Potential for city parks to reduce exposure to BTEX in air†
Received
5th June 2018
, Accepted 6th November 2018
First published on 7th November 2018
Abstract
Benzene, toluene, ethylbenzene, and xylenes (BTEX) are hazardous air pollutants commonly found in outdoor air. Several studies have explored the potential of vegetation to mitigate BTEX in outdoor air, but they are limited to a northern temperate climate and their results lack consensus. To investigate this subject in a subtropical climate, we deployed passive air samplers for two weeks in parks and outside nearby residences at four locations: three in an urban area and one in a rural area in Alabama, USA. All BTEX concentrations were below health-based guidelines and were comparable to those found in several other studies in populated settings. Concentrations of TEX, but not benzene, were 3–39% lower in parks than at nearby residences, and the differences were significant. Site type (park vs. residential) was a significant predictor of TEX concentrations, while distance to the nearest major road was a significant predictor of BTX concentrations. In and around two of the parks, toluene
:
benzene ratios fell outside the range expected for vehicular emissions (p < 0.01), suggesting that there were additional, industrial sources of benzene near these two locations. The ratio of m-,p-xylene
:
ethylbenzene was high at all locations except one residential area, indicating that BTEX were freshly emitted. Concentrations of individual BTEX compounds were highly correlated with each other in most cases, except for locations that may have been impacted by nearby industrial sources of benzene. Results of this study suggest that parks can help reduce exposure to TEX by a modest amount in some situations.
Environmental significance
Benzene, toluene, ethylbenzene, and xylenes (BTEX) in the atmosphere can be harmful to human health and are precursors to the formation of secondary organic aerosol and ozone. Vegetation has been shown to reduce concentrations of BTEX in chamber studies, but its potential to affect concentrations in outdoor air is not well known. In this work, BTEX levels were quantified in four parks and their surrounding residential areas in Alabama. Levels of TEX, but not benzene, were 3–39% lower in parks compared to residential areas, and the difference was significant. This work shows that parks can help reduce exposure to certain air pollutants by a modest amount.
|
Introduction
The hazardous air pollutants benzene, toluene, ethylbenzene, and ortho-, meta-, and para-xylene (BTEX) can comprise over 60% of anthropogenic non-methane organic compounds in the atmosphere in some locations.1 BTEX are present in a wide variety of commercial and industrial products including gasoline, solvents, paints, and varnishes.2–5 Benzene is a known carcinogen,6 and all BTEX compounds have noncancerous health effects. Chronic BTEX exposure has been associated with adverse health outcomes in the cardiovascular, respiratory, and endocrine systems, even at levels below the inhalation reference concentrations set by the US Environmental Protection Agency (EPA).7
In the United States, 35–47% of emissions of each BTEX compound come from onroad sources such as automobiles, compared to 0.4–0.7% from stationary point sources, according to the US Environmental Protection Agency's 2014 National Emissions Inventory. For TEX, onroad emissions are the predominant source, whereas for benzene, nonpoint emissions are higher (41%, compared to 35% onroad). In urban environments, concentrations of individual BTEX compounds are often highly correlated with each other,8–11 reflecting a common source of the pollutants. Additionally, analyses of toluene
:
benzene (T
:
B) and m-,p-xylene
:
ethylbenzene (m-,p-X
:
E) ratios provide information on source type and photochemical age. A T
:
B ratio of 1.5–3.0 is typical of emissions from traffic.9,12–14 A m-,p-X
:
E ratio of approximately 3.6 is expected for fresh emissions, regardless of source,15 while a lower ratio suggests photochemical aging since m-xylene and p-xylene degrade faster than ethylbenzene.16
Phytoremediation is the remediation of soil, water, and air quality through the implementation of vegetative systems. In the usual application of phytoremediation for BTEX, plants and trees are used to remediate contaminated groundwater, where the major removal pathway is evapotranspiration.17–19 However, vegetation can also remove BTEX from air. Several studies have assessed the capacity of house plants for uptake and removal of gas-phase BTEX in small chambers. In one study, plants in a 0.216 m3 chamber removed benzene from air at a rate of 40–88 mg m−3 d−1.20 Sriprapat and Thiravetyan (2013)21 demonstrated that BTEX at concentrations of ∼80 mg m−3 (roughly 1000 times higher than typical ambient concentrations) were removed by Zamioculcas zamiifolia at rates of 0.075–0.098 μg per m2 of plant leaf after 72 hours. In a separate chamber study, household plants exposed to benzene at much lower concentrations of 405–1380 μg m−3 removed 48–90% of benzene.22 In an open-top chamber experiment on grass (Lolium perenne), exposure to 250 μg m−3 of benzene yielded an in-grass concentration of 0.55 ± 0.08 ng g−1 after 24 hours.23
Uptake of BTEX by plants depends on several competing factors. Uptake from the air occurs through a plant's leaves, stem, and even rhizosphere. In leaves, the pollutants are absorbed primarily through the stomata-containing abaxial (bottom) side, although adaxial (top) absorption occurs as well.21,24 BTEX uptake is also attributable to the plant soil and its microbes, which accounted for 8.1 to 20.4% of uptake of benzene over 24 hours in one study22 and 50.5 to 53.0% of uptake of xylenes in another,25 even after the plant was removed.22
Studies have assessed concentrations of volatile organic compounds (VOCs) in and around city parks, all in a northern temperate climate. Upmanis et al. (2001)26 reported that concentrations of benzene and toluene were at least one-third lower inside parks compared to built-up areas in two Scandinavian cities. Thorsson and Eliasson (2006)27 profiled benzene concentrations inside a large urban park in Sweden and found that the reduction of benzene inside the park was more pronounced in May, when leaves were present, than in February, when leaves were not present. Setälä et al. (2013)28 observed that the total VOC concentration was significantly lower inside vs. outside the tree canopy by 19% in August (i.e., full leaf cover) but not in March (i.e., leaf-free) in two cities in Finland, although the totality of their results suggested that the ability of vegetation to remove air pollutants is minor. In a study in Finland contrasting tree-covered and open areas, Yli-Pelkonen et al. (2017)29 concluded that urban forests did not reduce total anthropogenic VOC levels. Focusing on urban parks in Sweden, Klingberg et al. (2017)30 found that the VOC phenanthrene was not consistently higher or lower before and after leaf emergence. The sum of these results indicate that urban parks play at most a small role in reducing ambient VOC concentrations, and some of the studies attributed part of the effect to greater distance from vehicular sources inside parks.
Studies beyond the northern temperate climate are needed to ascertain the potential of vegetation to reduce ambient BTEX concentrations. The objective of this study is to test the hypothesis that city parks can mitigate BTEX in air and therefore reduce exposure to the compounds. As part of a larger investigation of heat exposure,31–34 this study assesses whether parks have a measureable, beneficial effect on ambient BTEX concentrations. The analysis considers proximity to nearby vehicular and industrial sources. The study took place in Alabama, in the cities of Birmingham and Camden, where air pollution has been identified as a top environmental health priority in the underserved populations of these areas.31 An assessment of air toxics in northern Birmingham in 2011–2012 identified BTEX as chemicals of potential concern in this area.35
Materials and methods
Passive samplers
Air samples were collected using diffusion-based organic vapor monitors (OVM; 3500, 3M Occupational Health and Environmental Safety Division, St. Paul, MN) deployed over 7 day time periods. The samplers were protected from potential water damage through a rain cover consisting of the top section of a plastic water bottle (Dasani, Atlanta, GA) with the bottom section removed and several holes punched on the sides for ventilation, as shown in Fig. 1S in ESI.† An iButton temperature sensor (DS1923, Maxim Integrated Products, Inc., San Jose, CA) was also positioned inside the plastic housing.
Sample locations
Two cities in Alabama were selected for sampling: Birmingham (33.527° N, 86.799° W; area of 384.8 km2; population of 212
424 in 2016 (ref. 36)) in Jefferson County and Camden (31.998° N, 87.289° W; area of 10.87 km2; population of 2560 in 2016 (ref. 36)) in rural Wilcox County. They are part of a larger study of heat exposure.31–34 Four parks in residential areas were selected for sampling on the basis of input from local community members, proximity to large point sources, and accessibility. As shown in Fig. 1A, North Birmingham Park and Harriman Park are located in the northern part of Birmingham; North Birmingham Park is approximately 2.25 km west-southwest of Harriman Park park (“Harriman Park” is the name of the neighborhood that surrounds the park included in this study; the label “park” or “residential” will follow the name of the neighborhood for further clarification). Woodward Park is located in southwestern Birmingham. As shown in Fig. 1B, Westgate Park is in Camden and served as a background site; henceforth, this location will be simply referred to as “Camden.”
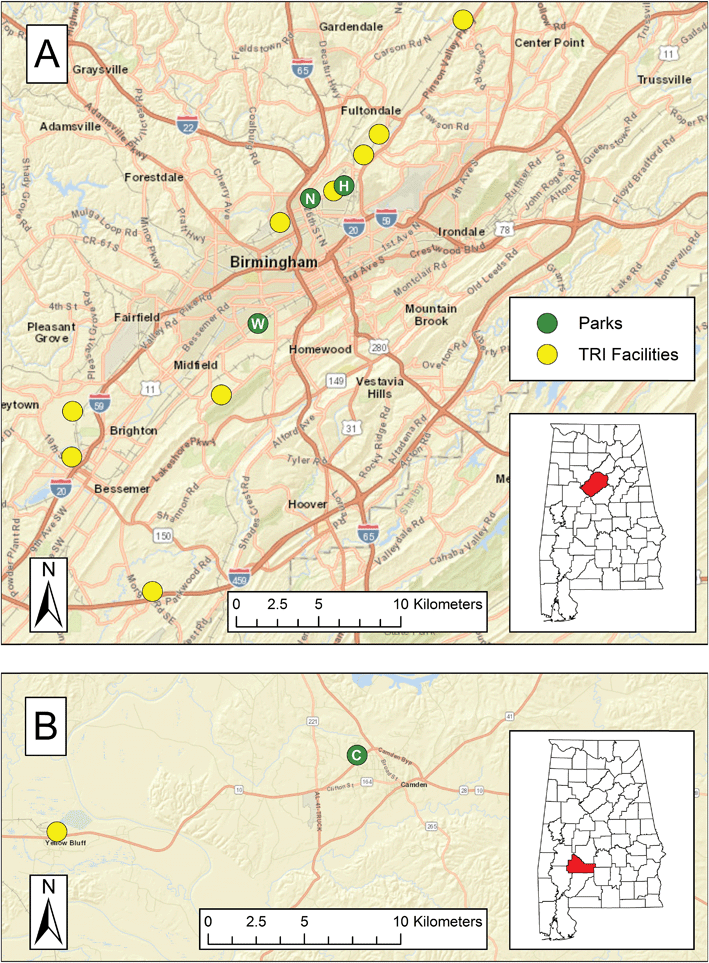 |
| Fig. 1 Parks selected for sampling and point sources of BTEX in 2016 in (A) Birmingham, AL and (B) Camden, AL. Labels denote specific parks, where “N” is North Birmingham, “H” is Harriman Park, “W” is Woodward, and “C” is Camden. | |
Characteristics of each park were derived from aerial imagery. North Birmingham Park (approximately 62
700 m2) is primarily covered in grass and has approximately 110 trees. By contrast, the much smaller Harriman Park park (4290 m2) has approximately 15 trees. Roughly half of the land defined as Harriman Park park is grassy while the other half is covered by pavement or buildings. Woodward Park (43
700 m2) is almost entirely covered in grass and has approximately 100 trees. The park in Camden (28
200 m2) is mostly grassy and has approximately 43 trees. All the parks have some combination of a community center, parking lot, picnic shelter, bathroom, sports courts or fields, swimming pool, and playground.
The sampling locations in Birmingham and Camden were evaluated for potential influence of BTEX sources. Line sources were identified through aerial imagery while the nearest point sources were identified in the EPA's Toxics Release Inventory (TRI); these TRI facilities are mapped in Fig. 1. North Birmingham Park is bordered by a railroad line along its eastern edge for ∼400 m, and the park is ∼900 m from an interstate highway at its closest distance. Furthermore, the TRI shows that North Birmingham Park is 2.4 km northeast of a cast iron pipe manufacturer, 1.5 km southwest of a coke production plant, and 3.9 km southwest of a second coke plant. These facilities emitted thousands of kilograms of BTX in 2016. Harriman Park is 4.5 km northeast of the pipe manufacturer, 0.72 km east of the former coke production plant, and 1.8 km southwest of the latter coke production plant. The wind roses in Fig. 2S and 3S in ESI† show that the prevailing wind in North Birmingham and Harriman Park varied during the study but was generally northerly. Therefore, these locations were usually downwind of the first coke production plant. The southeastern edge of Woodward Park borders (<100 m) a railroad line, and the park is an approximately 2.4 km upwind of the interstate highway. While Woodward's closest source is an industrial chemical facility 3.5 km north of the park, this facility emitted less than 400 kg of TEX combined in 2016. However, a petroleum-based refined products distributor that is 4.9 km south Woodward emitted hundreds of kilograms of BE and thousands of kilograms of TX in 2016. In Camden, located approximately 180 km southwest, the park is 0.31 km and 0.92 km from two state routes. There is one TRI facility (a paper processing plant) in Wilcox County, 16 km west of the Camden park, which was generally downwind during the study, per the wind roses shown in Fig. 4S and 5S in ESI.† Although it did not emit BTEX from 2008 to 2016 according to the TRI, the EPA 2014 National Emissions Inventory (NEI) estimated that the paper processing plant emits thousands of kilograms of BTEX per year.37
In each of the four locations, samples were collected at three sites in the park and three sites in the nearby residential area. A “location” describes a park with its surrounding residential area, and a “site” is where a VOC sampler is deployed; each location has multiple sampling sites. Two community leaders assisted with identification of the residential sampling sites. Criteria for site selection included proximity to the park and major roads, minimal obstruction of the sampler, and consent of residents for researchers to sample on their property. The residential sites near North Birmingham Park were located along its western edge, approximately 14–24 m from the park. Two residential sites near Harriman Park park were close to its southern edge while one was significantly further northeast; their distances from the park ranged from 51 to 350 m. Likewise, two residential sites were located near the northwestern edge of Woodward Park while one was situated much further southwest at distances of 17–560 m. All residential sites in Camden were north of the park at distances ranging from 45 to 190 m. All sites were along two-lane streets except the distant Woodward residence, which was located along a busy two-lane road close to an intersection with a three-lane road. Railroad lines were located near residential sites in North Birmingham (120–210 m), Harriman Park (120–480 m), and Woodward (110–170 m).
Sample deployment
Air samplers were deployed at sampling sites for two 7 day periods: July 8–15, 2017 (week 1) and July 15–22, 2017 (week 2). Three samplers were placed inside each park (total of 12 per week) and three in the residential area surrounding each park (total of 12 per week). The samplers were placed at a mean height of 2.1 ± 0.29 m above the ground, hanging from trees, fences, or porch frames. A map illustrating sampling sites in the parks and residential areas appears in Fig. 6S in ESI.†
Meteorological conditions
During the field campaign, the average temperatures at the nearest weather stations available through the National Centers for Environmental Information were 26 ± 9.1 °C (mean ± standard deviation) and 28 ± 9.2 °C during weeks 1 and 2, respectively, for Birmingham International Airport and 26 ± 9.6 °C and 27 ± 10 °C for Selma 6 SSE, the nearest station to Camden. Precipitation ranged from 3 to 35 mm on three days in Birmingham and from 0.5 to 16 mm on five days near Camden during the two week period. Table 1S in ESI† lists daily temperatures and precipitation amounts during the field campaign, while Fig. 2S–5S in ESI† present wind roses. Wind direction was variable but generally northerly in Birmingham and southeasterly in Camden.
Sample analysis
Samplers were capped and stored at 4 °C between sampling and analysis. The desorption protocol roughly followed the NIOSH Manual of Analytical Methods #4000 (ref. 38) but was adjusted to maximize desorption efficiency. Briefly, each sampler was disassembled and the adsorbent pad was placed in a 2 mL gas chromatography (GC) amber vial with tweezers. 30 μL of a surrogate standard containing 5 μg mL−1p-bromofluorobenzene (CAS no. 460-00-4) was injected onto the sampler's adsorbent pad, and the sealed vial was left to stand overnight in a fume hood at room temperature. In the morning, carbon disulfide (CAS no. 75-15-0) was added to the vial to bring the total solution volume to 1.5 mL; then, the vial was agitated every 20 minutes for 2 hours. Finally, a 1 mL aliquot of the solution was extracted and placed in a separate 2 mL GC vial for analysis by a gas chromatograph-mass spectrometer (Thermo-Fisher Trace GC, DSQ II).
Quality assurance and quality control
A BTEX standard (Restek, 200 μg mL−1 in methanol) was diluted in carbon disulfide (Sigma Aldrich ReagentPlus®, low benzene, ≥99.9%) to produce a six-point calibration curve. The method detection limit (MDL) was determined according to EPA-established guidelines.39 MDLs, reported in full in Table 2S in ESI,† were all below 31.4 ng mL−1 in the extract, corresponding to a gas-phase concentration of 0.132 μg m−3 for a 7 day sampling period. Benzene and toluene had the highest MDLs while those for ethylbenzene and xylenes were lower by a factor of 5–10. Desorption efficiency tests were conducted in triplicate at five concentrations according to the NIOSH Manual of Analytical Methods #4000 for toluene. One standard concentration was below the MDL and was therefore discarded, resulting in four usable concentrations. All BTEX compounds were recovered at close to 100% efficiency, as shown in Table 3S and Fig. 7S in ESI.† Overall, toluene had the highest recovery (118 ± 13.5%) while ethylbenzene produced the lowest recovery (96.4 ± 15.2%). At low concentrations, the recovery of benzene and toluene was >100%, probably due to the high MDLs for these compounds, while that of ethylbenzene and xylenes was <100%. Recoveries for all BTEX compounds converged on 100% at higher concentrations. Finally, reagent, method, and trip blanks were analyzed (n = 4 each). As shown in Table 4S in ESI,† no BTEX compounds were detected in any blanks except for toluene in method and trip blanks at levels extremely close to the MDL.
For each sample, the surrogate concentration detected was divided by the expected value and is reported as the surrogate recovery efficiency. Although these recovery efficiencies (60.9 ± 6.91%) are lower than expected, results of the desorption efficiency analysis indicated that recovery of p-bromofluorobenzene (73.8 ± 9.27%) may not be directly comparable to BTEX recovery (104 ± 14.7% as a whole); therefore, the BTEX results were not adjusted.
A separate experiment, described in detail in ESI,† was conducted to determine whether the rain covers used to protect the passive samplers might have affected the measurements. Briefly, four passive samplers, two with rain covers and two without, were deployed outside a residence for 7 days. Observed concentrations of all compounds were slightly lower with the rain covers, at most by 10%, but the difference in concentration with and without the rain covers was not significant except for m-,p-xylene (p = 0.02). This was a very limited study that can only rule out a large effect of the rain covers.
Gas-phase concentration calculations
Raw data were obtained from the mass spectrometer through peak detection and integration software (Xcalibur version 2.0.7). The mass adsorbed on each sampler was determined by multiplying the reported concentration in the extract by the injection volume (1 μL), multiplying by the solvent dilution factor used in the desorption step, and converting to micrograms. The effective volume of air sampled for each compound was calculated from the sampler deployment time and effective diffusive sampling rate of the compound according to the manufacturer. Effective passive sampling rates at 25 °C for benzene, toluene, ethylbenzenes, and xylenes were 35.5, 31.4, 27.3, and 27.3 mL min−1. The temperatures experienced by the samplers were 28.6 ± 6.6 °C and 29.6 ± 6.6 °C (mean ± standard deviation) during weeks 1 and 2, respectively, as measured by the collocated iButton temperature monitors. The sample volume was corrected using a multiplicative adjustment factor for temperature. Finally, the mass adsorbed was divided by the effective sample volume to produce average ambient BTEX concentrations.
Statistical analysis
Statistical analyses were conducted in the R programming language (version 3.4.4, R Foundation for Statistical Computing, University of California at Berkeley mirror in the Comprehensive R Archive Network (CRAN), Berkeley, CA). A multiple linear regression was conducted to assess the influence of distinct location, site type, and distance to the nearest major road on BTEX concentrations (lm, stats version 3.4.4). Regression parameters included categorical variables for location (North Birmingham, Harriman Park, and Woodward with Camden serving as the background location for the regression), site type (park or residence), a numerical variable for distance from a road with substantial vehicular traffic (greater than approximately 10 cars per minute), and an intercept. Normality was confirmed for most pollutants grouped by location name and site type through Shapiro–Wilk tests (shapiro.test, stats version 3.4.4). Normality was weakly rejected in North Birmingham residential TEX concentrations (p = 0.02 or higher for each compound), but for consistency, all data were subjected to the same multiple linear regression. Although ambient pollutant concentrations are often lognormally distributed, those observed in the current study may be approximately normally distributed because of the small sample size and/or low geometric standard deviation. A nonparametric Spearman rank correlation analysis (rcorr, Hmisc version 4.1-1) was also conducted to assess correlation between BTEX concentrations within locations. Finally, two one-sided Student's t-tests (t.test, stats version 3.4.4) with a Bonferroni correction (α = 0.025) were conducted on T
:
B ratios to examine whether the ratios were statistically different than the range expected for vehicular emissions. A one-sided t-test was conducted on m-,p-X
:
E ratios to assess whether they were greater than 3.6. Normality was confirmed through Shapiro–Wilk tests for all T
:
B and m-,p-X
:
E ratios except Woodward residential T
:
B ratios, which weakly rejected normality (p = 0.04); therefore, all ratio tests were conducted as parametric t-tests.
Results
BTEX concentrations
BTEX concentrations in and around each park are presented in Fig. 2. Week-long BTEX concentrations were stratified by park name and location type (park vs. residential); the original data are presented in Table 6S in ESI† while the means and standard deviations are presented by week in Tables 7S and 8S in ESI.† Benzene and toluene concentrations were 1.02–2.51 μg m−3 in North Birmingham and Harriman Park, and concentrations of ethylbenzene and xylenes were lower. Toluene in Woodward was highest among all the locations and all the compounds. In Camden, BTEX concentrations were lower than in the other three locations by a factor of 2.3–2.8, which was expected for a small city farther from major highways and point sources. Concentrations were similar or slightly higher in week 2, although this difference was only significant for BTEX in the Camden park and ethylbenzene in the Camden residential samples.
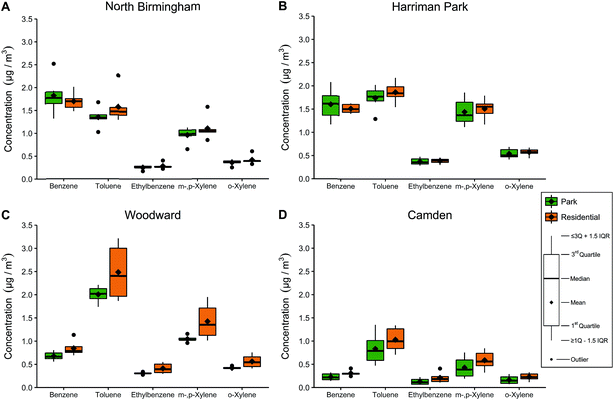 |
| Fig. 2 BTEX concentrations grouped by site type in: (A) North Birmingham, (B) Harriman Park, (C) Woodward, and (D) Camden. Each box represents six data points. Upper whisker is the maximum observation less than 1.5 times the inter-quartile range over the 3rd quartile, while lower whisker is the similar value under the 1st quartile. Outliers are beyond 1.5 times the interquartile range from the 1st and 3rd quartiles. | |
For TEX, concentrations were lower in parks than at nearby residences; the average differences were 6.7–19% for toluene, 3.7–39% for ethylbenzene, 4.5–27% for m-,p-xylene, and 5.8–29% for o-xylene. The differences were smallest in Harriman Park for TEX, while the differences were most pronounced in Camden for EX and Woodward for toluene. Benzene was 20–26% lower in parks in Camden and Woodward but 5.6–7.4% higher in parks in North Birmingham and Harriman Park.
Park effect vs. distance to major roads
Due to motor vehicle emissions, concentrations of VOCs are known to decrease with increasing distance from roads.40 The distance of sampling sites from nearby roads, and thus the impact of vehicle emissions, varied, so a multiple linear regression model was constructed to discern the effect of site type (park vs. residential) from the impact of vehicle emissions. Regression parameter estimates are presented for each BTEX compound in Table 1. Categorical parameters for location were significant (p < 0.02), meaning that BTEX concentrations in each of the four locations were different from each other. Furthermore, site type was a statistically significant parameter for TEX (p < 0.002) but not benzene (p = 0.33). Parks exhibited 0.0736–0.351 μg m−3 lower concentrations of TEX than surrounding residential areas, with the smallest reduction observed for ethylbenzene and the largest observation observed for toluene.
Table 1 Multiple linear regression estimates and p-values. Estimates are in μg m−3 for all parameters except road distance (μg m−3 m−1 from road)
Compound |
Parameter |
Type |
Estimate |
p-Value |
Benzene |
(Intercept) |
— |
0.622 |
0.00035 |
North Birmingham |
Categorical |
1.4 |
<2 × 10−16 |
Harriman Park |
Categorical |
1.05 |
4.10 × 10−10 |
Woodward |
Categorical |
0.319 |
0.0059 |
Inside park |
Categorical |
−0.0615 |
0.332 |
Road distance |
Numerical |
−0.0011 |
0.0207 |
Toluene |
(Intercept) |
— |
1.68 |
7.52 × 10−9 |
North Birmingham |
Categorical |
0.381 |
0.00587 |
Harriman Park |
Categorical |
0.45 |
0.0226 |
Woodward |
Categorical |
1 |
1.75 × 10−7 |
Inside park |
Categorical |
−0.351 |
0.00043 |
Road distance |
Numerical |
−0.0019 |
0.0056 |
Ethylbenzene |
(Intercept) |
— |
0.298 |
5.00 × 10−6 |
North Birmingham |
Categorical |
0.0762 |
0.0218 |
Harriman Park |
Categorical |
0.147 |
0.00283 |
Woodward |
Categorical |
0.141 |
0.00083 |
Inside park |
Categorical |
−0.0736 |
0.00201 |
Road distance |
Numerical |
−0.0003 |
0.0582 |
m-,p-Xylene |
(Intercept) |
— |
1.05 |
4.57 × 10−7 |
North Birmingham |
Categorical |
0.417 |
0.00013 |
Harriman Park |
Categorical |
0.666 |
3.34 × 10−5 |
Woodward |
Categorical |
0.503 |
0.00016 |
Inside park |
Categorical |
−0.253 |
0.0007 |
Road distance |
Numerical |
−0.0014 |
0.00784 |
o-Xylene |
(Intercept) |
— |
0.398 |
4.16 × 10−7 |
North Birmingham |
Categorical |
0.148 |
0.00028 |
Harriman Park |
Categorical |
0.254 |
2.78 × 10−5 |
Woodward |
Categorical |
0.214 |
2.97 × 10−5 |
Inside park |
Categorical |
−0.0983 |
0.0005 |
Road distance |
Numerical |
−0.0005 |
0.00993 |
In the multiple linear regressions, distance to the nearest major road was statistically significant for BTX (p < 0.02) but not for ethylbenzene (p = 0.06). All estimates were negative and estimates for BTX implied that their concentrations decreased by 0.0005–0.0019 μg m−3 per meter from a major road.
BTEX correlations
Correlation and ratio analyses of BTEX concentrations can provide insight into their likely sources and photochemical age. Table 2 presents the Spearman rank correlation coefficients for BTEX by location for the combined weeks. At all sites, TEX concentrations strongly and positively correlated with each other (p < 0.01). Benzene correlated less with TEX, if at all; in Camden, benzene was moderately correlated with TEX (p < 0.05). In Woodward, benzene and EX exhibited a moderately positive (p < 0.05) correlation with each other, but benzene and toluene did not. In North Birmingham, benzene had a moderately positive (p < 0.05) correlation with o-xylene but no other compound. In Harriman Park, benzene was negatively correlated with TEX, but no correlation was statistically significant.
Table 2 Spearman rank correlation coefficients between pollutants, within each site. * denotes significance at p < 0.05. ** denotes significance at p < 0.01. *** denotes significance at p < 0.001
Location |
|
Benzene |
Toluene |
Ethylbenzene |
m-,p-Xylene |
North Birmingham |
Toluene |
0.57 |
|
|
|
Ethylbenzene |
0.57 |
0.91*** |
|
|
m-,p-Xylene |
0.47 |
0.86*** |
0.98*** |
|
o-Xylene |
0.69* |
0.96*** |
0.96*** |
0.92*** |
Harriman Park |
Toluene |
−0.09 |
|
|
|
Ethylbenzene |
−0.28 |
0.87*** |
|
|
m-,p-Xylene |
−0.31 |
0.87*** |
0.99*** |
|
o-Xylene |
−0.29 |
0.90*** |
0.97*** |
0.97*** |
Woodward |
Toluene |
0.48 |
|
|
|
Ethylbenzene |
0.72** |
0.83*** |
|
|
m-,p-Xylene |
0.68* |
0.83*** |
0.99*** |
|
o-Xylene |
0.69* |
0.81** |
0.97*** |
0.99*** |
Camden |
Toluene |
0.64* |
|
|
|
Ethylbenzene |
0.78** |
0.94*** |
|
|
m-,p-Xylene |
0.78** |
0.94*** |
0.99*** |
|
o-Xylene |
0.78** |
0.94*** |
0.99*** |
1*** |
BTEX ratios
Toluene
:
benzene (T
:
B) and m-,p-xylene
:
ethylbenzene (m-,p-X
:
E) mass ratios were calculated for each sample; the results were again stratified by location and site type, as shown in Fig. 3 and Table 9S in ESI.† T
:
B ratios were exceptionally low in North Birmingham (0.666–1.29) and Harriman Park (0.795–1.56) but moderate in Woodward (2.36–4.03) and Camden (2.48–5.10). No T
:
B ratios were observed to be statistically greater than 3.0, but T
:
B ratios in both site types in Harriman Park and North Birmingham were significantly less than 1.5 (p < 0.01).
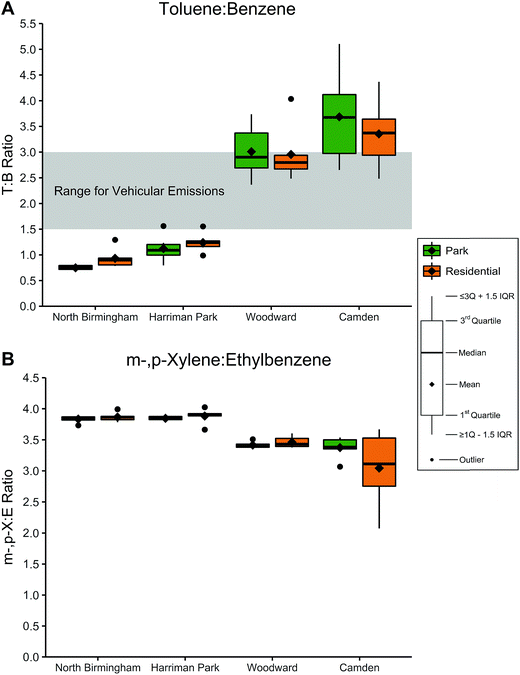 |
| Fig. 3 (A) Toluene : benzene (T : B) ratios by location and site type. (B) m-,p-Xylene : ethylbenzene (m-,p-X : E) ratios by park name and location. Each box represents six data points. Upper whisker is the maximum observation less than 1.5 times the inter-quartile range over the 3rd quartile, while lower whisker is the similar value under the 1st quartile. Outliers are beyond 1.5 times the interquartile range from the 1st and 3rd quartiles. | |
In North Birmingham and Harriman Park, the range of m-,p-X
:
E ratios was 3.7–4.0 while Woodward was slightly lower (3.4–3.6). Ratios in Camden were generally lower and more varied, ranging from 2.1 to 3.7; residential m-,p-X
:
E ratios had a lower mean (3.0) compared to the park ratios (3.4), although this difference was not statistically significant. A one-sided t-test on m-,p-X
:
E ratios showed that ratios in both site types in North Birmingham and Harriman Park were significantly greater than 3.6 (p < 0.01) while ratios in Woodward and Camden were not.
Discussion
Comparison to BTEX in other studies
In the present study, TEX concentrations were below the levels found in outdoor air presented in a review of ambient BTEX7 and below the lowest effect concentrations presented by Herberth et al. (2014)41 and Wallner et al. (2012).42 Benzene concentrations in North Birmingham and Harriman Park were at the lower end of the range described in the review7 and above the lowest concentration at which health effects have been observed.7,41 BTEX concentrations in the present study were mostly below annual means measured in similar studies in Naples, Italy43 and Birmingham, UK,44 although the latter study reported benzene concentrations similar to those found in North Birmingham and Harriman Park. BTEX concentrations were within the ranges found in Windsor, Ontario,10 with the exception of concentrations in Camden being generally lower. Compared to the results of a study in Dunkerque, France during the summertime,45 BTEX levels in North Birmingham, Harriman Park, and Woodward were comparable to those found in urban or industrial settings in France, while levels in Camden were below those documented in a rural setting in France.
Park effect
Regression analysis showed the existence of a park effect in TEX, with lower concentrations in the park compared to the surrounding residential area, even when accounting for distance to the nearest major roads. This finding builds upon prior studies in Scandinavia, where one identified a reduction in concentrations of benzene and toluene in parks26 and another identified a reduction of total VOCs in tree canopies.28 This result is also supported by the results of laboratory chamber experiments that examined uptake of these compounds by plants.21,22,24,25
Several factors likely contributed to the reduction of TEX in parks. First, the size of the park and amount of vegetation are likely significant parameters, as the smallest difference in TEX in parks was found in the smallest park (Harriman Park). Furthermore, the sampling campaign was conducted in the summer when leaf area is at a maximum, providing the optimal interface for plant uptake. Foliage was determined to have a significant influence on BTEX concentrations in large areas of vegetation in several studies,27,28 although a recent study determined that foliage was not significant.29 BTEX can be taken up by soil and its microbes,22,25 and Blume et al. (2002)46 showed that microbial activity increased by up to 83% in summertime temperatures. Finally, the wind speed, as shown in Fig. 2S–5S in ESI,† was relatively low and had significant fractions of calm periods recorded, allowing for any uptake by areas of dense vegetation to register as reduced ambient concentrations.
Benzene did not display a park effect, however. Fig. 2 shows that benzene levels were lower in parks in Woodward and Camden but higher in parks in North Birmingham and Harriman Park. These differences negated each other when combining the entire dataset for the multiple linear regression, which prevented the identification of a park effect for benzene across all locations. Benzene concentrations may have been higher in the parks in North Birmingham and Harriman Park because these two locations are close (1.5 km and 0.72 km, respectively) to a coke production plant that emitted 32
400 kg of benzene in 2016 according to the TRI, and at both locations, the park sites were closer to the coke plant than were the residential sites. Thus, higher concentrations would be expected in the parks if the coke plant is a dominant source of benzene in this area.
Source characterization
Spearman rank correlations presented in Table 2 show that the individual BTEX compounds correlated strongly and significantly with each other across several locations in urban environments, even in different cities. Our analysis suggests that all BTEX came from a shared source in Camden and likely Woodward, while all TEX came from a shared source in North Birmingham and Harriman Park. As onroad emissions are the predominant source of TEX and a major source of benzene,37 it is likely that this shared source was vehicular exhaust. However, there may be a separate source or sources of benzene in North Birmingham and Harriman Park. In hindsight, traffic counts on nearby roads would have been useful to collect during the field campaign and would have strengthened the characterization of sources and analysis of the park effect.
T
:
B ratios indicate that vehicles were likely the dominant source in Camden and Woodward but not in Harriman Park and North Birmingham. The low T
:
B ratios at the latter two sites suggest a separate source of benzene near these locations. Indeed, North Birmingham and Harriman Park are situated between a group of point sources that emit large amounts of benzene, while Woodward and Camden are much further from similar facilities. According to the TRI, the two coke production plants near North Birmingham and Harriman Park have T
:
B ratios in their emissions of 0.582 and 0.492; these values are only slightly lower than the lowest values found in North Birmingham (0.666) and Harriman Park (0.795). Clearly, these industrial sources impacted North Birmingham and Harriman Park. A prior study in Alabama found a small but significant association between preterm birth and residential proximity to coke and steel plants.47
The m-,p-X
:
E ratios in North Birmingham and Harriman Park were significantly greater than 3.6, the value expected for fresh emissions, regardless of source.15 Although the m-,p-X
:
E ratios in Woodward and the park in Camden were slightly lower, these ratios were also higher than most m-,p-X
:
E ratios in urban settings as reported by Miller et al. (2012)10 and Monod et al. (2001).16 Residential ratios in Camden were comparable to the reported value (median ± 2σ) of urban background sites (3.03 ± 0.79 compared to this study's 3.11 ± 1.2).16 Thus, the high m-,p-X
:
E ratios across all locations but the Camden residential sites indicate air masses relatively close to emission sources and yet unaffected by photochemical aging. Exceptionally high ratios in North Birmingham and Harriman Park suggest that these sites were particularly close to BTEX sources.
In summary, most BTEX in Woodward and Camden were likely due to traffic emissions because of the strong correlation between species, moderate T
:
B ratios, and moderate m-,p-X
:
E ratios. In North Birmingham and Harriman Park, TEX were also likely attributable to traffic emissions due to the strong correlation between species. However, there was likely another source of benzene in these locations, as suggested by the low T
:
B ratios and high m-,p-X
:
E ratios. Although BTEX levels were significantly different in each location, the similarity in results for North Birmingham and Harriman Park was likely due to their proximity to both large point sources and to each other.
Community impacts
Importantly, this study considered input from community members in the placement of organic vapor monitors, which allowed for the integration of community science in determining local exposure to BTEX. Upon analysis of the samples, the results were disseminated to the specific community members that participated in the study, along with BTEX ToxGuides from the Agency for Toxic Substances and Disease Registry. An example of the pamphlet shared with participants can be found in Fig. 8S in ESI.† The conveyance of results strengthened the relationship between the researchers and communities and fostered curiosity in air quality, public health, and the scientific method.
Conclusions and future work
Passive samplers were deployed in city parks and neighboring residential areas in two cities in Alabama for two weeks in July 2017 to evaluate whether parks can mitigate exposure to BTEX in air. The measured BTEX concentrations were within the range of expected values for summertime in urban and rural environments. Levels of each BTEX compound were significantly different between locations, and TEX concentrations in parks were 3–39% lower than in the surrounding residential areas. Site type (park vs. residential) was a significant predictor of TEX concentrations, while distance to the nearest major road was a significant predictor of BTX concentrations.
High Spearman rank correlation coefficients suggest a common source or source profile for BTEX in Camden and Woodward and for TEX in North Birmingham and Harriman Park. T
:
B ratios were within the standard range for vehicular emissions in Camden and Woodward but substantially lower in North Birmingham and Harriman Park; in conjunction with the correlation analysis, this suggests that there was a separate, industrial source of benzene nearby. At all sites except residential ones in Camden, the m-,p-X
:
E ratio was sufficiently high to suggest a sampled air mass with a young photochemical age.
Future work should further explore the effect of phytoremediation on BTEX in ambient air in both field and laboratory settings. Field campaigns would benefit by increasing spatial (i.e., sampler density) and/or temporal (i.e., active sampling) resolution to improve statistical power. Analyzing vegetation for evidence of BTEX uptake would also be informative. A short study could be conducted with active samplers to assess the presence of a diurnal pattern in BTEX uptake. Future studies should also involve a sampling campaign over the full year in order to assess seasonal effects, such as loss of deciduous tree leaf cover and change of microbial activity in the rhizosphere. Field studies would also benefit by placing samplers equidistant from roads (both inside and outside of parks) to attempt to equalize the influence from vehicular emissions. Finally, future studies should also include estimates of traffic density for nearby roads.
Conflicts of interest
There are no conflicts to declare.
Acknowledgements
This project was funded by the National Institute of Environmental Health Sciences (5R01ES023029). Additional support was provided by NanoEarth (NSF ECCS-1542100), a node within the National Science Foundation's National Nanotechnology Coordinated Infrastructure (NNCI). The authors thank Molly Richardson, Yuhao Wu, Pranavi Ghugare, Rupkatha Bardhan, and Mary Evans for their assistance in data collection; Councilwoman Sheila Tyson and Mrs Ethel Johnson for their assistance in identifying and securing sampling locations; and Jody Smiley for her assistance in method development and sampler analysis.
References
- S. C. Lee, M. Y. Chiu, K. F. Ho, S. C. Zou and X. Wang, Volatile organic compounds (VOCs) in urban atmosphere of Hong Kong, Chemosphere, 2002, 48, 375–382 CrossRef CAS PubMed.
- L. Fishbein, An overview of environmental and toxicological aspects of aromatic hydrocarbons. I. benzene, Sci. Total Environ., 1984, 40, 189–218 CrossRef CAS.
- L. Fishbein, An overview of environmental and toxicological aspects of aromatic hydrocarbons. II. toluene, Sci. Total Environ., 1985, 42, 267–288 CrossRef CAS PubMed.
- L. Fishbein, An overview of environmental and toxicological aspects of aromatic hydrocarbons. IV. ethylbenzene, Sci. Total Environ., 1985, 44, 269–287 CrossRef CAS PubMed.
- L. Fishbein, An overview of environmental and toxicological aspects of aromatic hydrocarbons. III. xylene, Sci. Total Environ., 1985, 43, 165–183 CrossRef CAS PubMed.
-
International Agency for Research on Cancer, IARC Monographs on the Evaluation of Carcinogenic Risks to Humans: Benzene, World Health Organization, Lyon, France, 2012, vol. 100F Search PubMed.
- A. L. Bolden, C. F. Kwiatkowski and T. Colborn, New look at BTEX: are ambient levels a problem?, Environ. Sci. Technol., 2015, 49, 5261–5276 CrossRef CAS PubMed.
- R. R. Hoque, P. S. Khillare, T. Agarwal, V. Shridhar and S. Balachandran, Spatial and temporal variation of BTEX in the urban atmosphere of Delhi, India, Sci. Total Environ., 2008, 392, 30–40 CrossRef CAS PubMed.
- J. Liu, Y. Mu, Y. Zhang, Z. Zhang, X. Wang, Y. Liu and Z. Sun, Atmospheric levels of BTEX compounds during the 2008 Olympic Games in the urban area of Beijing, Sci. Total Environ., 2009, 408, 109–116 CrossRef CAS.
- L. Miller, X. Xu, A. Grgicak-Mannion, J. Brook and A. Wheeler, Multi-season, multi-year concentrations and correlations amongst the BTEX group of VOCs in an urbanized industrial city, Atmos. Environ., 2012, 61, 305–315 CrossRef CAS.
- M. Miri, M. Rostami Aghdam Shendi, H. R. Ghaffari, H. Ebrahimi Aval, E. Ahmadi, E. Taban, A. Gholizadeh, M. Yazdani Aval, A. Mohammadi and A. Azari, Investigation of outdoor BTEX: concentration, variations, sources, spatial distribution, and risk assessment, Chemosphere, 2016, 163, 601–609 CrossRef CAS PubMed.
- B. Barletta, S. Meinardi, F. S. Rowland, C. Y. Chan, X. Wang, S. Zou, Y. C. Lo and D. R. Blake, Volatile organic compounds in 43 Chinese cities, Atmos. Environ., 2005, 39, 5979–5990 CrossRef CAS.
- A. Gelencsr, K. Siszler and J. Hlavay, Toluene–benzene concentration ratio as a tool for characterizing the distance from vehicular emission sources, Environ. Sci. Technol., 1997, 31, 2869–2872 CrossRef.
- P. Chiang, Y. Chiang, E.-E. Chang and S. Chang, Characterizations of hazardous air pollutants emitted from motor vehicles, Toxicol. Environ. Chem., 1996, 56, 85–104 CrossRef CAS.
- P. F. Nelson and S. M. Quigley, The m,p-xylenes:ethylbenzene ratio. A technique for estimating hydrocarbon age in ambient atmospheres, Atmos. Environ., 1983, 17, 659–662 CrossRef CAS.
- A. Monod, B. C. Sive, P. Avino, T. Chen, D. R. Blake and F. S. Rowland, Monoaromatic compounds in ambient air of various cities: a focus on correlations between the xylenes and ethylbenzene, Atmos. Environ., 2001, 35, 135–149 CrossRef CAS.
- J. G. Burken and J. L. Schnoor, Predictive relationships for uptake of organic contaminants by hybrid poplar trees, Environ. Sci. Technol., 1998, 32, 3379–3385 CrossRef CAS.
- K. K. Baduru, S. Trapp and J. G. Burken, Direct measurement of VOC diffusivities in tree tissues: impacts on tree-based phytoremediation and plant contamination, Environ. Sci. Technol., 2008, 42, 1268–1275 CrossRef CAS.
- S. Trapp, K. C. Zambrano, K. O. Kusk and U. Karlson, A phytotoxicity test using transpiration of willows, Arch. Environ. Contam. Toxicol., 2000, 39, 154–160 CrossRef CAS PubMed.
- R. L. Orwell, R. L. Wood, J. Tarran, F. Torpy and M. D. Burchett, Removal of benzene by the indoor plant/substrate microcosm and implications for air quality, Water, Air, Soil Pollut., 2004, 157, 193–207 CrossRef CAS.
- W. Sriprapat and P. Thiravetyan, Phytoremediation of BTEX from indoor air by Zamioculcas zamiifolia, Water, Air, Soil Pollut., 2013, 224, 1–9 CrossRef CAS.
-
B. C. Wolverton, A. Johnson and K. Bounds, Interior landscape plants for indoor air pollution abatement, NASA Stennis Space Center, MS, USA, 1989 Search PubMed.
- J. Binnie, J. N. Cape, N. Mackie and I. D. Leith, Exchange of organic solvents between the atmosphere and grass – the use of open top chambers, Sci. Total Environ., 2002, 285, 53–67 CrossRef CAS.
- D. Ugrekhelidze, F. Korte and G. Kvesitadze, Uptake and transformation of benzene and toluene by plant leaves, Ecotoxicol. Environ. Saf., 1997, 37, 24–29 CrossRef CAS.
- B. C. Wolverton and J. D. Wolverton, Plants and soil microorganisms: removal of formaldehyde, xylene, and ammonia from the indoor environment, J. Miss. Acad. Sci., 1993, 38, 11–15 Search PubMed.
- H. Upmanis, I. Eliasson and Y. Andersson-Sköld, Case studies of the spatial variation of benzene and toluene in parks and adjacent built-up areas, Water, Air, Soil Pollut., 2001, 129, 61–81 CrossRef CAS.
- S. Thorsson and I. Eliasson, Passive and active sampling of benzene in different urban environments in Gothenburg, Sweden, Water, Air, Soil Pollut., 2006, 173, 39–56 CrossRef CAS.
- H. Setälä, V. Viippola, A.-L. Rantalainen, A. Pennanen and V. Yli-Pelkonen, Does urban vegetation mitigate air pollution in northern conditions?, Environ. Pollut., 2013, 183, 104–112 CrossRef.
- V. Yli-Pelkonen, H. Setälä and V. Viippola, Urban forests near roads do not reduce gaseous air pollutant concentrations but have an impact on particles levels, Landsc. Urban Plann., 2017, 158, 39–47 CrossRef.
- J. Klingberg, M. Broberg, B. Strandberg, P. Thorsson and H. Pleijel, Influence of urban vegetation on air pollution and noise exposure – a case study in Gothenburg, Sweden, Sci. Total Environ., 2017, 599–600, 1728–1739 CrossRef CAS.
- M. C. Bernhard, M. B. Evans, S. T. Kent, E. Johnson, S. L. Threadgill, S. Tyson, S. M. Becker and J. M. Gohlke, Identifying environmental health priorities in underserved populations: a study of rural versus urban communities, Public Health, 2013, 127, 994–1004 CrossRef CAS PubMed.
- M. C. Bernhard, S. T. Kent, M. E. Sloan, M. B. Evans, L. A. McClure and J. M. Gohlke, Measuring personal heat exposure in an urban and rural environment, Environ. Res., 2015, 137, 410–418 CrossRef CAS PubMed.
- C. Y. H. Wu, M. B. Evans, P. E. Wolff and J. M. Gohlke, Environmental health priorities of residents and environmental health professionals: implications for improving environmental health services in rural versus urban communities, J. Environ. Health, 2017, 80, 28–36 Search PubMed.
- E. R. Kuras, M. B. Richardson, M. M. Calkins, K. L. Ebi, J. J. Hess, K. W. Kintziger, M. A. Jagger, A. Middel, A. A. Scott, J. T. Spector, C. K. Uejio, J. K. Vanos, B. F. Zaitchik, J. M. Gohlke and D. M. Hondula, Opportunities and challenges for personal heat exposure research, Environ. Health Perspect., 2017, 125, 85001 CrossRef.
-
US Environmental Protection Agency Region 4, North Birmingham air toxics risk assessment, Environmental Protection Agency Region 4, Atlanta, GA, USA, 2013 Search PubMed.
-
US Department of Commerce, American FactFinder – Community Facts, Washington, DC, USA, 2016 Search PubMed.
-
US Environmental Protection Agency, 2014 National Emissions Inventory, Washington, D.C., USA, 2017 Search PubMed.
-
NIOSH Manual of Analytical Methods, DHHS (NIOSH) Publication, 1994, 4th edn, pp. 94–113 Search PubMed.
-
US Environmental Protection Agency, Definition and procedure for the determination of the method detection limit, revision 2, Environmental Protection Agency Office of Water, Washington, DC, USA, 2016 Search PubMed.
- D. A. Olson, D. M. Hammond, R. L. Seila, J. M. Burke and G. A. Norris, Spatial gradients and source apportionment of volatile organic compounds near roadways, Atmos. Environ., 2009, 43, 5647–5653 CrossRef CAS.
- G. Herberth, M. Bauer, M. Gasch, D. Hinz, S. Röder, S. Olek, T. Kohajda, U. Rolle-Kampczyk, M. von Bergen, U. Sack, M. Borte and I. Lehmann, Maternal and cord blood miR-223 expression associates with prenatal tobacco smoke exposure and low regulatory T-cell numbers, J. Allergy Clin. Immunol., 2014, 133, 543–550 CrossRef CAS PubMed.
- P. Wallner, M. Kundi, H. Moshammer, K. Piegler, P. Hohenblum, S. Scharf, M. Fröhlich, B. Damberger, P. Tappler and H.-P. Hutter, Indoor air in schools and lung function of Austrian school children, J. Environ. Monit., 2012, 14, 1976 RSC.
- P. Iovino, R. Polverino, S. Salvestrini and S. Capasso, Temporal and spatial distribution of BTEX pollutants in the atmosphere of metropolitan areas and neighbouring towns, Environ. Monit. Assess., 2009, 150, 437–444 CrossRef CAS.
- S. Vardoulakis, E. Solazzo and J. Lumbreras, Intra-urban and street scale variability of BTEX, NO2 and O3 in Birmingham, UK: implications for exposure assessment, Atmos. Environ., 2011, 45, 5069–5078 CrossRef CAS.
- J. Roukos, V. Riffault, N. Locoge and H. Plaisance, VOC in an urban and industrial harbor on the French North Sea coast during two contrasted meteorological situations, Environ. Pollut., 2009, 157, 3001–3009 CrossRef CAS PubMed.
- E. Blume, M. Bischoff, J. M. Reichert, T. Moorman, A. Konopka and R. F. Turco, Surface and subsurface microbial biomass, community structure and metabolic activity as a function of soil depth and season, Appl. Soil Ecol., 2002, 20, 171–181 CrossRef.
- T. R. Porter, S. T. Kent, W. Su, H. M. Beck and J. M. Gohlke, Spatiotemporal association between birth outcomes and coke production and steel making facilities in Alabama, USA: a cross-sectional study, Environ. Health, 2014, 13, 85 CrossRef PubMed.
Footnote |
† Electronic supplementary information (ESI) available. See DOI: 10.1039/c8em00252e |
|
This journal is © The Royal Society of Chemistry 2019 |
Click here to see how this site uses Cookies. View our privacy policy here.