1
:
3000; rabbit anti-wide spectrum cytokeratin antibody (Abcam ab9377), 1
:
150; rabbit MelanA/MART-1 antibody (Novus A19-P), 1
:
600; rabbit ACTA2/SMA antibody (Abcam ab5694), 1
:
200) diluted in 1% BSA/PBS for 120 minutes at room temperature. Slides were washed with PBS twice, and incubated with rbEnhancer for 10 minutes (3 parts enhancer + 1 part PBS) followed by two further washing steps with PBS. Slides were incubated with rbHRP for 10 minutes and washed twice with PBS. AEC staining was carried out using one drop of reagent A + one drop of reagent B + one drop of reagent C and one mL bi-distilled water. After incubation with AEC for ∼20 minutes slides were washed with tap water. Counterstaining was performed using Harris haematoxylin (2
:
3 in water) for 10–12 seconds. Slides were washed with warm tap water for ∼10 minutes and covered using Aquatex.
Data analysis and statistics
The major analysis methods (Kaplan–Meier curves and Nelson–Aalen estimator) account for censored events = deaths of mice. After classification of the lesions (all lesions = non-proliferative, benign and malignant lesions for which histological classification was available) of each mouse Kaplan Maier curves were used to calculate and present lesion-free survival of mice for different skin cancer entities. Differences between groups in terms of tumour free survival were calculated using the log-rank test. In the case of no significant difference in the lesion-free survival between the groups, UVB and IRA + UVB-exposed mice were pooled and evaluated with regard to gender specific differences. In order to describe the quantity of arisen lesions, average numbers of different lesions per mouse ± SD are given in addition to Kaplan–Maier-curves. Significance was calculated by applying two-sided, unpaired Student's t test (with the help of R version 3.4.2).28
The expected cumulative number of tumours (representative of the cumulative hazard rate) for each group was estimated using the Nelson–Aalen estimator. In the context of recurrent events the Nelson–Aalen estimator is a nonparametric estimator of the mean cumulative function of the counting process, which can be used for censored survival data.29 For group comparison a marginal means mode was calculated with treatment or gender as a fixed effect and taking into account the dependency of observations of the same mouse.30 The calculations were performed using R version 3.4.28 Models were fitted using the ‘coxph’ function with the option ‘cluster’ of the R-package ‘survival’.31
We corrected for multiple hypothesis testing, whereas hypotheses of exposure type and gender were considered as two independent tests. Among these two tests we corrected for multiple hypothesis testing using Bonferroni correction.
Mice also developed proliferative lesions after we excised the first tumours of the mice. Since the assignment of relapses turned out to be extremely challenging, all arising lesions were counted as individual tumours. To verify that relapses do not occur more frequently in one group than in another, we repeated all analyses using only tumours of mice occurring prior to the first surgery of the respective mouse. In doing so we obtained results resembling the calculations using all lesions. Therefore, in the whole manuscript we show data and figures that include all lesions of all mice, prior to and after the first surgery.
Results
UVB and IRA + UVB-induced skin lesions in C57BL6/N mice
Male and female C57BL/6N wild type mice were exposed to a long-term irradiation protocol as described in the Materials and methods section. Mice were monitored at least twice per week for the development of skin lesions. Almost all of the UVB and IRA + UVB-exposed mice developed proliferative skin lesions, 85% of them within 500 days from their birth (Fig. 1A). Among the mice not exposed to UVB, only a single IRA treated mouse developed a proliferative skin lesion (squamous cell carcinoma, SCC, well differentiated; Fig. 1A and B). Although we cannot rule out that IRA did contribute to the onset of this lesion, it appears very likely that it was a random event. The expected number of lesions as estimated by the Nelson–Aalen-estimator is not significantly different between UVB and IRA + UVB-exposed mice (ESI Fig. 1B;† every single lesion of each mouse is shown in ESI Fig. 2A and B†).
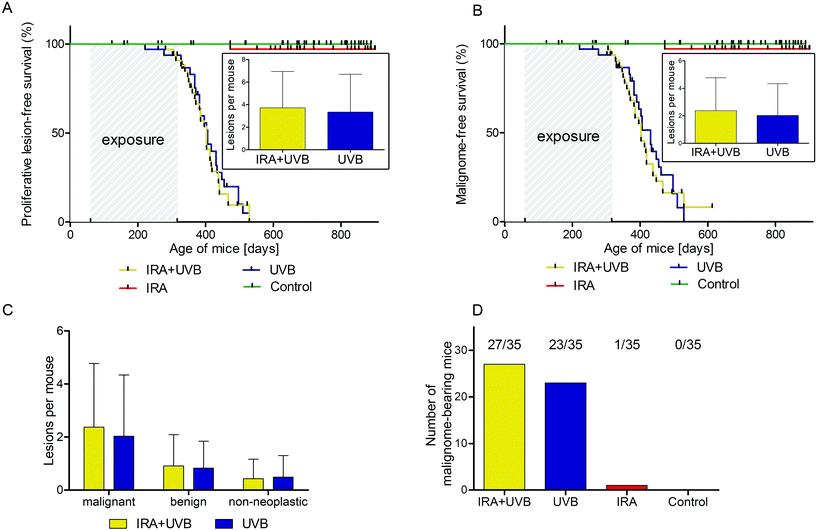 |
| Fig. 1 Development of lesions in mice exposed to UVB or IRA + UVB. (A) Kaplan–Meier-curve of proliferative lesion-free survival of mice exposed to UVB (blue), IRA + UVB (yellow) and IRA (red), and unexposed controls (green); UVB vs. IRA + UVB: p = 0.6667. Inset depicts the average number of proliferative lesions per mouse + SD developing in the two UVB-exposed groups; p = 0.5625. (B) Kaplan–Meier-curve of malignome-free survival of mice exposed to UVB (blue), IRA + UVB (yellow) and IRA (red), and unexposed controls (green); UVB vs. IRA + UVB: p = 0.6790. Inset depicts the average number of malignant lesions per mouse + SD developing in the two UVB-exposed groups; p = 0.6085. (C) Average number of malignant (p = 0.5445), benign (p = 0.7446) and non-neoplastic (p = 0.7600) lesions per mouse + SD developing in mice exposed to UVB (blue) and IRA + UVB (yellow). (D) Number of mice per group bearing malignant lesions. Each group consists of 35 mice; UVB vs. IRA + UVB: p = 0.6655. | |
Lesions were classified as non-proliferative, benign and malignant lesions. Non-proliferative lesions comprise erosions and ulcers, necrosis, and inflammatory cell infiltrates. Benign, proliferative lesions incorporate papilloma, cysts and fibroma (representative pictures: ESI Fig. 3;† numbers: Table 1). Epithelial malignant lesions comprise SCCs in three differentiation grades: well differentiated (including keratoacanthoma), moderately differentiated and poorly differentiated (representative pictures: ESI Fig. 4;† numbers: Table 2). Poorly differentiated, spindle-cell shaped SCCs were characterized by the expression of cytokeratins (representative picture: Fig. 2C, F, and I; numbers: Table 2). Non-epithelial malignant lesions were classified as sarcomas according to positive staining for vimentin and negative staining for cytokeratins, further divided into epithelioid-cell shaped and spindle-cell shaped tumours (representative pictures: Fig. 2A, B, D, E, G, and H; numbers: Table 2). Furthermore, we found tumours in the dermis showing spindle cell shaped and fascicularly arranged tumour cells spreading into dermal tissue scattering collagen and fat or muscle, like sarcomas. These dermal tumours were immunohistochemically positive for vimentin, and were furthermore positive for S100 and MelanA (MART1; representative pictures in Fig. 3; numbers: Table 2). Since the morphology was in accordance with positivity for S100 and MelanA, these tumours are most likely of melanocytic origin.
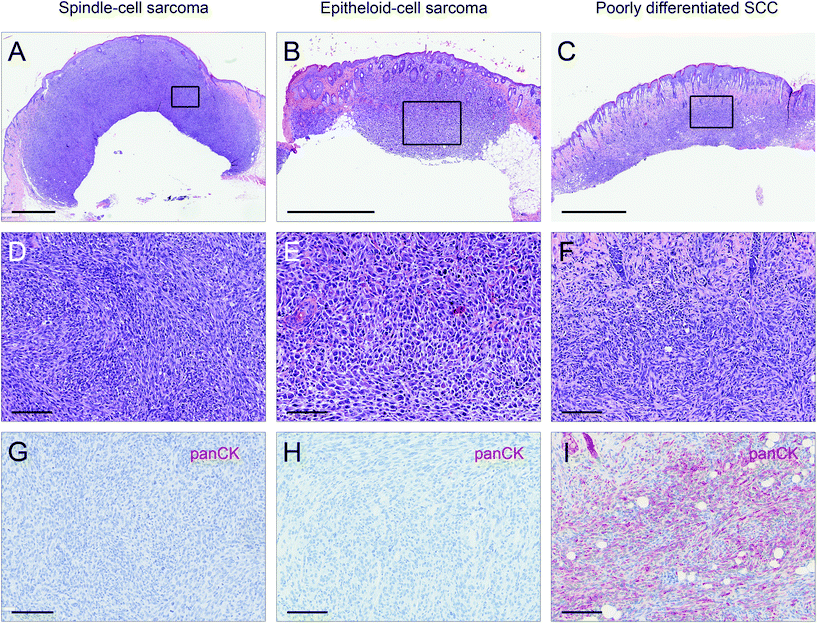 |
| Fig. 2 Representative images of sarcomas as compared to poorly differentiated SCC. (A, D, and G) Representative pictures of a spindle-cell sarcoma with (A and D) showing H&E sections in different magnifications and (G) showing the lesion stains negative for cytokeratins. (B, E, and H) Representative pictures of an epithelioid-cell sarcoma with (B and E) showing H&E sections in different magnifications and (H) showing the lesion stains negative for cytokeratins. (C, F, and I) Representative pictures of a spindle-cell SCC with (C and F) showing H&E sections in different magnifications and (I) showing the lesion stains positive for cytokeratins. Scale bars represent 1000 μm for overview screens (A–C), and 100 μm for detail screens (D–I). | |
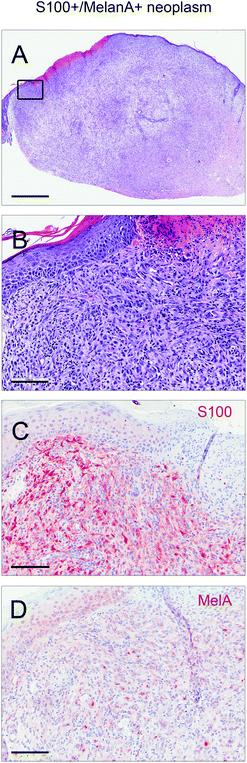 |
| Fig. 3 Representative images of a murine lesion staining positive for S100 and MelanA. (A, B) H&E stainings in different magnifications. (C) S100 staining of the same lesion. (D) MelanA staining of the same lesion. Scale bars represent 1000 μm for overview screen (A), and 100 μm for detail screens (B–D). | |
Table 1 Cumulative number of benign skin lesions arising in mice exposed to UVB or IRA + UVB
Benign lesions |
|
IRA + UVB |
UVB |
Papillomas |
27 |
27 |
Epithelial cysts |
2 |
0 |
Fibromas |
4 |
2 |
Table 2 Cumulative number of malignant skin lesions arising in mice exposed to UVB or IRA + UVB
Malignant lesions |
|
IRA + UVB |
UVB |
Squamous cell carcinomas
|
39 |
41 |
Well differentiated |
30 |
23 |
Moderately differentiated |
5 |
15 |
Poorly differentiated |
4 |
3 |
|
Sarcomas
|
37 |
28 |
Spindle-cell shaped |
23 |
7 |
Epithelioid-cell shaped |
2 |
8 |
Mixed phenotype |
12 |
13 |
|
S100+/MelanA+ neoplasms
|
7 |
2 |
Impact of IRA on UVB-induced lesions in C57BL/6 mice
We were not able to detect any statistically significant differences in lesion free (non-proliferative + benign + malignant lesions; ESI Fig. 1A†), proliferative lesion free (benign + malignant lesions; Fig. 1A) and malignant lesion free (Fig. 1B) survival between mice exposed to UVB and mice exposed to IRA + UVB. In addition, no significant differences in the number of lesions arising per treatment group were detected (insets of Fig. 1A, B, and C). No malignant lesion was observed in eight mice of the IRA + UVB group and twelve mice of the UVB group (number of tumour bearing mice: Fig. 1D).
Impact of IRA on UVB-induced non-epithelial tumours in C57BL/6 mice
While in our study malignant epithelial lesions were equally distributed between IRA + UVB and UVB-exposed mice, malignant non-epithelial lesions were remarkably more frequently observed in IRA + UVB-exposed mice (Fig. 4A). Moreover, a Kaplan–Meier-analysis revealed the trend that malignant non-epithelial tumours not only were more frequent but also developed earlier in IRA + UVB-exposed mice as compared to UVB only exposed animals (Fig. 4B). Using the Nelson–Aalen estimator we estimated the expected number of non-epithelial malignant lesions for UVB and IRA + UVB-exposed mice. We observed a trend towards more non-epithelial malignant lesions in the IRA + UVB-exposed group (Fig. 4C).
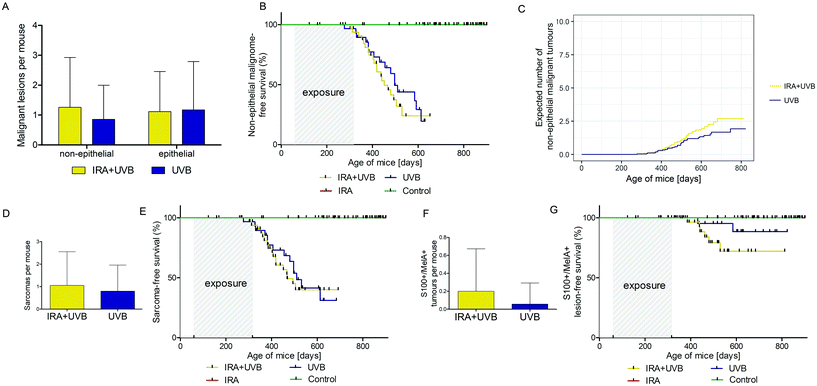 |
| Fig. 4 Epithelial and non-epithelial malignant lesions arising in UVB and IRA + UVB-exposed mice. (A) Average number + SD of epithelial (p = 0.8728) and non-epithelial (p = 0.2459) malignant lesions detected per mouse exposed to UVB (blue) and IRA + UVB (yellow). (B) Kaplan–Meier-curve of non-epithelial malignome-free survival of mice exposed to UVB (blue), IRA + UVB (yellow) and IRA (red), and unexposed controls (green); UVB vs. IRA + UVB: p = 0.3889. (C) Expected cumulative number of non-epithelial malignant tumours developing in mice exposed to UVB (blue) and IRA + UVB (yellow) as estimated by the Nelson–Aalen-estimator; UVB vs. IRA + UVB: p = 0.2100. (D) Average number + SD of sarcomas developing per mouse exposed to UVB (blue) and IRA + UVB (yellow); p = 0.4237. (E) Kaplan–Meier-curve of sarcoma-free survival of mice exposed to UVB (blue), IRA + UVB (yellow) and IRA (red), and unexposed controls (green); UVB vs. IRA + UVB: p = 0.6847. (F) Average number + SD of malignant S100+/MelanA+ lesions developing per mouse exposed to UVB (blue) and IRA + UVB (yellow); p = 0.1142. (G) Kaplan–Meier-curve of malignant lesion staining positive for S100 and MelanA. The survival curve for mice exposed to UVB is indicated in blue, for mice exposed to IRA + UVB is indicated in yellow, for mice exposed to IRA is indicated in red and for non-exposed mice is indicated in green; UVB vs. IRA + UVB: p = 0.1264. | |
Although we found high quantities of sarcomas among the malignant tumours in all mice, animals exposed to both IRA and UVB developed more sarcomas than those exposed to UVB only (Fig. 4D). A Kaplan–Maier-plot for sarcoma-free survival revealed the tendency that IRA drives an earlier, though not significantly different, development of UVB-induced sarcomas (Fig. 4E).
We did not classify nine tumours as sarcoma or SCC. IHC revealed that they were positive for S100 and MelanA. The majority of these supposedly melanocytic tumours (7/9) arose in the IRA + UVB-exposed group, while only 2/9 developed in the UVB only exposed group (Fig. 4F). A Kaplan–Maier analysis revealed that these supposedly melanocytic neoplasms developed earlier in the IRA + UVB than in the UVB-exposed group (Fig. 4G).
Influence of IRA on photocarcinogenesis in male and female mice
Investigating the differential influence of IRA exposure on genders, we did not observe a statistically significant difference in tumour development between mice exposed to UVB and mice exposed to IRA + UVB (data not shown). In order to focus on differences between sexes with respect to UVB-induced carcinogenesis, mice exposed to UVB and IRA + UVB were pooled and control mice and mice exposed to IRA only were excluded. Whereas the onset of all lesions (non-proliferative + benign + malignant lesions; Fig. 5A) and of all proliferative lesions (benign + malignant lesions; Fig. 5B) did not significantly differ between female and male mice, we observed a tendency of an earlier onset of malignant lesions in male mice (Fig. 5C). Interestingly, the gender difference turned out to be statistically significant when the onset of non-epithelial malignant lesions in male and female mice was compared: non-epithelial malignant lesions developed significantly earlier and in higher numbers in male mice than in their female counterparts (Fig. 5D and E). The number of non-proliferative and benign lesions arising per mouse was not significantly different between sexes (Fig. 5E). Similarly, the expected cumulative number of non-epithelial malignant tumours per mouse as calculated using the Nelson–Aalen estimator was significantly higher in males than that in females (Fig. 5F).
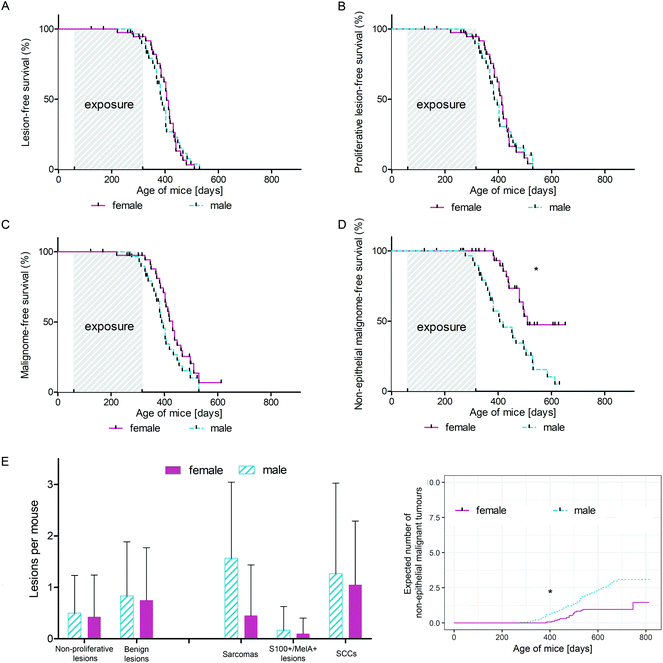 |
| Fig. 5 Gender differences in photocarcinogenesis of wild type C57BL/6 mice. (A) Kaplan–Meier-curve of lesion-free survival of mice exposed to UVB and IRA + UVB. The solid, pink line represents female animals, and the dashed, turquoise line represents male animals; p = 0.7857. (B) Kaplan–Meier-curve of proliferative lesion-free survival of mice exposed to UVB and IRA + UVB. The solid, pink line represents female animals, and the dashed, turquoise line represents male animals; p = 0.7817. (C) Kaplan–Meier-curve of malignome-free survival of mice exposed to UVB and IRA + UVB. The solid, pink line represents female animals, and the dashed, turquoise line represents male animals; p = 0.1258. (D) Kaplan–Meier-curve of non-epithelial malignome-free survival of mice exposed to UVB and IRA + UVB. The solid, pink line represents female animals, and the dashed, turquoise line represents male animals; * p = 0.0011. (E) Average number of lesions per mouse + SD, divided by gender. (F) Expected cumulative number of non-epithelial malignant tumours developing in mice exposed to UVB and IRA + UVB as estimated by the Nelson–Aalen-estimator. The solid, pink line represents female animals, and the dashed, turquoise line represents male animals; * p = 0.0014. | |
Discussion
Each exposure to terrestrial solar radiation does not only imply irradiation with UVR but also to a great extent IR, as IR comprises around 50% of terrestrial solar irradiance.1 In addition, there are numerous artificial IR sources emitting high quantities of IR which are used for wellness, cosmetic or medical purposes. Thus, it is quite interesting that biological effects of this part of the solar optical spectrum are poorly investigated. The aim of this project was to study the impact of IRA on photocarcinogenesis using male and female wild type mice.
The quantitation of the outcome of photocarcinogenesis experiments is a challenging task. Attaining statistical significance in terms of p values lower than 0.05 is even more difficult. This is, amongst other reasons, due to the fact that animal numbers should be kept as low as possible. Although we do not reach significant differences considering the primary endpoint of this study, the tumour numbers and growth patterns confirm the conclusions of earlier studies addressing similar questions.13
UVB induces skin cancer both in wild type mice and mice carrying mutations at specific loci/in particular tissues. IRA is believed to be non-tumourigenic. However, a previous study demonstrated that IRA, although delaying UVB-induced carcinogenesis, promotes a more aggressive tumour phenotype.13 In the present study similar numbers of benign and malignant epithelial lesions were found in mice exposed to UVB and IRA + UVB. However, IRA led to an increase of non-epithelial malignant tumours histomorphologically classified as sarcomas, as well as S100- and MelanA-expressing spindle cell tumours being very likely of melanocytic origin. Sarcomas were immunohistochemically identified by positivity for vimentin lacking staining of cytokeratin. Particularly tumours in the IRA + UVB group (high numbers of sarcomas, as compared to SCCs) strongly resembled those observed by Schanke et al. in a hairless mouse model upon intermittent high dose UVB exposures. Noteworthily, on the other hand, Schanke et al. observed that low chronic UVB exposure resulted in higher numbers of better differentiated epithelial tumours.32 The treatment regimen we applied resembled more the intermittent high dose protocol than the chronic low dose protocol by Schanke et al., and thereby confirms their hypothesis that intermittent high dose UVB exposure might lead to more aggressive tumours.
In humans, cutaneous sarcomas are very rare33 whereas in C57BL/6 mice sarcomas of the skin are quite frequently described upon long-term UVR-exposure. In some studies they even represent the most common UVR-induced skin cancer entity.34 The fact that UVR induces cutaneous sarcomas to a higher extent in mice than in humans might partly be explained by the thinner epidermis of dorsal rodent skin (two layers, ∼30 μm) as compared to human skin (five cell layers, ∼70 μm), leading to a higher exposure of fibroblasts and myofibroblasts.35 However, Morison and colleagues showed in a study irradiating haired mice to UVB, that a big portion of UV-induced, cutaneous, spindle shaped tumours might be squamous cell carcinomas.36 Therefore, we cannot rule out that at least some of the sarcomatous lesions indeed are highly dedifferentiated SCC after the epithelial-to-mesenchymal transition (EMT). Since these lesions exhibited no cytokeratin staining at all, we cannot confirm this hypothesis either. However, if some of the proposed sarcomas are in truth poorly differentiated SCCs, the major conclusion would not be changed: IRA seems to lead to a more aggressive form of UVB-induced skin cancers. The second non-epithelial tumour entity detected in this study was a type of spindle-shaped, proliferative lesion, expressing both S100 and MelanA. According to this immunohistochemical pattern these neoplasms are most likely melanomas, although we cannot rule out that the lesions are atypical fibroxanthomas and/or scars.37,38 While seven of these lesions developed in the IRA + UVB-group, only two developed in UVB-exposed mice (Table 2). The supposedly melanocytic origin of the observed UVB-induced S100+ and MelanA+ spindle-shaped malignant tumours is quite surprising as it is widely believed that UVR cannot induce melanomas in wild type mice. In this context it is noteworthy that in a recent paper we demonstrated in vitro that IRA might contribute to malignant transformation of melanocytes as it facilitates the survival of melanocytes carrying UVB-induced DNA damage.8 Furthermore, the present data are in line with our previous in vivo photocarcinogenesis study in which we observed two tumours diagnosed as melanomas in C57BL/6 wild type mice pre-irradiated with IRA prior to UVB (13% of all arising tumours in this group).13 The assumption of a melanocytic origin of the UVB-induced S100+ and MelanA+ spindle-shaped malignant tumours observed in this study is further supported by a recent publication of our group. Therein we propose that via the induction of interfollicular melanocytes UVR might be capable of inducing melanomas in wild type C57BL/6 mice.39
We observed that IRA did not differently affect UVB-induced skin carcinogenesis in male and female mice. Furthermore, unlike earlier studies investigating gender differences in the photocarcinogenesis of mice, we observed no significant differences between proliferative epithelial lesions arising in males and females. While in hairless albino Skh-1 mice males seem to develop epithelial tumours earlier,18 in the present study female mice developed epithelial lesions slightly earlier (ESI Fig. 5A and B†). On the other hand, male C57BL/6N mice developed more and earlier non-epithelial malignant lesions. As mentioned earlier, we cannot rule out that at least some of the supposed sarcomas represent poorly differentiated SCCs. Assuming this, we observe a higher hazard rate for ‘poorly differentiated spindle-shaped tumours’ in male mice, depicting the most aggressive form of observed lesions. This is in line with epidemiologic studies in humans demonstrating that skin tumours in men have a worse prognosis.14–16 Possible explanations for the more aggressive phenotype of lesions arising in male mice are that oestrogen protects against malignant transformation of skin cells.19 Moreover, the increased susceptibility to spindle-shaped tumours of male mice could be mediated by oxidative DNA damage due to decreased catalase activity in male skin.40 Furthermore, concerning the high proportion of sarcomas in male mice as compared to female mice, the decreased epidermal and increased dermal thickness in the skin of male mice might contribute to this phenomenon.41
In mouse skin carcinogenesis research, the usual approach is to use transgenic animal models and/or chemical tumour induction. However, we decided to use a wild type model of UVR-induced skin carcinogenesis, as (1) genetic alterations can affect several organs, not only the skin, (2) tissue-specific over-expressing a certain factor in the skin leads to non-natural preconditions, (3) many transgenic mouse models lack an effective immunosurveillance (4) and UVR is the predominating carcinogen skin cells are exposed to. As pigmentation is an important and highly discussed characteristic in skin cancer aetiology we chose a pigmented, wild type mouse model, C57BL/6N.
Conclusions
In this study we show that simultaneous exposures of wild type mice to IRA and UVB might lead to an increased hazard rate for aggressive skin tumours as compared to exposure to UVB alone. Noteworthily, compared to UVB only irradiated mice, more Melan-A + S100+ malignant spindle cell tumours develop in IRA + UVB exposed animals.
In addition, male mice seem to be more susceptible to poorly differentiated spindle cell tumours induced by UVB than females.
Abbreviations
IR | Infrared radiation |
IRA | Infrared A radiation |
H&E | Haematoxylin and eosin |
NMSC | Non-melanoma skin cancer |
SCC | Squamous cell carcinoma |
UVB | Ultraviolet B radiation |
UVR | Ultraviolet radiation |
Conflicts of interest
There are no conflicts to declare.
Acknowledgements
We thank Ruth Dingelmaier-Hovorka and Ulrike Mann for excellent technical assistance. This work was supported by the Austrian Science Fund (FWF, I 1204-B23).
References
- S. M. Schieke, P. Schroeder and J. Krutmann, Cutaneous effects of infrared radiation: from clinical observations to molecular response mechanisms, Photodermatol., Photoimmunol. Photomed., 2003, 19, 228–234 CrossRef CAS.
- P. Schroeder, C. Pohl, C. Calles, C. Marks, S. Wild and J. Krutmann, Cellular response to infrared radiation involves retrograde mitochondrial signaling, Free Radicals Biol. Med., 2007, 43, 128–135 CrossRef CAS PubMed.
- P. Sowa, J. Rutkowska-Talipska, K. Rutkowski, B. Kosztyła-Hojna and R. Rutkowski, Optical radiation in modern medicine, Adv. Dermatol. Allergol., 2013, 4, 246–251 CrossRef PubMed.
- S. Schieke, H. Stege, V. Kurten, S. Grether-Beck, H. Sies and J. Krutmann, Infrared-A radiation-induced matrix metalloproteinase 1 expression is mediated through extracellular signal-regulated kinase 1/2 activation in human dermal fibroblasts, J. Invest. Dermatol., 2002, 119, 1323–1329 CrossRef CAS PubMed.
- S. Frank, S. Menezes, C. Lebreton-De Coster, M. Oster, L. Dubertret and B. Coulomb, Infrared radiation induces the p53 signaling pathway: role in infrared prevention of ultraviolet B toxicity, Exp. Dermatol., 2006, 15, 130–137 CrossRef CAS PubMed.
- C. Jantschitsch, S. Majewski, A. Maeda, T. Schwarz and A. Schwarz, Infrared radiation confers resistance to UV-induced apoptosis via reduction of DNA damage and upregulation of antiapoptotic proteins, J. Invest. Dermatol., 2009, 129, 1271–1279 CrossRef CAS PubMed.
- C. Calles, M. Schneider, F. Macaluso, T. Benesova, J. Krutmann and P. Schroeder, Infrared A radiation influences the skin fibroblast transcriptome: mechanisms and consequences, J. Invest. Dermatol., 2010, 130, 1524–1536 CrossRef CAS PubMed.
- S. Kimeswenger, A. Schwarz, D. Födinger, S. Müller, H. Pehamberger, T. Schwarz and C. Jantschitsch, Infrared A radiation promotes survival of human melanocytes carrying ultraviolet radiation-induced DNA damage, Exp. Dermatol., 2016, 25, 447–452 CrossRef CAS PubMed.
- L. H. Kligman, Intensification of ultraviolet-induced dermal damage by infrared radiation, Arch. Dermatol. Res., 1982, 272, 229–238 CrossRef CAS PubMed.
- S. Menezes, B. Coulomb, C. Lebreton and L. Dubertret, Non-coherent near infrared radiation protects normal human dermal fibroblasts from solar ultraviolet toxicity, J. Invest. Dermatol., 1998, 111, 629–633 CrossRef CAS PubMed.
- R. Glaser, F. Navid, W. Schuller, C. Jantschitsch, J. Harder, J. M. Schroder, A. Schwarz and T. Schwarz, UV-B radiation induces the expression of antimicrobial peptides in human keratinocytes in vitro and in vivo, J. Allergy Clin. Immunol., 2009, 123, 1117–1123 CrossRef PubMed.
- C. Skobowiat and A. T. Slominski, Sun-derived infrared A and ultraviolet B radiation: allies or enemies in melanomagenesis?, Exp. Dermatol., 2016, 25, 760–762 CrossRef PubMed.
- C. Jantschitsch, M. Weichenthal, A. Maeda, E. Proksch, T. Schwarz and A. Schwarz, Infrared radiation does not enhance the frequency of ultraviolet radiation-induced skin tumors, but their growth behaviour in mice, Exp. Dermatol., 2011, 20, 346–350 CrossRef PubMed.
- B. Kozma and M. J. Eide, Photocarcinogenesis: An epidemiologic perspective on ultraviolet light and skin cancer, Dermatol. Clin., 2014, 32, 301–313 CrossRef CAS PubMed.
- J. Duran, F. C. Morgan, P. S. Karia and C. D. Schmults, An evaluation of high-stage cutaneous squamous cell carcinoma outcomes by gender, Br. J. Dermatol., 2016, 10–12 Search PubMed.
- S. Gupta, M. Artomov, W. Goggins, M. Daly and H. Tsao, Gender disparity and mutation burden in metastatic melanoma, J. Natl. Cancer Inst., 2015, 107, 10–13 CrossRef PubMed.
- B. Y. Kong, I. M. Haugh, B. J. Schlosser, S. Getsios and A. S. Paller, Mind the Gap: Sex Bias
in Basic Skin Research, J. Invest. Dermatol., 2016, 136, 12–14 CrossRef CAS PubMed.
- J. M. Thomas-Ahner, B. C. Wulff, K. L. Tober, D. F. Kusewitt, J. A. Riggenbach and T. M. Oberyszyn, Gender differences in UVB-induced skin carcinogenesis, inflammation, and DNA damage, Cancer Res., 2007, 67, 3468–3474 CrossRef CAS PubMed.
- M. Mancuso, D. Gallo, S. Leonardi, M. Pierdomenico, E. Pasquali, L. De Stefano, S. Rebessi, M. Tanori, G. Scambia, V. Di Majo, V. Covelli, S. Pazzaglia and A. Saran, Modulation of basal and squamous cell carcinoma by endogenous estrogen in mouse models of skin cancer, Carcinogenesis, 2009, 30, 340–347 CrossRef CAS PubMed.
- Y. Xue, L. Ying, R. L. Horst, G. Watson and D. Goltzman, Androgens Attenuate Vitamin D Production Induced by UVB Irradiation of Skin of Male Mice by an Enzymatic Mechanism, J. Invest. Dermatol., 2015, 135, 3125–3132 CrossRef CAS PubMed.
- S. Okazaki, Y. Funasaka, K. Wakamatsu, S. Kawana and H. Saeki, Effect of infrared radiation A on photoaged hairless mice harboring eumelanin and pheomelanin in the epidermis, J. Dermatol., 2015, 42, 382–390 CrossRef CAS PubMed.
- T. Hirobe, Control of melanocyte proliferation and differentiation in the mouse epidermis, Pigm. Cell Res., 1992, 5, 1–11 CrossRef CAS.
- A. Wolnicka-Glubisz and F. P. Noonan, Neonatal susceptibility to UV induced cutaneous malignant melanoma in a mouse model, Photochem. Photobiol. Sci., 2006, 5, 254–260 RSC.
- C. C. Solomon, E. White, A. R. Kristal and T. Vaughan, Melanoma and lifetime UV radiation, Cancer Causes Control, 2004, 15, 893–902 CrossRef PubMed.
- R. Zanetti, S. Franceschi, S. Rosso, S. Colonna and E. Bidoli, Cutaneous melanoma and sunburns in childhood in a southern European population, Eur. J. Cancer, Part A, 1992, 28, 1172–1176 CrossRef.
- J. A. Lo and D. E. Fisher, The melanoma revolution: from UV carcinogenesis to a new era in therapeutics, Science, 2014, 346, 945–949 CrossRef CAS PubMed.
- F. P. Noonan, J. A. Recio, H. Takayama, P. Duray, M. R. Anver, W. L. Rush, E. C. De Fabo and G. Merlino, Neonatal sunburn and melanoma in mice, Nature, 2001, 413, 271–272 CrossRef CAS PubMed.
-
RDevelopmentCoreTeam, R Foundation for Statistical Computing, Vienna, Austria, 2008 Search PubMed.
-
Ø. Borgan, Nelson-Aalen Estimator, in Encyclopedia of Biostatistics 2005 Search PubMed.
- L. D. A. F. Amorim and J. Cai, Modelling recurrent events: A tutorial for analysis in epidemiology, Int. J. Epidemiol., 2015, 44, 324–333 CrossRef PubMed.
- T. M. Therneau, A Package for Survival Analysis in S, Mayo Clin. Found., 1999, 70–73 Search PubMed.
- A. Van Schanke, G. M. C. A. L. van Venrooij, M. J. Jongsma, H. A. Banus, L. H. F. Mullenders, H. J. Van Kranen and F. R. De Gruijl, Induction of Nevi and Skin Tumors in Ink4a/Arf Xpa Knockout Mice by Neonatal, Intermittent, or Chronic UVB Exposures, Cancer Res., 2006, 2608–2616 CrossRef CAS PubMed.
- B. Aigner, S. Ugurel, S. Kaddu and J. C. Becker, Kutane Sarkome, Der Hautarzt, 2014, 65, 614–622 CrossRef CAS PubMed.
- M. L. Kripke, Latency, Histology, and Antigenicity of Tumors Induced by Ultraviolet Light in Three Inbred Mouse Strains, Cancer Res., 1977, 37, 1395–1400 CAS.
- F. R. de Gruijl and P. D. Forbes, UV-induced skin cancer in a hairless mouse model, Bioessays, 1995, 17, 651–660 CrossRef CAS PubMed.
- W. L. Morison, M. S. Jerdan, T. L. Hoover and E. R. Farmer, UV radiation-induced tumors in haired mice: identification as squamous cell carcinomas, J. Natl. Cancer Inst., 1986, 77, 1155–1162 CAS.
- C. Thum, K. Hollowood, J. Birch, J. R. Goodlad and T. Brenn, Aberrant Melan-A expression in atypical fibroxanthoma and undifferentiated pleomorphic sarcoma of the skin, J. Cutaneous Pathol., 2011, 38, 954–960 CrossRef PubMed.
- A. Robson, P. Allen and K. Hollowood, S100 expression in cutaneous scars: A potential diagnostic pitfall in the diagnosis of desmoplastic melanoma, Histopathology, 2001, 38, 135–140 CrossRef CAS PubMed.
- D. A. De Luca, B. Sterniczky, S. Kimeswenger, D. Födinger, A. Schwarz, T. Schwarz and C. Jantschitsch, Ultraviolet radiation induces Melan-A-expressing cells in interfollicular epidermis in wild-type mice, Arch. Dermatol. Res., 2018, 310(6), 529–532 CrossRef CAS PubMed.
- N. J. Sullivan, K. L. Tober, E. M. Burns, J. S. Schick, J. A. Riggenbach, T. A. Mace, M. A. Bill, G. S. Young, T. M. Oberyszyn and G. B. Lesinski, Ultraviolet Light B-Mediated Inhibition of Skin Catalase Activity Promotes Gr-1+CD11b+ Myeloid Cell Expansion, J. Invest. Dermatol., 2012, 132, 695–702 CrossRef CAS PubMed.
- L. Azzi, M. El-Alfy, C. Martel and F. Labrie, Gender differences in mouse skin morphology and specific effects of sex steroids and dehydroepiandrosterone, J. Invest. Dermatol., 2005, 124, 22–27 CrossRef CAS PubMed.
Footnote |
† Electronic supplementary information (ESI) available. See DOI: 10.1039/c8pp00118a |
|
This journal is © The Royal Society of Chemistry and Owner Societies 2019 |
Click here to see how this site uses Cookies. View our privacy policy here.