DOI:
10.1039/C8OB02140F
(Review Article)
Org. Biomol. Chem., 2019,
17, 11-23
Non-toxic cyanide sources and cyanating agents
Received
30th August 2018
, Accepted 25th September 2018
First published on 25th September 2018
Abstract
The present review gives an overview over non-toxic cyanation agents and cyanide sources used in the synthesis of structurally diverse products containing the nitrile function. Nucleophilic as well as electrophilic agents/systems that transfer the entire CN-group were taken in consideration. Reactions in which a preexisting carbon functionality is transformed into a nitrile function by addition of nitrogen are however not covered here.
1. Introduction
By treating potassium ferrocyanide with sulfuric acid, the famous Swedish–German chemist Carl Wilhelm Scheele produced a hitherto unknown chemical compound in 1782. Since the hydrogen cyanide evolved under the formation of Prussian blue, he called this new compound “Blausäure” (“blue acid”, prussic acid). Over the years, hydrogen cyanide – free or in form of its salts – became one of the most important C1 building blocks in chemical industry.1 It is essential for the synthesis of α-amino acids like methionine2 or chelators like ETDA,3 NTA4 and is needed for case hardening,5 electroplating6 and the processing of gold ore.7
For chemical companies, the handling of the highly toxic hydrogen cyanide requires closed reactors and substantial safety regulations but is still favored due to cost minimization. For smaller enterprises or research labs, free HCN is often not an option and commercial cyanide sources like metal cyanides, ketone cyanohydrins or trimethylsilyl cyanide are employed instead. Nevertheless, these compounds are still highly toxic and safer alternatives are highly welcome. Over the years, many approaches towards non-toxic alternatives for cyanation reactions have been reported. All these approaches fall into two classes. One class of cyanide sources contains a tightly bound cyano group that needs to be liberated for the reaction to occur. In the other class the cyanide moiety is formed during the reaction from a carbon and a nitrogen atom of any oxidation state.
Several aspects of cyanide sources and cyanating agents have already been discussed in the literature.8 In the focus of these reviews were cyanation reactions with palladium,9,10 copper11,12 or diverse transition metals,13–15 metal free cyanations,16,17 the synthesis of aryl nitriles,18,19 the formation of N/S/O–CN bonds20 or cyanations by C–H activation.21 Here, we intend to give an overview with a particular emphasis on agents that exhibit a low(er) toxicity. Toxic cyanide sources like KCN, NaCN, TMSCN, CuCN, Zn(CN)2, Bu4NCN, malononitriles, DDQ and cyanohydrins are therefore not discussed here.
2. Reagents with tightly bound CN
The first group of non-toxic cyanide sources already contain a CN-group that is tightly bound so that no significant concentrations of cyanide are released from these reagents under normal circumstances. For activation, the X–CN bond needs to be cleaved and the cyanide is transferred to the cyanide acceptor. Depending on the electronegativity of the α-atom and the bonding properties CN-group can be an electrophilic, nucleophilic or radical cyanation agent (Fig. 1).
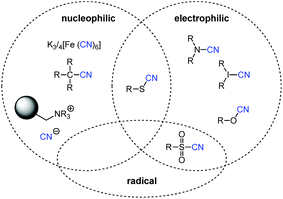 |
| Fig. 1 Overview of the 1. group of non-toxic sources. | |
Ferro-/ferricyanide
In 1877, Merz and Weith heated ferrocyanide and quartz sand in a furnace and passed a stream of vaporized halobenzene through the mixture. The collected condensate contained the corresponding aryl nitrile. Therefore, Merz and Weith were first to use ferrocyanide as a non-toxic cyanide source for a (nucleophilic) cyanation reaction.22 For a long time, the use of these iron complexes as non-toxic cyanide sources lay dormant until Beller and coworkers rediscovered it in form of an efficient palladium-catalyzed reaction at much lower temperature in 2004 (Scheme 1).23 Since then, a huge variety of reactions have been reported, in particular for the synthesis of aromatic nitriles from aromatic compounds containing a good nucleofuge. The reported reactions can be grouped by their reaction temperature and the catalyst used. For temperatures below 70 °C, two palladium catalyzed processes have been reported by Kwong (2011)24 and by Jian Li (2012).25 Some palladium26–32 and one copper33 catalyzed reaction have been reported to operate below 100 °C while the majority of reactions require higher reaction temperatures. Here, reports with palladium,23,34–62 copper,63–72 a palladium/copper co-catalysis,73,74 and nanoparticles or polymers75–92 can be found.
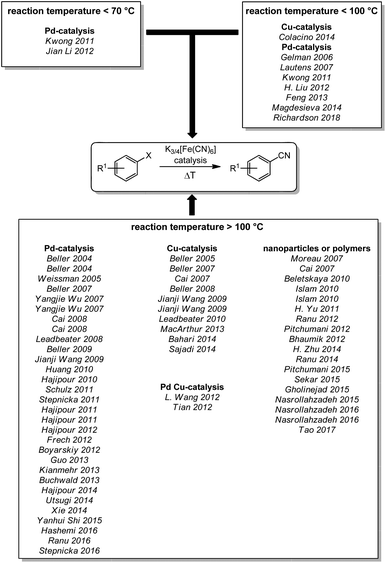 |
| Scheme 1 Cyanation with ferro-/ferricyanide of aromatic compounds containing a nucleofugic substituent. | |
Besides the nucleophilic aromatic cyanation, ferro- and ferricyanide can also be used to convert other aromatic compounds to their corresponding nitriles. In 2010, the Kwong group reported the conversion of mesylates under palladium catalysis with suitable ligands (Scheme 2).93 Recently, Qin and coworkers were able to cyanate phenols by converting the OH-group to a better leaving group with sulfuryl fluoride.94 This reaction might enable an alternative way to use fossil- or biomass-based phenols for the chemical industry, even though DMF was used as a solvent. The conversion of aldehydes to the corresponding nitriles with different nanoparticles and ferricyande have been shown by the groups of Karak (2016)95 and Nasrollahzadeh (2017).96 Unfortunately, they did not investigate the reaction mechanism and it remains unclear if the nitrile is formed by decarbonylation/cyanation or by a nitrogen transfer. In other reports, oxidative cyanations of electron rich benzenes or heterocycles were described as well. The group of Cheng described in 2009 the palladium catalyzed formation of aromatic nitriles from benzene derivatives with stoichiometric quantities of copper(II)salts as an terminal oxidant.97 In 2010, Jianbo Wang and coworkers used this reaction to cyanate indoles in 3-position.98 Since they used ferrocyanide in contrast to Cheng, the equivalents of the copper(II) salt were increased. By adding iodine and using ferricyanide, Jianji Wang was able to drastically decrease the copper equivalents again.99
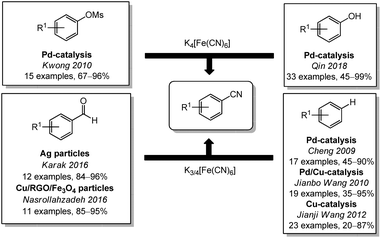 |
| Scheme 2 Cyanation of different aromatic compounds with ferro-/ferricyanide, RGO = reduced graphene oxide.100 | |
The cyanation of aliphatic compounds with ferro- or ferricyanide was investigated less extensively but some examples were reported nevertheless. The group of Jianji Wang reported in 2011 and 2012 the cyanation of different benzylic chlorides by palladium and copper catalysis (Scheme 3).101,102 Other leaving groups in α-carbonyl- or benzylic-position can be substituted by cyanide as shown by Gribble (2013)103 and Z. Li (2014).104 More recently, it was demonstrated that tertiary amines can be used in oxidative cyanation to produce α-amino nitriles. The Opatz group reported an environmentally benign thermal cyanation of different N-arylamines in water/alcohol mixtures in 2015105 and the conversion of aliphatic amines in a catalyst-free photooxidation in 2017.106 The addition to electrophilic C
C double bonds, carbonyl groups, imines or iminium ions have been studied by J. Zhu in 2007,107 by Z. Li et al. (2010–2014)108–112 as well as by Opatz et al. since 2015.105,106,113 Very recently, Bolm and coworkers were the first to report a Strecker reaction with ferric-/ferrocyanide or Prussian blue in a ball mill.114
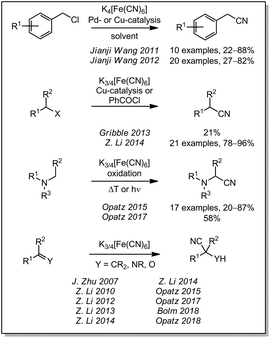 |
| Scheme 3 Cyanation of different aromatic compounds with ferro-/ferricyanide. | |
Nitriles
Nitriles are another compound class containing strongly bound cyano groups. In contrast to the coordinative bonding in metal complexes, a covalent C–C bond prevents the undesired spontaneous liberation of cyanide. Due to the high dissociation energy of this bond, most reactions require harsh conditions to proceed. The first report on the use of nitriles as a cyanide source was published in 1998 by C.-H. Cheng and coworkers (Scheme 4). They described the formation of aromatic nitriles from aryl bromines with benzyl cyanide or acetonitrile with an excess of zinc and in the presence of palladium or nickel catalysts.115 Since 2012, the cyanation with nitriles has moved into the focus of several research groups. In 2012 and 2013, Y. Wang worked on the cyanation with benzyl cyanide and was able to showcase the cyanation of aryl halides by palladium- or copper-catalysis,116,117 as well as the oxidative cyanation of 2-arylpyridines by copper-catalysis using air as terminal oxidant.118 In parallel, a remarkable synthesis of α-amino nitriles from arylamines with benzyl cyanide and tert-butyl hydroperoxide as an oxidant was reported by Wan (2013).119 Even though the oxidant and the cyanide source are still classified as toxic (although they are less dangerous than free cyanide), the oxidative cyanation of amines is standing out from all other syntheses of aromatic nitriles. While the cleavage of a benzylic nitrile function is aided by the benzylic stabilization of reaction intermediates, the activation of aliphatic nitriles is even more challenging. In 2012, the cyanation of aryl iodides with acetonitrile through copper-catalysis in the presence of silver oxide and air was reported by Jin-Heng Li.120 Based on this publication, Jizhen Li extended the reaction to arenediazonium salts in 2015.121 Apart from functionalized arenes carrying a leaving group, unsubstituted aromatic or heteroaromatic compounds can also be cyanated by copper catalysis in the presence of oxygen and N-iodosuccinimide or silver salts. These reactions were reported by C. Zhu in 2013122 and by Shen in 2013 and 2015.123–125 Just recently, the Opatz group described a new strategy to use benzylic and aliphatic nitriles as universal cyanide sources through activation by manganese catalysis. In this reaction, the nitriles were oxidized to their corresponding cyanohydrins with peroxodisulfate and cleaved under acidic conditions to release HCN.113 Another nitrile used for cyanation is the relatively inexpensive ethyl cyanoacetate. This cyanide source has been used by Shen et al. in a palladium-catalysis of aryl halides to yield the aryl nitriles in 2012.126 Diaminomaleonitrile (DAMN, the tetramer of HCN) has also been used as a cyanide source in a palladium-catalysis by Goswami in 2013.127 It should however be noted that the price of DAMN is substantial.
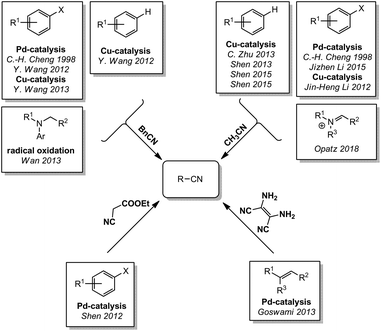 |
| Scheme 4 Cyanation of different compounds with nitriles. | |
Cyanates
Another class of cyanating agents are cyano chalcogenides such as cyanate and thiocyanate. In 1992, the Niclas group reported the first acidic transfer cyanation with aluminium chloride and hydrogen chloride for the cyanation of arenes with aryl cyanates (Scheme 5).128 This idea was used by Sato and coworkers who described the cyanation of different aromatic compounds by initial lithiation with n/tbutyl lithium and subsequent reaction with phenyl cyanate in 2002 and 2003.129,130
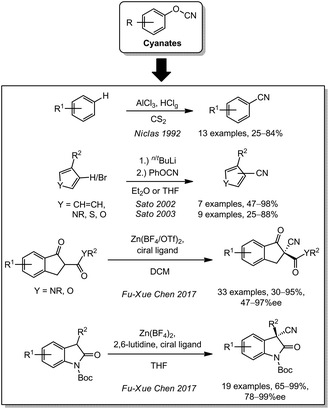 |
| Scheme 5 Cyanation of different compounds with cyanates. | |
Since aryl cyanates are usually synthesized from phenols and cyanogen bromide (which is substantially more expensive and more toxic than free cyanide),131 this compound class was not widely applied. Notably, Fu-Xue Chen used 4-acetylphenyl cyanate in 2017 for an asymmetric cyanation of 1,3-dicarbonyls132 and amides.133
Thiocyanates
Compared to the cyanates, organic and inorganic thiocyanates have attracted more attention as cyanide sources. In 1977, Kita and coworkers reported on thiocyanations of different aromatic and aliphatic alcohols with Ph3P(SCN)2 (Scheme 6). Under identical conditions, they however observed the formation of aryl nitriles for indoles and pyrrole in good yields.134 Six years later, Cava used 2-chlorobenzyl thiocyanate for the synthesis of 2-arylmalononitriles from aryl nitriles.135 More recently arylboronic acids and aryl halides were cyanated under palladium catalysis with equimolar amounts of copper(I) thiophene-2-carboxylate and organic or inorganic thiocyanates by the groups of Liebeskind (2006),136,137 J. Cheng (2013)138 and Vaghasiya (2018).139 Based on the work of Wan (2013),119 Ofial and coworkers reported a catalyst-free synthesis of α-amino nitriles from tertiary amines and potassium thiocyanate by oxidation with tert-butyl hydroperoxide in 2015.140 Even though the oxidant is classified as toxic, the application of KSCN instead of the more expensive benzyl cyanide119 is a significant improvement of this reaction. In 2017, the Jiang group presented a copper-catalyzed benzylic cyanation of N-tosylhydrazones which enables a unique two-step reductive cyanation of ketones or aldehydes.141
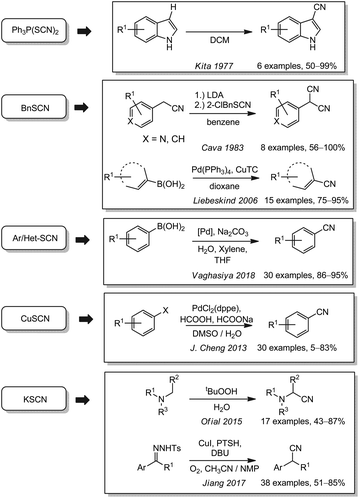 |
| Scheme 6 Cyanation of different compounds with thiocyanates. | |
Tosyl cyanide
In 2011, Inoue reported an oxidative photocyanation with benzophenone as an organic photocatalyst. Via a PHAT (photon induced hydrogen abstraction)-mechanism, ethers, alcohols, carbamates and aliphatic compounds were successfully cyanated using tosyl cyanide as the CN source (Scheme 7).142,143 Apart from this process involving free radicals, TsCN can also be used as an electrophilic cyanating agent. As an example, the group of Ibrahim demonstrated an electrophilic cyanation of 1,3-dicarbonlys under basic conditions in 2013.144
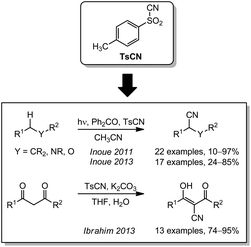 |
| Scheme 7 Cyanation of different compounds with tosyl cyanide. | |
CN-containing iodine(III) compounds
Some cyanation reactions were also performed with different CN-iodine species, like cyanobenziodoxoles or the more reactive aryl(cyano)-iodonium triflates. In 1995, the Zhdankin lab used for the first time cyanobenziodoxoles as electrophilic cyanation agents for the conversion of dimethylanilines under basic conditions (Scheme 8).145 It took approximately 20 years before the use of these agents was reported again. The groups of M. Waser (2015),146 Zheng (2015),147 and X. Liu (2017)148 described a enantioselective α-cyanation of β-keto esters with different chiral ligands. Fu-Xue Chen (2015) described a racemic variant without chiral ligands.149 Cyanobenziodoxoles were also applied for the cyanation of thiophenes by J. Waser in 2015150 and of silyl enol ethers by Minakata in 2017.151
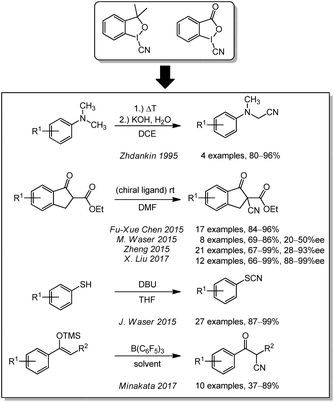 |
| Scheme 8 Cyanation of different compounds with cyanobenziodoxoles. | |
The more reactive aryl(cyano)iodonium triflates developed by Zhdankin and Stang152,153 have been further studied by Jianbo Wang (2014),154 L. Shi (2015)155 and Studer (2016)156 Unfortunately, they are not yet classified in terms of toxicity and are therefore not discussed in further detail here.
Cyanamides
Electrophilic cyanation can also be performed using cyanamide derivatives. One of the most important reagents of this class is N-cyano-N-phenyl-p-toluenesulfonamide (NCTS). This cyanating agent can be synthesized by a procedure of Kurzer et al. from N-phenylurea and p-toluenesulfonyl chloride.157–159 In 2011, Beller reported for the first time the application of NCTS as a non-toxic electrophilic cyanating agent (Scheme 9). It was used for the synthesis of aromatic nitriles from aryl bromides through the intermediate formation of Grignard reagents160 or from arylboronic acids through rhodium catalysis.161 In parallel, Jianbo Wang et al. were also able to cyanate indoles and pyrroles using a Lewis acid as the catalyst.162 In the following years, a variety of different catalysts were used for the cyanation of different aromatic compounds with NCTS by cleaving C–H or C–halogen bonds. In 2013, Anbarasan presented the cyanation of aromatic C–H bonds with a ruthenium catalyst using AgSbF6.163 Based on this research, Glorius and coworkers optimized the reaction and were able to exchange the catalyst for a cobalt complex in 2014.164 Buchwald published a copper-catalyzed cyanation of β-vinylnaphthalene derivatives with (BPin)2 in the same year.165 His former co-worker Yang continued the work (P. Liu, 2015) in this area.166 Gosmini (2015) and Jizhen Li (2016) used NCTS to cyanate aromatic compounds containing leaving groups like bromine or iodine, as well as arenediazonium salts. For this reaction, copper- or palladium-catalysis in combination with zinc or silver oxide were used.167,168 Since these substrates are electrophilic it is understandable that zinc as a reductant enables the cyanation with the electrophilic cyanating agent NCTS. It is less obvious why silver oxide or silver salts in DMSO leads to the same outcome. NCTS can also be used for the α-functionalization of simple and α,β-unsaturated carbonyl compounds as demonstrated by Minakata in 2016.169 In contrast to all these applications, Song and coworkers were able to generate aromatic nitriles from arenecarboxylic acids by a catalytic decarboxylation (2017).170
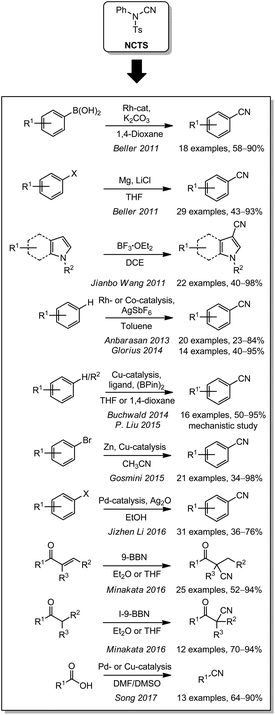 |
| Scheme 9 Cyanation of different compounds with NCTS. | |
Other N–CN-based cyanating agents were also employed in the literature. Unfortunately, they all require prior synthesis from an amine or N-chloroamine with another electrophilic or nucleophilic cyanide source (Scheme 10). As early as in 1985, the Crossley group reported an aliphatic C-cyanation with N,N-diisopropyl cyanamide after deprotonation of a benzylic position.171 In 1998 and 1999, the cyanation of benzylic nitriles with 1-cyanobenzotriazole to form aryl malononitriles under basic conditions was reported by Cava et al.172,173 The Katritzky group also used 1-cyanobenzotriazole as a cyanating agent under basic conditions for benzylic or aliphatic carbon center substituted with an electron withdrawing group in 2007.174 In another approach, N-cyanoimidazole was used by Yong-qian Wu and coworkers in 2000 to achieve the cyanation of amines, thiols, Grignard reagents or alkynes.175 Based on this work, Beller reported in 2010 the cyanation of aryl bromides with magnesium via the Grignard-pathway with the modified cyanating agent N-cyanobenzimidazole.176 A detailed optimization of N-cyanohetarenes was performed by Yoon in 2005. He varied the substitution pattern of 2-cyanopyridazin-3(2H)-ones to cyanate amines, thiols and 1,3-dicarbonyls and found 4,5-dichloro- and 5-chloro-4-methoxy-6-oxopyridazine-1(6H)-carbonitrile to be the optimum cyanating agents.177
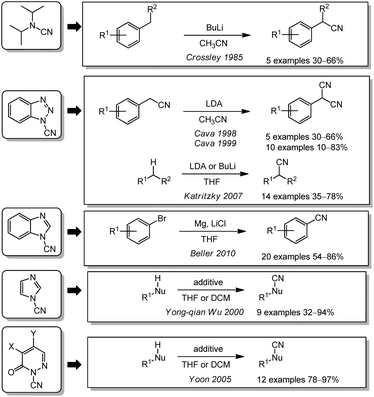 |
| Scheme 10 Cyanation of different compounds with other N–CN sources. | |
Isocyanides
A less frequently used group of alternative cyanating agents are the isocyanides. B. Xu and coworkers used tert-butyl isonitrile for the first time for a cyanation of heteroarenes in a palladium catalysis (Scheme 11).178 Disadvantages of this procedure were the use of copper(II) as terminal oxidant and the low yields for starting materials devoid of electron donating substituents. In 2015, the group of Y.-M. Zhu adopted the procedure for the cyanation of aryl iodides.179 Tosylmethyl isocyanide (TosMIC) and its derivates as less toxic alternatives to tert-butylisonitrile were applied to different 2-bromobenzyl bromides by Vaquero in 2013. In this case, not only the cyano group but also other parts of the cyanating agent are transferred to the substrate and a the structural diversity of the products is larger than with most other procedures.180
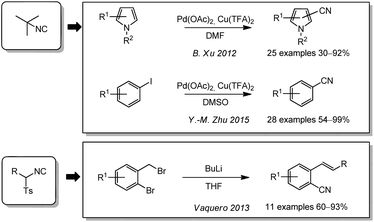 |
| Scheme 11 Cyanation of different compounds with isocyanides. | |
Cyanocarbonyls
Cyanocarbonyls are labile sources of cyanide and can release HCN upon aqueous hydrolysis. This was utilized in parallel by the working groups of Nageswar and Lingaiah in 2012 (Scheme 12).181,182 They used ethyl cyanoformate in combination with TBHP to synthesize α-aminonitriles from N,N-dialkylanilines with ruthenium on charcoal or a niobia-supported iron molybdophosphate as the catalysts. While industry takes specific precautions to handle HCN safely in a well-defined setting, its use in research labs is often refrained from although a safe handling is doubtlessly also possible in this environment. In an attempt to make lab applications of HCN safer, Ley and coworkers reduced the free inventory of this reagent by using a flow setup. In a biocatalytic approach, they achieved the synthesis of chiral O-acetyl cyanohydrins from aldehydes in 2016.183 In 2012, the use of pyruvonitrile as a cyanating agent for synthesis of α-ketiminophosphonates was shown by Palacios.184
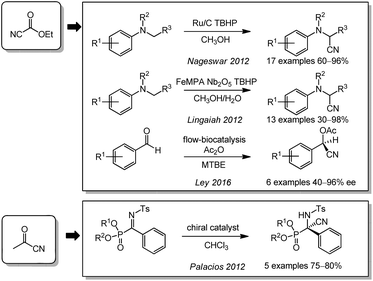 |
| Scheme 12 Cyanation of different compounds with cyanocarbonyles. | |
AIBN
Apart from its well-known application as a radical starter, azobis(isobutyronitrile) (AIBN) and its derivatives can also be used for cyanation reactions. Here, the labile compound breaks down in a radical dediazotation to generate 2-cyanopropan-2-yl radicals. Han and coworkers suggested in a publication of 2013 that these radicals form acetone and cyanide radicals in the presence of oxygen. They described a copper-mediated direct aryl C–H cyanation via this free radical pathway. However the scope was limited to 2-arylpyridines due to the copper coordination by the nitrogen (Scheme 13).185 The idea of a free radical cyanation through AIBN was also proposed by Jiang Cheng in 2014186 for their cleavage of disulfides to form thiocyanates as well as for their synthesis of cyano amides from amines (2015).187 In the same year, X. Xu et al. used AIBN and AMBN (azobisisoamylonitrile) for the cyanation of terminal alkynes. By variation of the equivalents, they were able to either perform the cyanation or an addition of isobutyronitrile.188 He and coworkers optimized the reaction further in 2017 and were able to forgo the use of any metal. They applied their procedure to an oxidative α-cyanation of arylamines.189
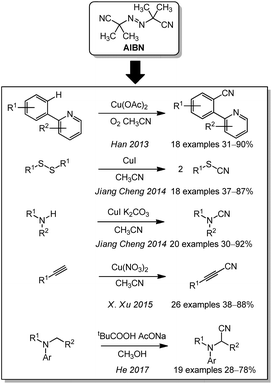 |
| Scheme 13 Cyanation of different compounds with AIBN. | |
Miscellaneous
In other works, insoluble polymers were used as ion exchange resins for nucleophilic cyanations. In 2008, Tajima used a cyanide loaded polystyrene-based ion exchange resin in an electrochemical oxidative cyanation of carbamates, amides, and ethers (Scheme 14).190 Similar resins have been used 2012 by the Ebrahimi group in a Sandmeyer-type deaminative cyanation of anilines through the corresponding diazonium salts.191 A transfer cyanation in which arenes containing a nucleofuge are subjected to an O/C-exchange with α-amino nitriles as cyanide donors was reported by Yamaguchi in 2016. In this nickel-catalyzed process, the starting material can also contain less reactive leaving groups such as carbamates or phenol esters, while an impressive chemoselectivity could be observed.192
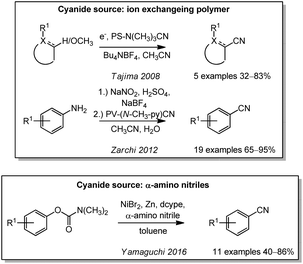 |
| Scheme 14 Cyanation with different cyanide sources. | |
Some less frequently used cyanating agents can also be found in the literature. Many of them have not yet been assessed toxicologically and are not discussed in detail. These include diethyl phosphorocyanidate (DEPC, classified as toxic), 1-cyano-4-dimethylaminopyridinium bromide (CAP, potential BrCN releasing agent), and cyanoethyl zinc bromide (potential cyanide releasing agent). Surprisingly, the latter reagent is only classified as harmful. Two interesting reagents are N-cyanosuccinimide193 and cyano halo (imidazolium) sulfuranes (analogues to the iodine(III) reagents).194 Although not yet investigated, they might turn out to exhibit acceptable toxicity values in the future.
3. Group of non-toxic sources: formation of the C
N-bond
In the second and smaller group of non-toxic cyanide sources, the cyano group is formed during the reaction itself (Scheme 15). Different oxidation states of the carbon and the nitrogen atom source have been employed.
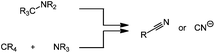 |
| Scheme 15 Schematic representation of the formation of the cyano group from different carbon and nitrogen sources. | |
Formamides
A relatively large group of cyanide sources developed in the last years comprises simple formamides such as DMF or formamide itself. Two major reaction pathways were established for the cyanide formation. In the first case, the cyano carbon originates from the carbonyl group of DMF or formamide and activation with phosphoryl chloride is required. Here, the group of Pelcman described in 1986 the cyanation of electron rich aromatics like indole or veratrole with Viehe's salt, N-(dichloromethylene)dimethyliminium chloride, prepared from DMF (Scheme 16).195 Based on the palladium-catalyzed aminocarbonylation with the Vilsmeier–Haack-reagent, N-(chloromethylene)dimethyliminium phosphorodichloridate, published by Hiyama,196 the group of Bhanage developed palladium- or rhodium-catalyzed nucleophilic cyanations of aryl halides in 2011 and 2013.197,198 By using an analogue of the Vilsmeier reagent synthesized from formamide (N-(chloromethylene)iminium phosphorodichloridate), they were able to achieve a cyanation instead of an amidation.
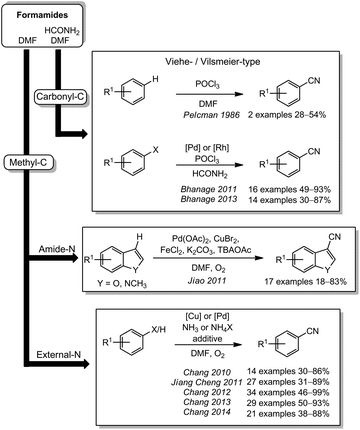 |
| Scheme 16 Cyanation of different compounds with formamides. | |
For DMF, a second reaction pathway is also possible. Here, the methyl group functions as the carbon source for the cyanide generation. The nitrogen can either originate from the DMF itself or from an external source. The former pathway has been reported in 2011 by Jiao and coworkers.199 In a complex catalysis using iron(II), palladium(II), and copper(II), they were able to achieve the electrophilic cyanation of indoles or benzofurans. By isotope labeling, they could prove the origin of the carbon and the nitrogen atom forming the cyano group. In a series of papers, palladium or copper catalysis were used to turn DMF in combination with ammonium salts into a binary cyanide source. The groups of Chang (2010–2014) and J. Cheng (2011) were able to apply this procedure to arenes, heteroarenes,200 aryl halides,201,202 arylboronate esters, arylboronic acids,203 and organosilanes.204 Even though the general procedures of all reports are quite similar (oxygen, 130–150 °C and optionally some additives), the protocol is capable of cyanating both electrophilic and nucleophilic starting materials in very high yields.
Miscellaneous
In 2006, the J.-Q. Yu laboratory investigated a cyanative C–H functionalization of aromatic compounds. After the assumed oxidation of the π-system with air under copper catalysis, they trapped the intermediate radical cation with different nucleophiles. In a blank-experiment, they observed the formation an aryl nitrile without any additional cyanide source in nitromethane as the solvent (Scheme 17).205 In analogy to the cyanations with formamides, DMSO has also been used as a carbon source to form cyanide. By employing ammonium carbonate as the nitrogen source, J. Cheng reported an oxidative cyanation of indoles with dual palladium/copper catalysis.206 Fan Chen and coworkers were able to extend the substrate spectrum to the cyanation of aryl iodides with urea as the CN-nitrogen source in 2013. An extension of the substrate spectrum was achieved by choosing phenanthroline as the ligand on copper. In 13C-labeling experiments, the origin of the carbon could be identified.207 In a recent publication, the Opatz group reported the use of α-amino acids and even protein hydrolysates as a source of HCN with the aid of manganese catalysis. To this end, the amino acids were first converted in their corresponding nitrile by an oxidative decarboxylation with the inexpensive sodium dichloroisocyanurate (NaDCC). In a second step, the nitriles were oxidized with ammonium peroxodisulfate under manganese catalysis to the corresponding cyanohydrins. Their degradation released HCN even under acidic conditions.113
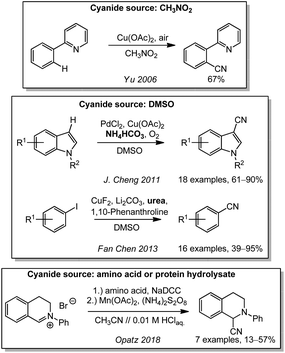 |
| Scheme 17 Cyanations with miscellaneous cyanide sources. | |
Toxicity of cyanide sources and cyanating agents
All reagents and reagent systems described in the present review are less toxic than cyanide itself by definition. They provide additional safety during handling, transportation and often also during their use, while the health risks associated with the respective reagents differ largely. The sources can be subdivided into groups of different toxicity. In the first column of Table 1, sources are listed that are still classified as toxic or at least as harmful. The sources listed in the second column require toxic chemicals for their activation or the overall process. Many of the systems require for their synthesis toxic starting materials such as alkali cyanides or cyanogen halides. These are listed in the third column. Finally, there are reagents or systems that are entirely non-toxic in every way and should be considered as the ideal cyanide sources for future reactions.
Table 1 Overall toxicity of all cyanide sources
Toxic |
Toxic reagents required for activation |
Toxic reagents required for preparation |
Low toxicity |
α-Amino nitriles |
Formamide |
Ferro-/ferricyanide208 |
α-Amino acids/proteins |
Nitromethane |
KSCN |
AIBN |
DMSO |
DMF |
|
TsCN |
NCTS |
PhSCN |
|
PhOCN |
BnSCN |
Ph3P(SCN)2 |
|
NC–CH2–COOEt |
CuSCN |
BnCN |
|
DAMN |
CH3CN |
CH3COCN |
|
Ion exchanging polymer |
Ferro-/ferricyanide209 |
|
|
|
|
Conflicts of interest
There are no conflicts to declare.
Notes and references
-
I. C. M. Code, Use in mining, https://www.cyanidecode.org/cyanide-facts/use-mining, (accessed 01.05.2018).
- T. Willke, Appl. Microbiol. Biotechnol., 2014, 98, 9893–9914 CrossRef CAS.
-
J. R. Hart, in Ullmann's Encyclopedia of Industrial Chemistry, 2000 Search PubMed.
-
C. Gousetis and H.-J. Opgenorth, in Ullmann's Encyclopedia of Industrial Chemistry, 2011 Search PubMed.
-
C. R. Brooks, K. Hacias, R. Hart, D. P. Murphy and M. Petschel, Kirk-Othmer Encyclopedia of Chemical Technology, 1, 2005, vol. 15, pp. 1–34 Search PubMed.
-
Merkblatt über die besten verfügbaren Techniken für die Herstellung anorganischer Spezialchemikalien, Umweltbundesamt, German Federal Environmental Agency, Dessau, 2007 Search PubMed.
-
L. D. Pesce, Kirk-Othmer Encyclopedia of Chemical Technology, 1, 2000, vol. 8, pp. 171–199 Search PubMed.
-
Science of Synthesis, Cross Coupling and Heck-Type Reactions, ed. G. Yan, Y. Zhang and J. Wang, Georg Thieme Verlag KG, Stuttgart, 2013 Search PubMed.
- M. Sundermeier, A. Zapf and M. Beller, Eur. J. Inorg. Chem., 2003, 2003, 3513–3526 CrossRef.
- P. Anbarasan, T. Schareina and M. Beller, Chem. Soc. Rev., 2011, 40, 5049–5067 RSC.
-
T. Schareina and M. Beller, in Copper-Mediated Cross-Coupling Reactions, ed. G. Evano and N. Blanchard, Wiley, Weinheim, 2014, ch. 9, pp. 313–334 Search PubMed.
- Q. Wen, J. Jin, L. Zhang, Y. Luo, P. Lu and Y. Wang, Tetrahedron Lett., 2014, 55, 1271–1280 CrossRef CAS.
- Q. Wen, P. Lu and Y. Wang, RSC Adv., 2014, 4, 47806–47826 RSC.
- T. Najam, S. S. A. Shah, K. Mehmood, A. U. Din, S. Rizwan, M. Ashfaq, S. Shaheen and A. Waseem, Inorg. Chim. Acta, 2018, 469, 408–423 CrossRef CAS.
- J.-T. Hou, Synlett, 2010, 3115–3116 CrossRef CAS.
- J. Schorgenhumer and M. Waser, Org. Chem. Front., 2016, 3, 1535–1540 RSC.
- J. Kim, H. J. Kim and S. Chang, Angew. Chem., Int. Ed., 2012, 51, 11948–11959 CrossRef CAS.
- G. P. Ellis and T. M. Romney-Alexander, Chem. Rev., 1987, 87, 779–794 CrossRef CAS.
- G. Yan, Y. Zhang and J. Wang, Adv. Synth. Catal., 2017, 359, 4068–4105 CrossRef CAS.
- J.-T. Yu, F. Teng and J. Cheng, Adv. Synth. Catal., 2016, 359, 26–38 CrossRef.
- Y. Ping, Q. Ding and Y. Peng, ACS Catal., 2016, 6, 5989–6005 CrossRef CAS.
- V. Merz and W. Weith, Ber. Dtsch. Chem. Ges., 1877, 10, 746–765 CrossRef.
- T. Schareina, A. Zapf and M. Beller, Chem. Commun., 2004, 1388–1389 RSC.
- P. Y. Yeung, C. P. Tsang and F. Y. Kwong, Tetrahedron Lett., 2011, 52, 7038–7041 CrossRef CAS.
- L. Liu, J. Li, J. Xu and J.-t. Sun, Tetrahedron Lett., 2012, 53, 6954–6956 CrossRef CAS.
- O. Grossman and D. Gelman, Org. Lett., 2006, 8, 1189–1191 CrossRef CAS.
- B. Mariampillai, J. Alliot, M. Li and M. Lautens, J. Am. Chem. Soc., 2007, 129, 15372–15379 CrossRef CAS.
- P. Y. Yeung, C. M. So, C. P. Lau and F. Y. Kwong, Org. Lett., 2011, 13, 648–651 CrossRef CAS.
- D. Zhang, H. Sun, L. Zhang, Y. Zhou, C. Li, H. Jiang, K. Chen and H. Liu, Chem. Commun., 2012, 48, 2909–2911 RSC.
- T. Zou, X. Feng, H. Liu, X. Yu, Y. Yamamoto and M. Bao, RSC Adv., 2013, 3, 20379–20384 RSC.
- T. V. Magdesieva, O. M. Nikitin, E. V. Zolotukhina and M. A. Vorotyntsev, Electrochim. Acta, 2014, 122, 289–295 CrossRef CAS.
- J. Richardson and S. P. Mutton, J. Org. Chem., 2018, 83, 4922–4931 CrossRef CAS.
- G. Guido, F. Marco, O. Werner, L. Frederic, M. Jean and C. Evelina, ChemSusChem, 2014, 7, 919–924 CrossRef.
- T. Schareina, A. Zapf and M. Beller, J. Organomet. Chem., 2004, 689, 4576–4583 CrossRef CAS.
- Y.-n. Cheng, Z. Duan, T. Li and Y. Wu, Synlett, 2007, 0543–0546 CAS.
- S. A. Weissman, D. Zewge and C. Chen, J. Org. Chem., 2005, 70, 1508–1510 CrossRef CAS.
- T. Schareina, A. Zapf, W. Mägerlein, N. Müller and M. Beller, Tetrahedron Lett., 2007, 48, 1087–1090 CrossRef CAS.
- C. Yi-nan, D. Zheng, L. Ting and W. Yangjie, Lett. Org. Chem., 2007, 4, 352–356 CrossRef.
- Y.-Z. Zhu and C. Cai, Aust. J. Chem., 2008, 61, 581–584 CrossRef CAS.
- Y.-Z. Zhu and C. Cai, Synth. Commun., 2008, 38, 2753–2760 CrossRef CAS.
- S. Velmathi and N. E. Leadbeater, Tetrahedron Lett., 2008, 49, 4693–4694 CrossRef CAS.
- T. Schareina, R. Jackstell, T. Schulz, A. Zapf, A. Cotté, M. Gotta and M. Beller, Adv. Synth. Catal., 2009, 351, 643–648 CrossRef CAS.
- Y. Ren, Z. Liu, S. He, S. Zhao, J. Wang, R. Niu and W. Yin, Org. Process Res. Dev., 2009, 13, 764–768 CrossRef CAS.
- M. Becker, A. Schulz and K. Voss, Synth. Commun., 2011, 41, 1042–1051 CrossRef CAS.
- J. Zhang, X. Chen, T. Hu, Y. Zhang, K. Xu, Y. Yu and J. Huang, Catal. Lett., 2010, 139, 56–60 CrossRef CAS.
- A. R. Hajipour, K. Karami and A. Pirisedigh, Appl. Organomet. Chem., 2010, 24, 454–457 CrossRef CAS.
- J. Schulz, I. Císařová and P. Štěpnička, Organometallics, 2012, 31, 729–738 CrossRef CAS.
- A. R. Hajipour, F. Abrisham and G. Tavakoli, Transition Met. Chem., 2011, 36, 725 CrossRef CAS.
- A. R. Hajipour, K. Karami, G. Tavakoli and A. Pirisedigh, J. Organomet. Chem., 2011, 696, 819–824 CrossRef CAS.
- A. R. Hajipour, F. Rafiee and A. E. Ruoho, Tetrahedron Lett., 2012, 53, 526–529 CrossRef CAS.
- R. Gerber, M. Oberholzer and C. M. Frech, Chem. – Eur. J., 2012, 18, 2978–2986 CrossRef CAS.
- E. A. Savicheva and V. P. Boyarskiy, Russ. Chem. Bull., 2012, 61, 980–983 CrossRef CAS.
- M. Guo, J. Ge, Z. Zhu and X. Wu, Lett. Org. Chem., 2013, 10, 213–215 CrossRef CAS.
- E. Kianmehr, H. Hashemi and A. Darvish, Tetrahedron, 2013, 69, 5193–5196 CrossRef CAS.
- T. D. Senecal, W. Shu and S. L. Buchwald, Angew. Chem., Int. Ed., 2013, 52, 10035–10039 CrossRef CAS.
- A. R. Hajipour and F. Rafiee, J. Iran. Chem. Soc., 2014, 11, 1391–1395 CrossRef CAS.
- M. Utsugi, H. Ozawa, E. Toyofuku and M. Hatsuda, Org. Process Res. Dev., 2014, 18, 693–698 CrossRef CAS.
- Y. Tu, Y. Zhang, S. Xu, Z. Zhang and X. Xie, Synlett, 2014, 25, 2938–2942 CrossRef CAS.
- Z. Xu, Y. Xiao, H. Ding, C. Cao, H. Li, G. Pang and Y. Shi, Synthesis, 2015, 47, 1560–1566 CrossRef CAS.
- M. Vafaeezadeh, M. M. Hashemi and M. Karbalaie-Reza, Inorg. Chem. Commun., 2016, 72, 86–90 CrossRef CAS.
- B. Majhi and B. C. Ranu, Org. Lett., 2016, 18, 4162–4165 CrossRef CAS.
- J. Schulz, F. Horky and P. Stepnicka, Catalysts, 2016, 6, 182 CrossRef.
- T. Schareina, A. Zapf and M. Beller, Tetrahedron Lett., 2005, 46, 2585–2588 CrossRef CAS.
- Y.-Z. Zhu and C. Cai, J. Chem. Res., 2007, 2007, 484 CrossRef.
- T. Schareina, A. Zapf, W. Mägerlein, N. Müller and M. Beller, Chem. – Eur. J., 2007, 13, 6249–6254 CrossRef CAS.
- T. Schareina, A. Zapf, A. Cotté, N. Müller and M. Beller, Synthesis, 2008, 3351–3355 CAS.
- Y. Ren, Z. Liu, S. Zhao, X. Tian, J. Wang, W. Yin and S. He, Catal. Commun., 2009, 10, 768–771 CrossRef CAS.
- Y. Ren, W. Wang, S. Zhao, X. Tian, J. Wang, W. Yin and L. Cheng, Tetrahedron Lett., 2009, 50, 4595–4597 CrossRef CAS.
- C. DeBlase and N. E. Leadbeater, Tetrahedron, 2010, 66, 1098–1101 CrossRef CAS.
- M. M. Coughlin, C. K. Kelly, S. Lin and A. H. R. MacArthur, Organometallics, 2013, 32, 3537–3543 CrossRef CAS.
- S. Bahari and A. Rezaei, Lett. Org. Chem., 2014, 11, 519–523 CrossRef CAS.
- S. M. Sajadi and M. Maham, Lett. Org. Chem., 2014, 11, 136–140 CrossRef CAS.
- W. Zhou, W. Chen and L. Wang, Org. Biomol. Chem., 2012, 10, 4172–4178 RSC.
- X. Tian, Y. Sun, C. Dong, K. Zhang, T. Liang, Y. Zhang and C. Hou, Chem. Lett., 2012, 41, 719–721 CrossRef CAS.
- Y. Z. Zhu and C. Cai, Eur. J. Org. Chem., 2007, 2401–2404 CrossRef CAS.
- V. Polshettiwar, P. Hesemann and J. J. E. Moreau, Tetrahedron, 2007, 63, 6784–6790 CrossRef CAS.
- I. P. Beletskaya, A. V. Selivanova, V. S. Tyurin, V. V. Matveev and A. R. Khokhlov, Russ. J. Org. Chem., 2010, 46, 157–161 CrossRef CAS.
- M. Islam, P. Mondal, K. Tuhina, A. S. Roy, S. Mondal and D. Hossain, J. Organomet. Chem., 2010, 695, 2284–2295 CrossRef CAS.
- S. M. Islam, P. Mondal, K. Tuhina and S. Roy Anupam, J. Chem. Technol. Biotechnol., 2010, 85, 999–1010 CrossRef CAS.
- H. Yu, R. N. Richey, W. D. Miller, J. Xu and S. A. May, J. Org. Chem., 2011, 76, 665–668 CrossRef CAS.
- D. Saha, L. Adak, M. Mukherjee and B. C. Ranu, Org. Biomol. Chem., 2012, 10, 952–957 RSC.
- I. A. Azath, P. Suresh and K. Pitchumani, New J. Chem., 2012, 36, 2334–2339 RSC.
- A. Modak, J. Mondal and A. Bhaumik, Green Chem., 2012, 14, 2840–2855 RSC.
- W. Yin, R. Liu, G. He, W. Lv and H. Zhu, RSC Adv., 2014, 4, 37773–37778 RSC.
- T. Chatterjee, R. Dey and B. C. Ranu, J. Org. Chem., 2014, 79, 5875–5879 CrossRef CAS.
- D. Ganapathy, S. S. Kotha and G. Sekar, Tetrahedron Lett., 2015, 56, 175–178 CrossRef CAS.
- B. Suresh Kumar, A. J. Amali and K. Pitchumani, ACS Appl. Mater Interfaces, 2015, 7, 22907–22917 CrossRef CAS.
- M. Gholinejad and A. Aminianfar, J. Mol. Catal. A: Chem., 2015, 397, 106–113 CrossRef CAS.
- M. Nasrollahzadeh, S. M. Sajadi, A. Rostami-Vartooni and M. Khalaj, J. Colloid Interface Sci., 2015, 453, 237–243 CrossRef CAS.
- M. Nasrollahzadeh, Tetrahedron Lett., 2016, 57, 337–339 CrossRef CAS.
- M. Nasrollahzadeh and S. M. Sajadi, J. Colloid Interface Sci., 2016, 469, 191–195 CrossRef CAS.
- Z.-C. Wu, Q. Yang, X. Ge, Y.-M. Ren, R.-C. Yang and T.-X. Tao, Catal. Lett., 2017, 147, 1333–1338 CrossRef.
- Y. Yeung Pui, M. So Chau, P. Lau Chak and Y. Kwong Fuk, Angew. Chem., Int. Ed., 2010, 49, 8918–8922 CrossRef.
- C. Zhao, W.-Y. Fang, K. P. Rakesh and H.-L. Qin, Org. Chem. Front., 2018, 5, 1835–1839 RSC.
- V. K. Das, S. N. Harsh and N. Karak, Tetrahedron Lett., 2016, 57, 549–553 CrossRef CAS.
- M. Nasrollahzadeh, M. Atarod and S. M. Sajadi, J. Colloid Interface Sci., 2017, 486, 153–162 CrossRef CAS.
- X. Jia, D. Yang, W. Wang, F. Luo and J. Cheng, J. Org. Chem., 2009, 74, 9470–9474 CrossRef CAS.
- G. Yan, C. Kuang, Y. Zhang and J. Wang, Org. Lett., 2010, 12, 1052–1055 CrossRef CAS.
- Y. Ren, M. Yan, S. Zhao, J. Wang, J. Ma, X. Tian and W. Yin, Adv. Synth. Catal., 2012, 354, 2301–2308 CrossRef CAS.
- For most cited publication in this review we count the number of examples and notify the yield range. However, we excluded examples with yields below 10% or optimization reactions.
- Y. Ren, M. Yan, S. Zhao, Y. Sun, J. Wang, W. Yin and Z. Liu, Tetrahedron Lett., 2011, 52, 5107–5109 CrossRef CAS.
- Y. Ren, C. Dong, S. Zhao, Y. Sun, J. Wang, J. Ma and C. Hou, Tetrahedron Lett., 2012, 53, 2825–2827 CrossRef CAS.
- L. Fu and G. W. Gribble, Org. Lett., 2013, 15, 1622–1625 CrossRef CAS.
- X. Hu, R. Li and Z. Li, J. Chem. Res., 2014, 38, 432–436 CrossRef CAS.
- A. M. Nauth, N. Otto and T. Opatz, Adv. Synth. Catal., 2015, 357, 3424–3428 CrossRef CAS.
- A. M. Nauth, J. C. Orejarena Pacheco, S. Pusch and T. Opatz, Eur. J. Org. Chem., 2017, 6966–6974 CrossRef CAS.
- A. Pinto, Y. Jia, L. Neuville and J. Zhu, Chem. – Eur. J., 2007, 13, 961–967 CrossRef CAS.
- Z. Zhao and Z. Li, Eur. J. Org. Chem., 2010, 5460–5463 CrossRef CAS.
- X. Hu, Z. Zhao and Z. Li, Phosphorus, Sulfur Silicon Relat. Elem., 2012, 187, 1003–1008 CrossRef CAS.
- Z. Li, R. Li, H. Zheng, F. Wen, H. Li, J. Yin and J. Yang, J. Brazil. Chem. Soc., 2013, 24, 1739–1743 Search PubMed.
- X. Hu, H. Li, J. Yang and Z. Li, Synlett, 2014, 1786–1790 Search PubMed.
- Z. Li, J. Yin, T. Li, G. Wen, X. Shen and J. Yang, Tetrahedron, 2014, 70, 5619–5625 CrossRef CAS.
- A. M. Nauth, T. Konrad, Z. Papadopulu, N. Vierengel, B. Lipp and T. Opatz, Green Chem., 2018, 20, 4217–4223 RSC.
- C. Bolm, R. Mocci, C. Schumacher, M. Turberg, F. Puccetti and G. Hernández José, Angew. Chem., Int. Ed., 2018, 57, 2423–2426 CrossRef CAS.
- F.-H. Luo, C.-I. Chu and C.-H. Cheng, Organometallics, 1998, 17, 1025–1030 CrossRef CAS.
- Q. Wen, J. Jin, B. Hu, P. Lu and Y. Wang, RSC Adv., 2012, 2, 6167–6169 RSC.
- Q. Wen, J. Jin, Y. Mei, P. Lu and Y. Wang, Eur. J. Org. Chem., 2013, 4032–4036 CrossRef CAS.
- J. Jin, Q. Wen, P. Lu and Y. Wang, Chem. Commun., 2012, 48, 9933–9935 RSC.
- C. Zhang, C. Liu, Y. Shao, X. Bao and X. Wan, Chem. – Eur. J., 2013, 19, 17917–17925 CrossRef CAS.
- R.-J. Song, J.-C. Wu, Y. Liu, G.-B. Deng, C.-Y. Wu, W.-T. Wei and J.-H. Li, Synlett, 2012, 23, 2491–2496 CrossRef CAS.
- W. Xu, Q. Xu and J. Li, Org. Chem. Front., 2015, 2, 231–235 RSC.
- C. Pan, H. Jin, P. Xu, X. Liu, Y. Cheng and C. Zhu, J. Org. Chem., 2013, 78, 9494–9498 CrossRef CAS PubMed.
- M. Zhao, W. Zhang and Z. Shen, J. Org. Chem., 2015, 80, 8868–8873 CrossRef CAS PubMed.
- Y. Zhu, M. Zhao, W. Lu, L. Li and Z. Shen, Org. Lett., 2015, 17, 2602–2605 CrossRef CAS PubMed.
- X. Kou, M. Zhao, X. Qiao, Y. Zhu, X. Tong and Z. Shen, Chem. – Eur. J., 2013, 19, 16880–16886 CrossRef CAS PubMed.
- S. Zheng, C. Yu and Z. Shen, Org. Lett., 2012, 14, 3644–3647 CrossRef CAS PubMed.
- S. Goswami, A. Manna, A. K. Maity, S. Paul, A. K. Das, M. K. Das, P. Saha, C. K. Quah and H.-K. Fun, Dalton Trans., 2013, 42, 12844–12848 RSC.
- K. Buttke, T. Reiher and H. J. Niclas, Synth. Commun., 1992, 22, 2237–2243 CrossRef CAS.
- N. Sato, Tetrahedron Lett., 2002, 43, 6403–6404 CrossRef CAS.
- N. Sato and Q. Yue, Tetrahedron, 2003, 59, 5831–5836 CrossRef CAS.
- R. E. Murray and G. Zweifel, Synthesis, 1980, 150–151 CrossRef CAS.
- J.-S. Qiu, Y.-F. Wang, G.-R. Qi, G. Karmaker Pran, H.-Q. Yin and F.-X. Chen, Chem. – Eur. J., 2016, 23, 1775–1778 CrossRef PubMed.
- J. Qiu, D. Wu, P. G. Karmaker, G. Qi, P. Chen, H. Yin and F.-X. Chen, Org. Lett., 2017, 19, 4018–4021 CrossRef CAS PubMed.
- Y. Tamura, T. Kawasaki, M. Adachi, M. Tanio and Y. Kita, Tetrahedron Lett., 1977, 50, 4417–4420 CrossRef.
- W. A. Davis and M. P. Cava, J. Org. Chem., 1983, 48, 2274–2275 CrossRef.
- Z. Zhang and L. S. Liebeskind, Org. Lett., 2006, 8, 4331–4333 CrossRef CAS PubMed.
- Z. Zhang and L. S. Liebeskind, Synfacts, 2006, 2006, 1270–1270 CrossRef.
- G.-Y. Zhang, J.-T. Yu, M.-L. Hu and J. Cheng, J. Org. Chem., 2013, 78, 2710–2714 CrossRef CAS PubMed.
- B. K. Vaghasiya, S. P. Satasia, R. P. Thummar, R. D. Kamani, J. R. Avalani, N. H. Sapariya and D. K. Raval, J. Sulfur Chem., 2018, 1–9 Search PubMed.
- A. Wagner and A. R. Ofial, J. Org. Chem., 2015, 80, 2848–2854 CrossRef CAS PubMed.
- Y. Huang, Y. Yu, Z. Zhu, C. Zhu, J. Cen, X. Li, W. Wu and H. Jiang, J. Org. Chem., 2017, 82, 7621–7627 CrossRef CAS PubMed.
- S. Kamijo, T. Hoshikawa and M. Inoue, Org. Lett., 2011, 13, 5928–5931 CrossRef CAS PubMed.
- T. Hoshikawa, S. Yoshioka, S. Kamijo and M. Inoue, Synthesis, 2013, 45, 874–887 CrossRef CAS.
- R. Akula, Y. Xiong and H. Ibrahim, RSC Adv., 2013, 3, 10731–10735 RSC.
- V. V. Zhdankin, C. J. Kuehl, A. P. Krasutsky, J. T. Bolz, B. Mismash, J. K. Woodward and A. J. Simonsen, Tetrahedron Lett., 1995, 36, 7975–7978 CrossRef CAS.
- R. Chowdhury, J. Schörgenhumer, J. Novacek and M. Waser, Tetrahedron Lett., 2015, 56, 1911–1914 CrossRef CAS PubMed.
- M. Chen, Z.-T. Huang and Q.-Y. Zheng, Org. Biomol. Chem., 2015, 13, 8812–8816 RSC.
- B. Ma, X. Lin, L. Lin, X. Feng and X. Liu, J. Org. Chem., 2017, 82, 701–708 CrossRef CAS PubMed.
- Y.-F. Wang, J. Qiu, D. Kong, Y. Gao, F. Lu, P. G. Karmaker and F.-X. Chen, Org. Biomol. Chem., 2015, 13, 365–368 RSC.
- R. Frei, T. Courant, D. Wodrich Matthew and J. Waser, Chem. – Eur. J., 2014, 21, 2662–2668 CrossRef PubMed.
- T. Nagata, H. Matsubara, K. Kiyokawa and S. Minakata, Org. Lett., 2017, 19, 4672–4675 CrossRef CAS PubMed.
- P. J. Stang and V. V. Zhdankin, Chem. Rev., 1996, 96, 1123–1178 CrossRef CAS PubMed.
- V. V. Zhdankin and P. J. Stang, Chem. Rev., 2002, 102, 2523–2584 CrossRef CAS PubMed.
- Z. Shu, W. Ji, X. Wang, Y. Zhou, Y. Zhang and J. Wang, Angew. Chem., Int. Ed., 2014, 53, 2186–2189 CrossRef CAS PubMed.
- D. Zhu, D. Chang and L. Shi, Chem. Commun., 2015, 51, 7180–7183 RSC.
- X. Wang and A. Studer, J. Am. Chem. Soc., 2016, 138, 2977–2980 CrossRef CAS PubMed.
- F. Kurzer, J. Chem. Soc., 1949, 1034–1038 RSC.
- F. Kurzer, J. Chem. Soc., 1949, 3029–3033 RSC.
- S. Mo, Synlett, 2014, 1337–1338 CAS.
- P. Anbarasan, H. Neumann and M. Beller, Chem. – Eur. J., 2011, 17, 4217–4222 CrossRef CAS PubMed.
- P. Anbarasan, H. Neumann and M. Beller, Angew. Chem., Int. Ed., 2011, 50, 519–522 CrossRef CAS PubMed.
- Y. Yang, Y. Zhang and J. Wang, Org. Lett., 2011, 13, 5608–5611 CrossRef CAS PubMed.
- M. Chaitanya, D. Yadagiri and P. Anbarasan, Org. Lett., 2013, 15, 4960–4963 CrossRef CAS PubMed.
- D.-G. Yu, T. Gensch, F. de Azambuja, S. Vásquez-Céspedes and F. Glorius, J. Am. Chem. Soc., 2014, 136, 17722–17725 CrossRef CAS PubMed.
- Y. Yang and S. L. Buchwald, Angew. Chem., Int. Ed., 2014, 53, 8677–8681 CrossRef CAS PubMed.
- Y. Yang and P. Liu, ACS Catal., 2015, 5, 2944–2951 CrossRef CAS.
- Y. Cai, X. Qian, A. Rérat, A. Auffrant and C. Gosmini, Adv. Synth. Catal., 2015, 357, 3419–3423 CrossRef CAS.
- J. Li, W. Xu, J. Ding and K.-H. Lee, Tetrahedron Lett., 2016, 57, 1205–1209 CrossRef CAS.
- K. Kiyokawa, T. Nagata and S. Minakata, Angew. Chem., Int. Ed., 2016, 55, 10458–10462 CrossRef CAS PubMed.
- F. Song, R. Salter and L. Chen, J. Org. Chem., 2017, 82, 3530–3537 CrossRef CAS PubMed.
- R. Crossley and R. G. Shepherd, J. Chem. Soc., Perkin Trans. 1, 1985, 2479–2481 RSC.
- T. V. Hughes, S. D. Hammond and M. P. Cava, J. Org. Chem., 1998, 63, 401–402 CrossRef CAS.
- T. V. Hughes and M. P. Cava, J. Org. Chem., 1999, 64, 313–315 CrossRef CAS PubMed.
- A. R. Katritzky, R. Akue-Gedu and A. V. Vakulenko, ARKIVOC, 2007, 3, 5–12 Search PubMed.
- Y.-q. Wu, D. C. Limburg, D. E. Wilkinson and G. S. Hamilton, Org. Lett., 2000, 2, 795–797 CrossRef CAS PubMed.
- P. Anbarasan, H. Neumann and M. Beller, Chem. – Eur. J., 2010, 16, 4725–4728 CrossRef CAS PubMed.
- J.-J. Kim, D.-H. Kweon, S.-D. Cho, H.-K. Kim, E.-Y. Jung, S.-G. Lee, J. R. Falck and Y.-J. Yoon, Tetrahedron, 2005, 61, 5889–5894 CrossRef CAS.
- S. Xu, X. Huang, X. Hong and B. Xu, Org. Lett., 2012, 14, 4614–4617 CrossRef CAS PubMed.
- X. Jiang, J.-M. Wang, Y. Zhang, Z. Chen, Y.-M. Zhu and S.-J. Ji, Tetrahedron, 2015, 71, 4883–4887 CrossRef CAS.
- A. Coppola, P. Sánchez-Alonso, D. Sucunza, C. Burgos, R. Alajarín, J. Alvarez-Builla, M. E. G. Mosquera and J. J. Vaquero, Org. Lett., 2013, 15, 3388–3391 CrossRef CAS PubMed.
- K. H. V. Reddy, G. Satish, V. P. Reddy, B. S. P. A. Kumar and Y. V. D. Nageswar, RSC Adv., 2012, 2, 11084–11088 RSC.
- V. Rao Kasanneni Tirumala, B. Haribabu, S. S. Prasad Potharaju and N. Lingaiah, ChemCatChem, 2012, 4, 1173–1178 CrossRef.
- A. Brahma, B. Musio, U. Ismayilova, N. Nikbin, S. B. Kamptmann, P. Siegert, G. E. Jeromin, S. V. Ley and M. Pohl, Synlett, 2016, 27, 262–266 CAS.
- J. Vicario, J. M. Ezpeleta and F. Palacios, Adv. Synth. Catal., 2012, 354, 2641–2647 CrossRef CAS.
- H. Xu, P.-T. Liu, Y.-H. Li and F.-S. Han, Org. Lett., 2013, 15, 3354–3357 CrossRef CAS PubMed.
- F. Teng, J.-T. Yu, H. Yang, Y. Jiang and J. Cheng, Chem. Commun., 2014, 50, 12139–12141 RSC.
- F. Teng, J.-T. Yu, Z. Zhou, H. Chu and J. Cheng, J. Org. Chem., 2015, 80, 2822–2826 CrossRef CAS PubMed.
- G. Rong, J. Mao, Y. Zheng, R. Yao and X. Xu, Chem. Commun., 2015, 51, 13822–13825 RSC.
- P.-Y. Liu, C. Zhang, S.-C. Zhao, F. Yu, F. Li and Y.-P. He, J. Org. Chem., 2017, 82, 12786–12790 CrossRef CAS PubMed.
- T. Tajima and A. Nakajima, J. Am. Chem. Soc., 2008, 130, 10496–10497 CrossRef CAS PubMed.
- A. Karimi Zarchi Mohammad and N. Ebrahimi, J. Appl. Polym. Sci., 2012, 125, 2163–2169 CrossRef.
- R. Takise, K. Itami and J. Yamaguchi, Org. Lett., 2016, 18, 4428–4431 CrossRef CAS PubMed.
- A. B. Pawar and S. Chang, Org. Lett., 2015, 17, 660–663 CrossRef CAS PubMed.
- G. Talavera, J. Peña and M. Alcarazo, J. Am. Chem. Soc., 2015, 137, 8704–8707 CrossRef CAS PubMed.
- J. Bergman and B. Pelcman, Tetrahedron Lett., 1986, 27, 1939–1942 CrossRef CAS.
- K. Hosoi, K. Nozaki and T. Hiyama, Org. Lett., 2002, 4, 2849–2851 CrossRef CAS PubMed.
- N. Sawant Dinesh, S. Wagh Yogesh, J. Tambade Pawan, D. Bhatte Kushal and M. Bhanage Bhalchandra, Adv. Synth. Catal., 2011, 353, 781–787 CrossRef.
- A. B. Khemnar, D. N. Sawant and B. M. Bhanage, Tetrahedron Lett., 2013, 54, 2682–2684 CrossRef CAS.
- S. Ding and N. Jiao, J. Am. Chem. Soc., 2011, 133, 12374–12377 CrossRef CAS PubMed.
- J. Kim and S. Chang, J. Am. Chem. Soc., 2010, 132, 10272–10274 CrossRef CAS PubMed.
- G. Zhang, X. Ren, J. Chen, M. Hu and J. Cheng, Org. Lett., 2011, 13, 5004–5007 CrossRef CAS PubMed.
- A. B. Pawar and S. Chang, Chem. Commun., 2014, 50, 448–450 RSC.
- J. Kim, J. Choi, K. Shin and S. Chang, J. Am. Chem. Soc., 2012, 134, 2528–2531 CrossRef CAS PubMed.
- Z. Wang and S. Chang, Org. Lett., 2013, 15, 1990–1993 CrossRef CAS PubMed.
- X. Chen, X.-S. Hao, C. E. Goodhue and J.-Q. Yu, J. Am. Chem. Soc., 2006, 128, 6790–6791 CrossRef CAS PubMed.
- X. Ren, J. Chen, F. Chen and J. Cheng, Chem. Commun., 2011, 47, 6725–6727 RSC.
- K. Zheng, B. Liu, S. Chen and F. Chen, Tetrahedron Lett., 2013, 54, 5250–5252 CrossRef CAS.
- For industrial synthesis from iron salts and HCN.
- For synthesis from blood, horn, bone or flesh.
|
This journal is © The Royal Society of Chemistry 2019 |