DOI:
10.1039/C7NJ03146G
(Paper)
New J. Chem., 2018,
42, 228-236
Hydrothermal conversion of glucose to levulinic acid using multifunctional ionic liquids: effects of metal ion co-catalysts on the product yield
Received
25th August 2017
, Accepted 16th November 2017
First published on 16th November 2017
Abstract
Efficient conversion of glucose to levulinic acid (LA) was achieved with –SO3H functionalized ionic liquids in combination with a catalytic amount of metal salts under environmentally benign mild reaction conditions. Ionic liquids having different Bronsted acidic functional groups were prepared in the laboratory. The acidity of these ionic liquids was determined on the basis of the Hammett acidity function using UV-Visible spectroscopy. These catalysts were then screened in the conversion of glucose to LA using a batch autoclave under autogenous pressure at a temperature range of 140 to 175 °C. The structure–activity relationship of these multifunctional ionic liquids was correlated based on the Hammett function (Ho) and the catalytic activity results obtained in terms of glucose conversion and LA yield. The catalytic activity of the functionalized ionic liquids decreased in the order: IL-SO3H > IL-COOH > IL-OH, which is in good agreement with their Bronsted acidity trends. Furthermore, a metal salt based Lewis acidic co-catalyst, with metal ions (Fe, Co, Ni, Cu and Zn), was used in combination with the laboratory prepared Bronsted acidic functionalized ionic liquids in the efficient conversion of glucose to LA. Among all the investigated catalyst and co-catalyst systems, the –SO3H functionalized ionic liquid with nickel sulfate (NiSO4·6H2O) showed the highest LA yield (56.33%) and selectivity (56.37%). The synergistic effect of the metal ions helps in improving the LA yield by 10% as observed in our investigations. The co-catalytic effect of the transition metal chlorides and metal nitrates was not obvious as compared to transition metal sulfates. The catalyst could be recovered easily and reused three times without significant loss of activity.
1. Introduction
The production of industrially important fine and bulk chemicals from readily available lignocellulosic biomass has become a new trend in catalysis.1 Lignocellulosic biomass is composed of lignin, cellulose and hemicellulose, and is found to be a natural renewable carbon resource.2 Cellulose is readily available and is a sustainable biomass-derived raw material, made up of D-glucose units linked together by β-1,4 glycosidic bonds.3 Cellulose can be hydrolyzed to glucose and further isomerization of glucose leads to the production of industrially important chemicals such as LA, 5-hydroxymethylfurfural (HMF) and lactic acid as shown in Scheme 1.4 LA is the rehydrated product that can be produced from the rehydration of HMF, an intermediate that can be obtained by the catalytic conversion of lignocellulosic biomass.5 LA with two active, carbonyl and carboxyl functional groups can be converted to γ-valerolactone and other liquid transportation fuels through hydrogenation reactions.6
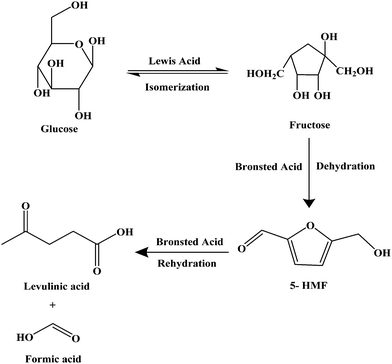 |
| Scheme 1 Mechanistic representation of the formation of LA and formic acid from glucose. | |
To date, several studies have been reported on the conversion of lignocellulosic biomass to LA using mineral acids,7 metal sulfates8 and heteropoly acids as catalysts. Despite the good yield, the above processes possess the disadvantage of product separation, recycling of the catalyst, reactor corrosion, selectivity to the desired products and economically expensive heteropoly acids. Recently, ionic liquids have been reported as solvents as well as catalysts for the catalytic transformation of cellulose to LA.9 Ionic liquids are also known as designer solvents due to their unique properties of low vapor and task specificity that can be achieved by the modification of the cations and anions.10–13 Tao et al. have recently reported functionalized carboxylic acid based ionic liquids for alcoholysis reactions.14 Yu et al. reported high glucose conversion to HMF using Bronsted acidic ionic liquids as a catalyst and metal chlorides as a co-catalyst.15 Amin et al. reported the conversion of glucose to LA using a dual Bronsted and Lewis acidic ionic liquid with tetrachloroferrate as an anion.16 However, the available literature on glucose conversion to LA still lacks a detailed study of the effect of transition metal ions (chlorides, sulfates, and nitrates) as co-catalysts along with ionic liquids as catalysts.
In this study, ionic liquids with varied functional groups (IL-SO3H, IL-COOH, and IL-OH) were employed in the catalytic conversion of glucose to LA. Moreover, the effect of metal salts (MSO4, MCl, and MNO3) in combination with ionic liquids has been investigated. The effect of various reaction conditions such as time and temperature was also investigated on the glucose conversion to LA. The recyclability of ionic liquids was also investigated for the cost-optimization of the process.
2. Materials and methods
Glucose, fructose (99%), LA (99%), 5-hydroxymethylfurfural (98%) (HMF), and formic acid (99%) (FA) were purchased from Fisher Scientific, India. 1,6-Anhydro-β-D-glucose (99%) and 5-chlorovaleric acid (96%) were purchased from TCI chemicals, USA. 4-Chloro-1-butanol (85%), 1-methylimidazole (99%), and 1,4-butane sultone (99%) were purchased from Alfa Aesar, China. Diethyl ether, FeCl3 (97%), CoCl2 (97%), NiCl2 (98%), CuCl2 (98%) and ZnCl2 (97%) were purchased from Merck, India. FeSO4·7H2O (98%), CoSO4·7H2O (97%), NiSO4·6H2O (97%), CuSO4·5H2O (99%) and ZnSO4·7H2O (95%) were purchased from Sigma Aldrich and BDH chemicals. Metal nitrates Fe(NO3)2·7H2O (99%), Co(NO3)2·7H2O (97%), Ni(NO3)2·6H2O (98%), Cu(NO3)2·5H2O (99%) and Zn(NO3)2·7H2O (96%) were purchased from CDH chemicals. 1-Butyl-2,3-dimethylimidazolium hexafluorophosphate (98%) and 1-butyl-3-methylimidazolium tetrafluoroborate (98%) were purchased from Merck, Germany.
2.1 Synthesis of functionalized ionic liquids
A series of Bronsted acid functionalized ionic liquids were synthesized as shown in Scheme 2 for the catalytic conversion of glucose to LA, and their catalytic activity was compared with the literature reported ionic liquids.
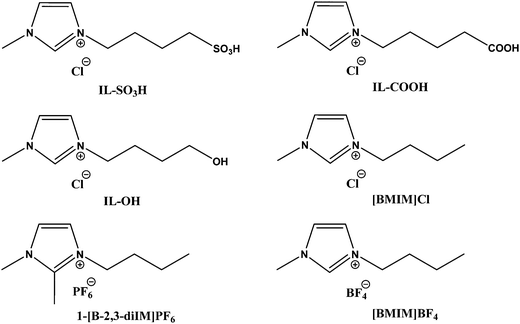 |
| Scheme 2 Chemical structures of the functionalized ionic liquids used in this study. | |
2.1.1 1-(4-Sulfonic acid)butyl-3-methylimidazolium chloride.
The sulfonic acid functionalized ionic liquid was synthesized according to the reported literature.17–22 Equimolar amounts of 1-methylimidazole and 1,4-butane sultone at a ratio of 1
:
1.2 were mixed in a flask with toluene as a solvent and stirred at 80 °C for 6 h to obtain the solid zwitterion. The zwitterionic solid was washed three times (8 mL × 3) with diethyl ether and dried under vacuum at room temperature for 8 h. The resultant zwitterion was neutralized by adding an equimolar amount of hydrochloric acid with stirring at room temperature for 8–9 h. The yield of the synthesized ionic liquid, IL-SO3H, was obtained to be 89%. 1H NMR: (400 MHz, D2O): δ (ppm) 1.67–1.74 (m, 2H), 1.95–2.02 (m, 2H), 2.88–2.92 (t, 2H), 3.86 (s, 3H), 4.19–4.23 (t, 2H), 7.41–7.47 (d, 2H), 8.71 (s, 1H). 13C NMR: (100 MHz; D2O): δ (ppm) 20.84, 28.02, 35.58, 48.80, 49.98, 122.06, 123.55, and 135.88.
2.1.2 1-(5-Carboxypentyl)-3-methylimidazolium chloride.
The carboxylic acid functionalized ionic liquid was synthesized by stirring a mixture of equimolar amounts of 1-methylimidazole and 5-chlorovaleric acid at 70 °C for 12 h. The resultant pale yellow mixture was washed with ether (50 mL × 3). The yellow sticky product was dried under vacuum at 40 °C overnight. The yield of the synthesized ionic liquid, IL-COOH, was found to be 92%. 1H NMR: (400 MHz; D2O): δ (ppm) 1.52–1.58 (m, 2H), 1.85–1.89 (m, 2H), 2.35–2.39 (t, 2H), 3.87 (s, 3H), 4.18–4.22 (t, 2H), 7.43–7.49 (d, 2H), 8.68 (s, 1H). 13C NMR: (100 MHz, D2O): δ (ppm) 20.98, 28.65, 35.60, 35.81, 49.09, 122.17, 123.60, 135.88, and 178.01.
2.1.3 Synthesis of 1-(4-hydroxybutyl)-3-methylimidazolium chloride.
An ionic liquid containing OH as a functional group was synthesized according to the procedure reported in ref. 23. 1-Methylimidazole and 4-chloro-1-butanol were mixed in 1
:
1.2 molar ratios and stirred at 80 °C for 5 h. The desired ionic liquid was obtained in a viscous liquid form, and the unreacted materials were washed off by diethyl ether (8 mL × 3). The reminiscent diethyl ether was removed under reduced pressure at room temperature. The yield of the obtained colorless viscous ionic liquid, IL-OH, was found to be 89%. 1H NMR (400 MHz, D2O) δ (ppm) 1.59–1.62 (2H, m, CH2), 1.95–1.98 (m, 2H, CH2), 3.94 (s, 3H, N–CH3), 3.63–3.66 (t, 2H, N–CH2), 4.28 (dt, 2H, CH2–OH), 7.50, 7.56 [two singlets, 2H, C(4,5)–H], 8.74 [s, 1H, C(2)–H]. 13C NMR: (100 MHz, D2O): δ (ppm) 26.24, 28.00, 35.59, 49.29, 60.88, 122.21, 123.04 and 135.93.
2.1.4 Synthesis of [BMIM]Cl.
The ionic liquid [BMIM]Cl was synthesized following the reported literature.24 1-Methyl imidazole and 1-chlorobutane were stirred at 60 °C overnight. The unreacted material was washed by diethyl ether and acetonitrile. The diethyl ether was removed under reduced pressure followed by heating at room temperature. The resultant ionic liquid was characterized using 1H NMR and 13C NMR prior to use. 1H NMR (400 MHz, D2O) δ (ppm) 0.797 (3H, t, CH3), 1.18 (m, 2H, CH2), 1.707 (m, 2H, CH2), 3.75 (s, 3H, N–CH3), 4.03–4.08 (t, 2H, N–CH2), 4.60 (s, 2H, CH2-OH), 7.29–7.34 [two singlets, 2H, C(4,5)–H], 8.584 [s, 1H, C(2)–H]. 13C NMR: (100 MHz, D2O): δ (ppm) 12.81, 18.84, 31.32, 35.75, 49.28, 122.22, 123.49 and 135.57.
2.2 Catalytic activity tests in glucose conversion to LA
Catalytic conversion of glucose to LA was carried out in a 25 mL autoclave by heating an aqueous reaction mixture at a temperature of 140–175 °C. In a typical reaction, 0.56 mmol glucose, 1.8 wt% metal salts and 1 g of aqueous solution of the ionic liquid were charged into the autoclave. After the reaction, heating was terminated and the reaction was quenched by cooling the autoclave in an ice bath. During the separation of the product, the viscosity of the reaction mixture was reduced by adding some amount of distilled water. Recycling of the ionic liquids was carried out as described in the reported literature.4,25,26
2.3 Product analysis from HPLC
Quantitative analysis of the liquid sample was performed using high pressure liquid chromatography (HPLC). The liquid samples were collected from aliquots of the reaction mixture and the samples were filtered by using cellulose acetate membrane filters (0.2 μm) and analyzed using an Agilent HPLC equipped with an HPX-87H column and RID with 5 mM sulfuric acid as the mobile phase.
The conversion of glucose was estimated according to eqn (1):
| Conversion of glucose (%) = {(Initial no. of moles of glucose − remaining moles of the glucose after the reaction)/Initial moles of glucose added} × 100% | (1) |
The yield of LA was estimated according to eqn (2):
| Yield of LA (%) = (Moles of LA obtained/Initial moles of glucose) × 100% | (2) |
2.4 Determination of acidity of ionic liquids using UV-vis spectroscopy
The Bronsted acidic strength of the synthesized ionic liquids was determined using the Hammett function. A solution of 4-nitroaniline was used as an indicator to trap the dissociated acidic protons of the ionic liquids in water using the literature reported procedure.24,27–32 The acidity function (Ho) value was estimated according to eqn (3): | 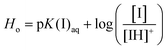 | (3) |
where pK(I)aq is the pKa value of the indicator, and [I] and [IH+] are the molar concentrations of the unprotonated and protonated forms of the indicator in the solvent, respectively. The absorbance of the probe (4-nitroaniline solution) and the probe in the presence of different concentrations of the ionic liquids was recorded at 380 nm using a HALO DB-20 UV-visible double-beam spectrophotometer.
3. Results and discussion
3.1 Screening of ionic liquids for glucose conversion to LA
The effect of different functional groups on the conversion of glucose and the selectivity of LA was studied at 155 °C for 5 h as shown in Fig. 2. From Fig. 2, it is evident that the Bronsted acid functionalized ionic liquids (IL-SO3H, IL-COOH, and IL-OH) showed a higher catalytic conversion of glucose to LA as compared to the non-functionalized ionic liquids. Among the Bronsted acidic ionic liquids, IL-SO3H showed a maximum conversion which can be attributed to its highest acidity as shown in Table 1 and Fig. 1, and the fact that it possessed a maximum Bronsted acidity with a minimum value of the Hammett function (Ho).
Table 1 The Hammett acidity function (Ho) values of all Bronsted acidic ionic liquids in water
ILs |
Amax |
[I] (%) |
[IH+] (%) |
[Ho] |
None |
1 |
100 |
0 |
— |
IL-SO3H |
0.83 |
93.29 |
6.71 |
1.97 |
IL-COOH |
0.84 |
94.93 |
5.07 |
2.12 |
IL-OH |
0.86 |
97.13 |
2.87 |
2.39 |
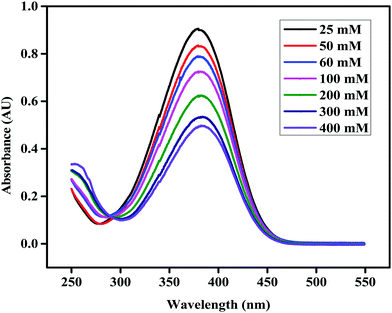 |
| Fig. 1 Absorption spectra of 4-nitroaniline in the presence of various concentrations of IL-SO3H in H2O. | |
Furthermore, the highly acidic proton of IL-SO3H and the chloro anion create a polar environment through hydrogen bonding that is required for glucose conversion to LA.33 Among the non-functionalized ionic liquids, [BMIM]Cl showed a higher conversion of glucose but low selectivity of LA due to the absence of Bronsted acidity. The presence of the non-interacting hydrophobic cation in [BMIM]Cl results in free chloro groups, which are more reactive than BF4− and PF6− anions.
From Fig. 2, the order of activity is observed to be IL-SO3H > IL-COOH > IL-OH > [BMIM]Cl > 1-[B-2,3-diIM]PF6 > [BMIM]BF4 > Blank. This trend can be explained by the Hammett function of the acidic ionic liquids and the reactivity of the anion of the non-functionalized ionic liquids. Thus, it can be concluded that IL-SO3H having higher Bronsted acidity results in almost 100% conversion of glucose with a yield of LA as high as 46.60%.
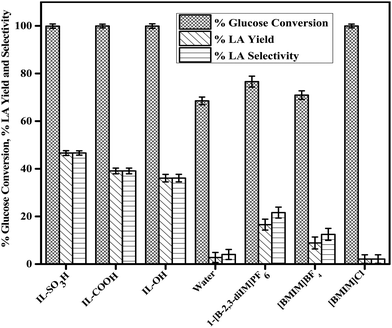 |
| Fig. 2 Effect of different ionic liquids on the catalytic conversion of glucose. Reaction conditions: 100 mg of glucose, 400 mg of ionic liquid, 0.6 mL of water, T = 155 °C, and t = 5 h. | |
3.2 Effects of temperature on LA yield
The effects of reaction parameters were studied using IL-SO3H as the catalyst to determine the optimum reaction conditions for glucose conversion to LA. The reactions were carried out at a temperature range of 140–175 °C. From Fig. 3, it is evident that a reaction temperature of 155 °C and a reaction time of 5 h are the optimum reaction conditions for glucose conversion to LA. The maximum glucose conversion and LA yield achieved were 99.90% and 46.60% respectively at 155 °C and 5 h. Fig. 3 illustrates the significant effect of temperature and time on the yield of LA. At a lower reaction temperature of 140 °C, the maximum glucose conversion and the yield of LA were obtained to be 99% and 43%, respectively. This can be attributed to the formation of HMF at a lower temperature rather than the rehydrated product LA that requires a higher reaction temperature. However, at higher temperatures of 165 °C and 175 °C, the yield of LA further decreased due the formation of humins and other side products.30,34 Hence, it can be concluded that 155 °C is the optimum temperature for the conversion of glucose to LA, because at lower temperatures HMF resists rehydration to LA and at higher temperatures side reactions occur.
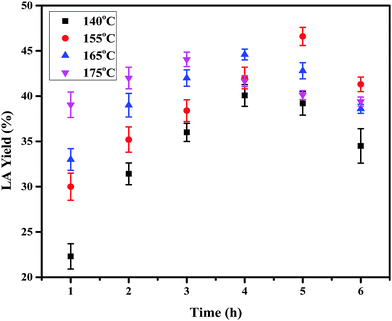 |
| Fig. 3 Effects of reaction temperature and reaction time on the conversion of glucose to LA using IL-SO3H as the catalyst. Reaction conditions: 100 mg of glucose, 400 mg of ionic liquid, 0.6 mL of water. | |
3.3 Effects of transition metal chlorides as co-catalysts on LA yield
A series of reactions were performed in order to gain a better perspective of the effect of metal chlorides as a co-catalyst with the ionic liquid, IL-SO3H. Around 1.8 wt% metal chloride salts were added in the reaction mixture as the co-catalyst, and the reaction was conducted for 5 h at a reaction temperature of 155 °C. Table 2 summarizes the performance of the IL-SO3H with different transition metal chlorides as the co-catalyst in the conversion of glucose to LA. From Table 2, it is clear that in the absence of metal chlorides, 100% glucose conversion and 46.60% yield of LA were obtained at a reaction temperature of 155 °C within a reaction time of 5 h. Moreover, in the presence of only water, around 68.52% glucose conversion was obtained with only 2.76% yield of levulinic acid (LA). However, in the presence of catalytic amounts of metal chlorides such as FeCl3, CoCl2, NiCl2, CuCl2 and ZnCl2, higher catalytic activity was obtained as compared to IL-SO3H. In the case of NiCl2, a maximum LA yield of 49.65% was obtained with LA selectivity as high as 49.68%. However, not all metal chlorides were effective co-catalysts. The conversion of glucose and the yield of LA using these metal chlorides were found to be in the order: water < FeCl3 < IL-SO3H < CuCl2 < CoCl2 < ZnCl2 < NiCl2 under the same reaction conditions. Thus the selectivity towards LA followed the order: water < FeCl2 < IL-SO3H < CuCl2 < CoCl2 < ZnCl2 < NiCl2. Based on the results shown in Table 2, it is evident that the IL-SO3H zwitterionic form is highly stable and it shows acidity via in situ HCl. This is evident from the experimentally obtained incremented yield of LA (46.60%) at 155 °C within a reaction time of 5 h. However, addition of similar types of metal chloride salts cannot affect significant changes in the LA yield except in the case of NiCl2. These results further imply that nickel acts as an active metal and as a co-catalyst, and hence, it plays an important role in glucose conversion and LA yield as compared to other transition metals used in this study. Moreover, in addition to the in situ formation of HCl, metal chlorides in aqueous solution similarly produce metal hydrate complexes. Hence, the regeneration possibility of a similar acidity in the aqueous phase reaction mixture causes less affectivity of the metal chlorides in the reaction system. A plausible reaction mechanism is proposed based on the catalytic activity results as shown in Fig. 4 to summarize all the possible observations and experimental outcomes in order to explain the effect of these metal chlorides (Co, Ni, Cu and Zn) as co-catalysts in combination with Bronsted acidic ionic liquids.31 The metal salts in the complex form [MClx(H2O)n](x−2)− bind with the –OH groups of the glucose molecule through hydrogen bonding. A similar mechanism is followed in the case of FeCl3 as the co-catalyst in the complex form [MClx(H2O)n](x−1)−. The hydrated metal salt complex promotes the rapid conversion of the α-anomers of glucose to the β-anomers through hydrogen bonding between the Cl-atom of the complex and the –OH group of glucose. However, the extent of hydrogen bonding ability of the Cl-atom is not high enough to alter the process to a greater extent. Hence, metal halides were also found to be less active in the aqueous phase, and the overall outcomes of the reaction were also mentioned in previous literature reports.35
Table 2 Effect of metal chlorides on the conversion of glucose and the yield of LA
Catalysts |
LA yield (%) |
LA selectivity (%) |
Glucose conversion (%) |
Reaction conditions: 100 mg of glucose, 400 mg of ionic liquid, 0.6 mL of water, T = 155 °C, t = 5 h, and the amount of MClx used = 1.8 wt%. |
Water |
2.76 |
4.02 |
68.52 |
IL-SO3H |
46.60 |
46.64 |
99.90 |
IL-SO3H + FeCl3 |
37.24 |
37.29 |
99.86 |
IL-SO3H + CoCl2 |
47.32 |
47.35 |
99.92 |
IL-SO3H + NiCl2 |
49.65 |
49.68 |
99.93 |
IL-SO3H + CuCl2 |
47.08 |
47.10 |
99.94 |
IL-SO3H + ZnCl2 |
48.28 |
48.28 |
99.99 |
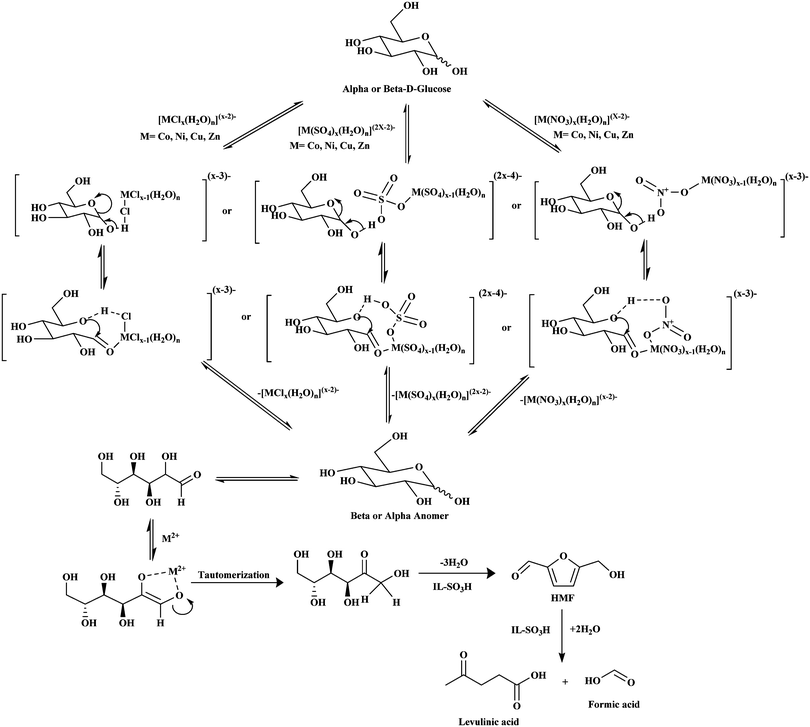 |
| Fig. 4 Plausible reaction mechanism of glucose conversion to LA and formic acid in the presence of metal salts. | |
3.4 Effects of transition metal sulfates as co-catalysts on the LA yield
Furthermore, to investigate the effects of metal sulfates as co-catalysts, along with IL-SO3H on the conversion of glucose and the yield of LA, a series of the transition metal sulfate salts were added to the reaction system to carry out the catalytic reactions at a reaction temperature of 155 °C for 5 h. Several combinations of cations and anions in the reaction system showed significant effects on the LA production as shown in Table 3. The conversion of glucose followed the order: water < CoSO4·7H2O < FeSO4·7H2O < CuSO4·5H2O < IL-SO3H < ZnSO4·7H2O < NiSO4·6H2O. Thus the selectivity to LA followed the order: water < CoSO4·7H2O < FeSO4·7H2O < CuSO4·5H2O < IL-SO3H < ZnSO4·7H2O < NiSO4·6H2O. The catalytic activity trends of the metal sulfates were in the following order: CoSO4·7H2O < FeSO4·7H2O < CuSO4·5H2O < ZnSO4·7H2O < NiSO4·6H2O. The yield of LA varied from 40.37% to 56.33% in the presence of these metal sulfates as co-catalysts as compared to only IL-SO3H catalyzed reactions (46.60%). The first three metal salts showed a negative synergistic effect on the yield of LA. Among the metal sulfates, the IL-SO3H-NiSO4·6H2O system showed the highest synergistic effect in terms of LA yield.
Table 3 Effect of metal sulfates on the conversion of glucose and the yield of LA
Catalysts |
LA yield (mol%) |
LA selectivity (%) |
Glucose conversion (mol%) |
Reaction conditions: 100 mg of glucose, 400 mg of ionic liquid, 0.6 mL of water, T = 155 °C, t = 5 h, and the amount of MSO4·nH2O used = 1.8 wt%. |
Water |
2.76 |
4.02 |
68.52 |
IL-SO3H |
46.60 |
46.64 |
99.90 |
IL-SO3H + FeSO4·7H2O |
41.09 |
41.25 |
99.61 |
IL-SO3H + CoSO4·7H2O |
40.37 |
40.38 |
99.97 |
IL-SO3H + NiSO4·6H2O |
56.33 |
56.37 |
99.92 |
IL-SO3H + CuSO4·5H2O |
41.45 |
41.54 |
99.77 |
IL-SO3H + ZnSO4·7H2O |
52.30 |
52.35 |
99.90 |
Furthermore, as is evident from Table 3, the effect of metal sulfates as co-catalysts is more predominant in this reaction as compared to metal chlorides (as shown in Table 2). The higher activity of the sulfates can be attributed to the reduced side product formation in this reaction. Moreover, these observations indicate that the Bronsted acidity alone is not the sole factor affecting the glucose conversion to LA. The anions play significant roles in the glucose dehydration and rehydration processes to yield LA as per the plausible mechanism shown in Fig. 4. The sulfate salts in the complex form [M(SO4)x(H2O)n](2x−2)− co-ordinate with the –OH groups of the α-anomers of glucose by forming stronger hydrogen bonds than the chloride salts. Consequently, metal sulfates play a significant role in the rapid conversion of the α-anomers to β-anomers to facilitate the dehydration process.
3.5 Effect of transition metal nitrates as co-catalysts on LA yield
To investigate the role of metal nitrate salts as a co-catalyst with IL-SO3H, a series of reactions were performed at a reaction temperature of 155 °C for 5 h. The addition of transition metal nitrates of Fe, Co, Ni, Cu and Zn with IL-SO3H resulted in a negative synergistic effect on the yield of LA as shown in Table 4. The negative synergistic effect for the metal nitrates can be attributed to the formation of more side products via multiple side reactions. The co-catalytic effects of the Cu, Fe, Co and Zn metal nitrates effectively decreased the yield of LA due to the formation of the other side products during the reaction, which is unfavorable for the formation of LA. The decreased yield during the LA synthesis from glucose can be attributed to the formation of soluble polymers and insoluble humins, which are dark-colored tarry solids. The maximum yield of LA drops from 47.16% in the case of Ni to 37.29% in the case of Fe. The conversion of glucose followed the order: water <CuNO3·5H2O < FeNO3·7H2O < CoNO3·7H2O < ZnNO3·7H2O < IL-SO3H < NiNO3·6H2O. Thus the selectivity of LA followed the order: water < CuNO3·5H2O < FeNO3·7H2O < CoNO3·7H2O < ZnNO3·7H2O < IL-SO3H < NiNO3·6H2O. The transition metal nitrate salts in the form [M(NO3)x(H2O)n](x−2)− bind less weakly with the –OH groups of the α-anomers of glucose. Hence, the rate of their conversion to the β-anomers decreases and consequently the conversion of glucose to LA also decreases. Moreover, nitrates are inherently weaker ligands to form a stable coordination bond with the α-anomer. In general, nitrates possess strong oxidizing properties and can oxidize glucose to other insoluble products, which are effectively unfavorable for the conversion of glucose into LA.26 The obtained glucose conversion in the case of nitrates was found to be comparable with the metal chlorides and metal sulfates with a distinct change in the yield of LA. This indicates that the converted glucose formed more side products other than LA. This is also evident from the decreasing glucose conversion and LA yield as shown in Table 4.
Table 4 Effects of metal nitrates in the conversion of glucose and the yield of LA
Catalysts |
LA yield (mol%) |
LA selectivity (%) |
Glucose conversion (mol%) |
Reaction conditions: the amount of MClx used = 1.8 wt%, T = 155 °C, t = 5 h, and the amount of MNO3·nH2O used = 1.8 wt%. |
Water |
2.76 |
4.02 |
68.52 |
IL-SO3H |
46.60 |
46.64 |
99.90 |
IL-SO3H + FeNO3·7H2O |
37.29 |
40.15 |
92.87 |
IL-SO3H + CoNO3·7H2O |
41.10 |
41.17 |
99.82 |
IL-SO3H + NiNO3·6H2O |
47.16 |
47.21 |
99.89 |
IL-SO3H + CuNO3·5H2O |
37.98 |
38.56 |
98.49 |
IL-SO3H + ZnNO3·7H2O |
43.51 |
44.31 |
98.18 |
3.6 Reusability of the catalyst
Reusability of the ionic liquid catalyst was investigated in the glucose conversion to LA with IL-SO3H as well as with IL-SO3H with a Ni metal salt. Ionic liquids were regenerated following the previous reported literature.4,10,26 After completion of the reaction, the reaction mixture was diluted with 2 mL of deionized water to reduce the viscosity. The products were extracted using ethyl acetate, and the rest of the solution contained ionic liquid–water or ionic liquid–Ni metal salt mixtures. Water was removed from the ionic liquid or ionic liquid–Ni metal salt mixture by drying overnight under reduced pressure (0.01 Torr) at 120 °C. The recovered ionic liquids or ionic liquid–Ni metal salt mixtures were subjected to further catalytic runs and the results are summarized in Table 5. The glucose conversion and yield of LA were almost retained for the first three recycling reactions. The decrease in recyclability in further recycling experiments can be attributed to the increasing water content as well as the side products accumulating in each step of the reaction, which required harsh recycling conditions.
Table 5 Recyclability of IL-SO3H and IL-SO3H with a Ni metal salt
No. of runs |
LA yielda (mol%) |
LA selectivitya (%) |
Glucose conversiona (mol%) |
LA yieldb (mol%) |
LA selectivityb (%) |
Glucose conversionb (mol%) |
Reaction conditions: reaction temperature = 155 °C and reaction time = 5 h. LA yield and selectivity and the conversion of glucose in the presence of only IL-SO3H. LA yield and selectivity and the conversion of glucose in the presence of only IL-SO3H with Ni metal salt. |
Fresh run |
46.60 |
46.64 |
99.90 |
56.33 |
56.37 |
99.92 |
Recycle 1 |
42.40 |
44.25 |
95.80 |
51.46 |
55.17 |
93.27 |
Recycle 2 |
39.60 |
46.37 |
85.40 |
47.86 |
57.70 |
82.94 |
Recycle 3 |
37.30 |
46.33 |
80.50 |
43.92 |
55.82 |
78.67 |
4. Conclusions
In this study, glucose conversion to LA was investigated using multifunctional ionic liquids containing –OH, –COOH and –SO3H groups. The ionic liquids were synthesized in the laboratory and characterized using spectroscopic techniques such as 1H NMR and 13C NMR. From the detailed catalytic activity study, the –SO3H functionalized ionic liquid was found to be the most efficient catalyst in terms of LA selectivity and high conversion of glucose. The parameters such as reaction temperature and reaction time were optimized and the maximum LA yield of 46.60% was obtained using IL-SO3H at a temperature of 155 °C within 5 h. From the recyclability experiments, IL-SO3H and the combination of IL-SO3H with a Ni metal salt were found to retain their catalytic activity for up to 3 cycles. Furthermore, to investigate the effect of transition metal salts as co-catalysts along with the IL-SO3H, a series of transition metal chlorides, sulfates and nitrates were added and the reactions were carried out under the optimum reaction conditions. Metal sulfates showed a predominant synergistic effect on the catalytic activity in terms of the LA yield as compared to the chloride and nitrate salts. Among these catalyst and co-catalyst combinations, IL-SO3H–NiSO4 showed the highest catalytic efficiency with the highest LA yield of 56.33% at 155 °C within a reaction time of 5 h with 56.37% LA selectivity. This study is highly beneficial for the development of an efficient catalytic system for glucose conversion into high value platform chemicals.
Conflicts of interest
There are no conflicts to declare.
Acknowledgements
Komal Kumar and Firdaus Parveen thank the University Grants Commission (UGC), India, for a research fellowship. Tanmoy Patra thanks the Council of Scientific and Industrial Research (CSIR) for the research fellowship. Dr Sreedevi Upadhyayula thanks the Indian Institute of Technology, Delhi, for research grants. The authors are also thankful to NRF (Nanoscale Research Facility) for providing the high-pressure liquid chromatography (HPLC) equipment for the detection of LA yield and the conversion of glucose.
References
- V. B. Kumar, I. N. Pulidindi and A. Gedanken, Synergistic catalytic effect of the ZnBr2 –HCl system for levulinic acid production using microwave irradiation, RSC Adv., 2015, 5(15), 11043–11048 RSC.
- F. Yang, J. Fu, J. Mo and X. Lu, Synergy of Lewis and Brønsted acids on catalytic hydrothermal decomposition of hexose to levulinic acid, Energy Fuels, 2013, 27(11), 6973–6978 CrossRef CAS.
- G. Jäger and J. Büchs, Biocatalytic conversion of lignocellulose to platform chemicals, Biotechnol. J., 2012, 7(9), 1122–1136 CrossRef PubMed.
- J. Fu, X. Xu, X. Lu and X. Lu, Hydrothermal decomposition of carbohydrates to Levulinic acid with catalysis by ionic liquids, Ind. Eng. Chem. Res., 2016, 55(42), 11044–11051 CrossRef CAS.
- Z. Zhang, Synthesis of γ-Valerolactone from carbohydrates and its applications, ChemSusChem, 2016, 9(2), 156–171 CrossRef CAS PubMed.
- A. Mukherjee, M.-J. Dumont and V. Raghavan, Review: Sustainable production of Hydroxymethylfurfural and levulinic acid: Challenges and Opportunities, Biomass Bioenergy, 2015, 72, 143 CrossRef CAS.
- C. Chun, M. A. Xiaojian and C. Peilin, Kinetics of Levulinic acid formation from glucose decomposition at high temperature, Chin. J. Chem. Eng., 2006, 14(5), 708 CrossRef.
- X. Cao, X. Peng, S. Sun, L. Zhong, W. Chen, S. Wang and R.-C. Sun, Hydrothermal conversion of xylose, glucose, and cellulose under the catalysis of transition metal sulfates, Carbohydr. Polym., 2015, 118, 44 CrossRef CAS PubMed.
- H. Li, Q. Zhang, X. Liu, F. Chang, Y. Zhang, W. Xue and S. Yang, Immobilizing Cr3+ with SO3H-functionalized solid polymeric ionic liquids as efficient and reusable catalysts for selective transformation of carbohydrates into 5-Hydroxymethylfurfural, Bioresour. Technol., 2013, 144, 21 CrossRef CAS PubMed.
- Y. Shen, J.-K. Sun, Y.-X. Yi, B. Wang, F. Xu and R.-C. Sun, One-pot Synthesis of Levulinic acid from cellulose in ionic liquids, Bioresour. Technol., 2015, 192, 812 CrossRef CAS PubMed.
- F. F. Chen, K. Huang, Y. Zhou, Z.-Q. Tian, X. Zhu, D.-J. Tao, D. Jiang and S. Dai, Multi-Molar Absorption of CO2 by the Activation of Carboxylate Groups in Amino Acid Ionic Liquids, Angew. Chem., Int. Ed., 2016, 55(25), 7166 CrossRef CAS PubMed.
- D. J. Tao, F. F. Chen, Z. Q. Tian, K. Huang, S. M. Mahurin, D. E. Jiang and S. Dai, Highly Efficient Carbon Monoxide Capture by Carbanion-Functionalized Ionic Liquids through C-Site Interactions, Angew. Chem., Int. Ed., 2017, 56(24), 6843 CrossRef CAS PubMed.
- F. Ouyang, Z. Z. Wang, Y. Zhou, Z. Cheng, Z. H. Lu, Z. Yang and D. J. Tao, Highly Efficient and Selective Synthesis of Dibutyl Carbonate via the Synergistic Dual Activation Catalysis of Tetraethylammonium Prolinate Ionic Liquids, Appl. Catal., A, 2015, 492, 177 CrossRef CAS.
- D. J. Tao, F. Ouyang, Z. M. Li, N. Hu, Z. Yang and X. S. Chen, Synthesis of Tetrabutylphosphonium Carboxylate Ionic Liquids and Its Catalytic Activities for the Alcoholysis Reaction of Propylene Oxide, Ind. Eng. Chem. Res., 2013, 52(48), 17111 CrossRef CAS.
- I. K. M. Yu and D. C. W. Tsang, Conversion of biomass to hydroxymethylfurfural: A review of catalytic systems and underlying mechanisms, Bioresour. Technol., 2017, 238, 716 CrossRef CAS PubMed.
- N. A. S. Ramli and N. A. S. Amin, A New functionalized ionic liquid for efficient glucose conversion to 5-Hydroxymethyl furfural and Levulinic acid, J. Mol. Catal. A: Chem., 2015, 407, 113 CrossRef CAS.
- A. C. Cole, J. L. Jensen, I. Ntai, K. L. T. Tran, K. J. Weaver, D. C. Forbes and J. H. Davis, Novel Bronsted Acidic ionic liquids and their use as dual solvent-catalysts, J. Am. Chem. Soc., 2002, 124(21), 5962 CrossRef CAS PubMed.
- J. Gui, H. Ban, X. Cong, X. Zhang, Z. Hu and Z. Sun, Selective alkylation of phenol with tert-butyl alcohol catalyzed by Brönsted acidic imidazolium salts, J. Mol. Catal. A: Chem., 2005, 225(1), 27 CrossRef CAS.
- J. Gui, X. Cong, D. Liu, X. Zhang, Z. Hu and Z. Sun, Novel Brønsted acidic ionic liquid as efficient and reusable catalyst system for esterification, Catal. Commun., 2004, 5(9), 473 CrossRef CAS.
- J. Fraga-Dubreuil, K. Bourahla, M. Rahmouni, J. P. Bazureau and J. Hamelin, Catalysed esterifications in room temperature ionic liquids with acidic counteranion as recyclable reaction media, Catal. Commun., 2002, 3(5), 185 CrossRef CAS.
- K. Kondamudi, P. Elavarasan, P. J. Dyson and S. Upadhyayula, Alkylation of p-cresol with tert-butyl alcohol using benign Bronsted acidic ionic liquid catalyst, J. Mol. Catal. A: Chem., 2010, 321(1–2), 34 CrossRef CAS.
- T. Patra, S. Ahamad and S. Upadhyayula, Highly efficient alkylation of phenol with tert-butyl alcohol using environmentally benign Bronsted acidic ionic liquids, Appl. Catal., A, 2015, 506, 228 CrossRef CAS.
- M. Mirzai and H. Valizadeh, Microwave-promoted synthesis of 3,4-Dihydropyrimidin-2(1H)-(Thio)ones using IL-ONO as recyclable base catalyst under solvent-free conditions, Synth. Commun., 2012, 42(9), 1268 CrossRef CAS.
- F. Parveen, T. Patra and S. Upadhyayula, Hydrolysis of microcrystalline cellulose using functionalized Bronsted acidic ionic liquids – A comparative study, Carbohydr. Polym., 2016, 135, 280 CrossRef CAS PubMed.
- Y. Shen, J.-K. Sun, Y.-X. Yi, B. Wang, F. Xu and R.-C. Sun, One-pot synthesis of Levulinic acid from cellulose in ionic liquids, Bioresour. Technol., 2015, 192, 812 CrossRef CAS PubMed.
- F. Tao, H. Song and L. Chou, Efficient Conversion of Cellulose into Furans Catalyzed by Metal Ions in Ionic Liquids, J. Mol. Catal. A: Chem., 2012, 357, 11 CrossRef CAS.
- G. P. Smith, A. S. Dworkin, R. M. Pagni and S. P. Zingg, Bronsted superacidity of HC1 in a liquid chloroaluminate A1C13-1-Ethyl-3-Methyl-1H-Imidazolium chloride, J. Am. Chem. Soc., 1989, 111, 525 CrossRef CAS.
- D. King, R. Mantz, R. Osteryoung, N. Carolina, R. V. June, V. Re, M. Recei and V. August, Acidity of HCl in neutral buffered chloroaluminate molten salts, J. Am. Chem. Soc., 1996, 118(47), 11933–11938 CrossRef CAS.
- C. Thomazeau, H. Olivier-Bourbigou, L. Magna, S. Luts and B. Gilbert, Determination of an acidic scale in room temperature ionic liquids, J. Am. Chem. Soc., 2003, 125(18), 5264–5265 CrossRef CAS PubMed.
- K. Kondamudi, P. Elavarasan, P. J. Dyson and S. Upadhyayula, Alkylation of p-Cresol with tert-Butyl Alcohol Using Benign Bronsted Acidic Ionic Liquid Catalyst, J. Mol. Catal. A: Chem., 2010, 321, 34 CrossRef CAS.
- Z. Duan, Y. Gu and Y. Deng, Green and Moisture-stable lewis acidic ionic liquids (Choline chloride·xZnCl2) catalyzed protection of carbonyls at room temperature under solvent-free conditions, Catal. Commun., 2006, 7(9), 651 CrossRef CAS.
- S. M. Kumbar, G. V. Shanbhag, F. Lefebvre and S. B. Halligudi, Heteropoly acid supported on titania as solid acid catalyst in alkylation of p-Cresol with tert-butanol, J. Mol. Catal. A: Chem., 2006, 256(1–2), 324–334 CrossRef CAS.
- J. Li, J. Li, D. Zhang and C. Liu, Theoretical elucidation of glucose dehydration to 5-Hydroxymethylfurfural catalyzed by a SO3H-functionalized ionic liquid, J. Phys. Chem. B, 2015, 119(42), 13398 CrossRef CAS PubMed.
- A. Bayu, G. Guan, S. Karnjanakom, X. Hao, K. Kusakabe and A. Abudula, Catalytic synthesis of levulinic acid and formic acid from glucose in choline chloride aqueous solution, ChemistrySelect, 2016, 1(2), 180–188 CrossRef CAS.
- Y. J. Pagan-Torres, T. Wang, J. M. R. Gallo, B. H. Shanks and J. A. Dumesic, Production of 5-Hydroxymethylfurfural from glucose using a combination of Lewis and Bronsted acid catalysts in water in a biphasic reactor with an alkylphenol solvent, ACS Catal., 2012, 2(6), 930–934 CrossRef CAS.
|
This journal is © The Royal Society of Chemistry and the Centre National de la Recherche Scientifique 2018 |