DOI:
10.1039/C7AY02103H
(Paper)
Anal. Methods, 2018,
10, 109-122
Fast screening of flavonoids from switchgrass and Mikania micrantha by liquid chromatography hybrid-ion trap time-of-flight mass spectrometry
Received
2nd September 2017
, Accepted 26th November 2017
First published on 11th December 2017
Abstract
Weeds contain flavonoids that have antioxygenation, circulation improvement, and cholesterol-lowering effects. The novel and widely applicable method of liquid chromatography hybrid-ion trap time-of-flight mass spectrometry (LC/MS-IT-TOF) was established to quickly screen non-target flavonoid components from weeds in this study. This method required two steps, the detection of characteristic ions of the flavonoid components and their identification by comparison of their manual fragments. Then all non-target and semi-target flavonoid components could be directly determined via automatically screening out the characteristic fragments. This method was successfully applied for the fast determination of flavonoid components in the stem extract of switchgrass. One glycoside of isorhamnetin (isorhamnetin-3-O-rutinoside) and six glycosides of quercetin (quercetin-3-O-galactoside, quercetin-3-O-glucoside, rutin, quercetin-3-O-xyloside, quercetin-3-O-arabinoside, and quercetin-3-O-rhamnoside) were detected in switchgrass. In addition, syringaresinol hexoside was also found in this weed. Among these compounds, six (quercetin-3-O-galactoside, quercetin-3-O-glucoside, quercetin-3-O-xyloside, quercetin-3-O-arabinoside, isorhamnetin-3-O-rutinoside and syringaresinol hexoside) were first found in switchgrass. In Mikania micrantha, three more flavonoid components were detected than that in our previous study. This efficient method could be universally applied for the identification of components in some complex matrix samples like food samples and plant samples.
1. Introduction
The invasive bunchgrass switchgrass (Panicum virgatum L.), which has adapted to the Loess plateau climate condition, originates from North American and has been introduced to China's Loess Plateau in the past several years. This invasive plant is an energy crop and has hence attracted researchers' attention.1 It took many years for researchers to study switchgrass in yields, productive lowland, resistance to lodging, and so on.2 It has been reported that switchgrass can be used as a biochar to effectively remove phenoxy herbicides from aqueous solutions and it can improve water use efficiency of degraded claypan soil.3,4 In addition, potential of switchgrass in allelopathy and allelochemicals has been investigated.3,5–8 Previous work has reported that switchgrass contains flavonoids having antioxidant effects.9 The compounds responsible for the inhibition of low-density lipoprotein oxidation in switchgrass are identified by LC-MS to be flavonoids such as quercetin (quercetin-3-O-rhamnoside) and rutin (quercetin-3-O-rutinoside).10 However, few studies about all components of flavonoids of this weed have been conducted.
Hybrid-ion trap/time-of-flight mass spectrometry coupled with high-performance liquid chromatography (LC/MS-IT-TOF) using a significantly advanced MS detector provided MSn ability of an IT, high mass accuracy, and high sensitivity of TOF. These advantages have been presented previously. Ten impurities were detected in 2C-E tablets (illegal drugs) using an optimized LC/MS-IT-TOF method according to the exact mass data of MSn, which helped trace the manufacturing source and acquire the synthetic route of the illegal drug.11 Using accurate LC/MS-IT-TOF measurements, the metabolites of Huan-Nao-Yi-Cong-Fang were simultaneously characterized and their structures were elucidated based on accurate MS2 spectra and comparisons of changes in accurate molecular masses and fragmented ions of the metabolites and those of the parent compounds.12 The fraction of flavonoids could be analysed by ESI-MS. Some flavonoids were identified by this powerful technique.13 Flavonoids readily formed ion fragments in MSn mode, and lignans also had the same ion fragments.14,15 Therefore, the ion fragments of compounds need to be verified during the identification of flavonoids.
In a previous report, it was stated that plants contain large numbers of flavonoids. The traditional process for determining flavonoids requires a long time for purification of flavonoids before identifying them, including IR, NMR, and MS. In addition, some trace flavonoids might be ignored because their content were less than the limit of detection.
In the present study, LC/MS-IT-TOF was used to improve the efficiency and sensitivity of flavonoid identification in switchgrass. The novel and widely applicable method of LC/MS-IT-TOF was applied to quickly screen non-target flavonoid components in switchgrass. Key steps were the detection of characteristic ions of flavonoid components and the identification by the comparison of manual fragments. The direct determination of all non-target and semi-target flavonoids was achieved via automatic screening out and classifying them rapidly by extracting ion chromatograms of the diagnostic fragment ions. The analytical time was shortened and the procedure of structural identification was simplified. This efficient method could be universally appropriate for the identification of components in some complex matrix samples like food samples or plant samples.
2. Materials and methods
2.1. Chemicals and plants
HPLC-grade methanol was purchased from Fisher Scientific (Fair Lawn, NJ, USA), and formic acid was obtained from J & K Chemical Ltd (Beijing, China). Water was purified using a Synergy Purification System (Millipore, Molsheim, France). All other chemicals and reagents were of analytical grade.
Switchgrass was provided by Dr. Xincun Hou from the Beijing Academy of Agriculture and Forestry Sciences. Mikania micrantha Kunth was provided and identified by Dr. Guoliang Zhang from the Chinese Academy of Agricultural Sciences.
2.2. Preparation of the crude sample
The stems of the switchgrass were dried and ground with a grinder. Then, the powder (10 g) was refluxed with ethyl acetate (300 mL) for 2 h. The extract of ethyl acetate was concentrated under reduced pressure in a rotary evaporator. The ethyl acetate extract was then dissolved using methanol at a concentration of 20 mg mL−1 and filtered through a 0.22 μm pore size PTFE membrane. A volume of 5 μL supernatant was injected into a LC/MS-IT-TOF analysis system. The preparation of M. micrantha was the same as that of switchgrass.
2.3. LC/MS-IT-TOF conditions
LC experiments were conducted on a Shimadzu (Kyoto, Japan) HPLC system consisting of a solvent delivery pump (LC-20AD), an auto-sampler (SIL-20AC), a DGU-20A3 degasser, a photodiode array detector (SPD-M20A), a communication base module (CBM-20A), and a column oven (CTO-20A). Separation was carried out with an Inertsil ODS-SP column (150 × 4.6 mm I.D.; particle size 5 μm) using a gradient eluent consisting of mobile phase A (0.5% formic acid) and mobile phase B (methanol). Isocratic elution was performed under the following conditions: 0–55 min for 31% B at a flow rate of 0.5 mL min−1 for the crude sample of switchgrass. Linear gradient was performed under the following conditions: 0–50 min from 35% to 40% B at a flow rate of 0.5 mL min−1 for M. micrantha. The sample chamber in the auto-sampler was maintained at 4 °C, while the column was set at 40 °C. The injection volume was 5 μL.
A hybrid ion trap/time-of-flight instrument (Shimadzu Corp., Kyoto, Japan) equipped with an ESI source in negative ion mode was used for the identification of the flavonoid components in switchgrass and M. micrantha. The optimized MS conditions were as follows: nebulizing gas (N2), 1.5 L min−1; drying gas (N2), pressure, 0.1 MPa; CDL temperature, 200 °C; block heater temperature, 200 °C; ion accumulation time, 100 ms; and detector voltage, 1.8 kV. For fragmentation analysis of switchgrass and M. micrantha, mass spectrometric analyses were carried out by full-scan MS with a mass range of 200–1000 Da, while the MSn data were acquired in the range of m/z 50–1000. The software automatically selected precursor ions for MSn analysis according to criterion settings (such as ion intensity). Argon was used as the collision gas and the collision energy was set at 50% for MS2 and 40% for MS3.
External mass calibration was carried out using a trifluoroacetic acid sodium solution (2.5 mM) from 100 to 2000 Da. The flow rate of the infusion pump was 5 mL min−1. The tuning operation was performed in the auto-tuning mode, and the tuning result was saved as a tuning file. All calculated mass errors were less than 10 ppm after mass calibration with the reference standard.
2.4. Flavonoid identification strategy
To quickly screen non-target flavonoid components in switchgrass and M. micrantha and analyse structural characterization by mass spectrometry, a novel strategy was established according to our previous work. This strategy was based on the diagnostic fragment-ion-based extension strategy (DFIBES), which can quickly extract structural information from complicated peaks. The principle is that the compounds with the same carbon skeleton are subjected to a similar fragmentation pathway in the collision-induced dissociation (CID) mode, thus generating a common diagnostic fragment ion (DFI) from the common carbon skeleton.16 Flavonoids were classified into three types (type A, type B, and type C) based on their different DFIs (m/z 151.0037, 165.9908, and 166.9986) in CID mode.17 Therefore, the DFIs were treated as the target to screen and rapidly extract a type of flavonoid from the mixture.
Two steps were needed in this method. First, the MSn information was acquired in the automatic mode to extract the flavonoids in switchgrass and M. micrantha through the extracted ion chromatogram (EIC) obtained by inputting the characteristic ion fragmentation at m/z 151.0037, 165.9908, and 166.9986 into the fragment table at the workstation. Consequently, all the peaks that produced fragmentation at m/z 151.0037, 165.9908, and 166.9986 were classified as type A, B, and C flavonoids, respectively. In the next step, the structures of the flavonoids were verified by the corresponding DFI and the proposed fragmentation pathways obtained by the MSn analysis.
3. Results and discussion
Switchgrass is a kind of invasive weed of Panicum (Gramineae) in China. The investigation of bioactive compounds in this weed is helpful for learning the invasion mechanism. The traditional process takes a long time to identify large numbers of flavonoids in plants and some flavonoids in lower concentrations might be ignored. To improve the efficiency and sensitivity of determining the compounds, LC/MS-IT-TOF was used in the present study.
3.1. Detection and identification of type A flavonoids in switchgrass
As shown in Fig. 1, the characteristic ion fragmentation (m/z 151.0037, C7H3O4−) of type A flavonoids could be determined. The base peak chromatogram (BPC) of each flavonoid molecular ion and EIC of m/z 151.0037 in negative ion mode were presented. Molecular ion peaks were identified by the MS1 spectra, and the aglycone moieties could be surveyed by the MS2 spectra. As previously reported, intense radical fragment ions produced by ESI were due to homolytic glycan cleavage at position 3, but few radical fragment ions could be observed at position 7. Both the positions are glycosylation sites.18–20 Most radical fragment ions were observed at position 3, which might be due to easy glycosylation at this position. Fragment ions were observed for aglycones of flavonoids: [M − H]−m/z 301 or 315 (for quercetin or isorhamnetin, respectively), [M − H − CH2O]− at m/z 271 or 285, [M − H − CH3]−˙ at m/z 300 and [M − H − OCH3]−˙ at m/z 285 (for isorhamnetin), [M − H − H2O − CO]− at m/z 255 (for quercetin), [M − H − CO]−m/z 273 or 287 (for quercetin and isorhamnetin, respectively), [M − H − CO2]−m/z 257 or 271 (for quercetin and isorhamnetin, respectively). The DFI of type A flavonoids was observed at m/z 151, which was formed by Retro-Diels–Alder reaction (RDA). These fragment ions were reported in literature.21–26 In addition, the m/z 463 and 609 could be extracted by the MS1 spectra of peak 3 in BPC. m/z 463 might be produced from m/z 609 according to the structure of rutin. However, m/z 463 was not detected in the MS2 spectra of m/z 609 in the manual mode. It might be that m/z 463 was another precursor ion in the MS1 spectra. The above result indicated the importance of the verification of compounds by comparison of manual fragments. m/z 151.0037 of peak 7 was extracted from the MS2 spectra in the auto mode (Fig. 1), but it was not found in the manual mode (Table 1). The proposed pathways of peak 7 explained that other fragments in the manual mode were attributed to the isorhamnetin 3-O-rutinoside (Fig. 2). In the manual mode, m/z 151.0037 of peak 7 was not found probably due to it being under the detection limit of the intensity of ion with m/z 151.0037, which was cleaved from isorhamnetin-3-O-rutinoside. In the auto mode, the ion of m/z 151.0037 cleaved from other precursor ions might be accumulated at peak 7.
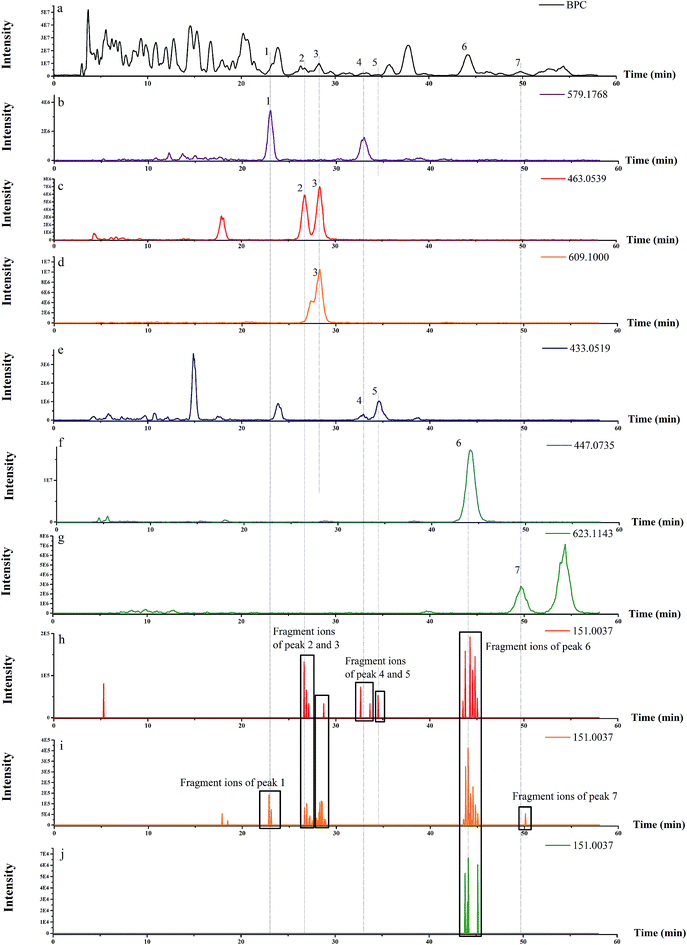 |
| Fig. 1 BPC of switchgrass (a); EIC of MS1 at m/z 463.0539, m/z 609.1000, m/z 623.1143, m/z 447.0735, m/z 433.0519, and m/z 579.1768 (b, c, d, e, f, g, respectively); the MS2, MS3, and MS4 fragment ions at m/z 151.0037 in the auto mode (h, i, j, respectively). | |
Table 1 Investigation of type A flavonoids in switchgrass
Invasive weeds |
Peak no. |
R
t (min) |
[M − H]−m/z |
ESI-MSnm/z (relative intensity %) |
Identification |
Ref. |
Switchgrass |
2 |
26.737 |
463.0557 |
MS2 (463.0557): 301.0153 (100), 300.0078 (71.7) |
Quercetin-3-O-galactoside |
21–23
|
271.0072 (31.92), 255.0143 (17.59), 178.9908 (12.91) |
302.0192 (9.63), 323.0574 (7.12), 150.998 (4.83) |
272.0135 (2.33), 301.1693 (1.99), 301.3454 (1.8) |
243.0169 (1.72), 324.0573 (1.24), 273.0248 (1.1) |
3 |
28.24 |
463.0557 |
MS2 (463.0557): 301.0159 (100), 300.0082 (46.16) |
Quercetin-3-O-glucoside |
21–23
|
271.0072 (23.32), 255.0134 (14.41), 178.9922 (12.83) |
302.0208 (12.4), 150.9986 (3.88), 461.1518 (3.32) |
609.1029 |
MS2 (609.1029): 301.0167 (100), 300.0091 (68.3) |
Rutin |
21
|
271.009 (45.41), 255.0143 (25.82), 302.0208 (25.16) |
611.1170 (9.5), 272.0114 (7.77), 303.0223 (5.49) |
243.0149 (4.75), 256.0184 (4.58), 227.0226 (4.02) |
MS3 (301.0149): 271.0068 (100), 255.0136 (62.44) |
150.9978 (13.1), 178.9886 (2.38), 272.0105 (1.28) |
254.0055 (1.01), 243.0173 (0.94), 254.9658 (0.76) |
4 |
32.642 |
433.0525 |
MS2 (433.0525): 301.016 (100), 300.0157 (36.25) |
Quercetin-3-O-xyloside |
23
|
371.0997 (17.21), 341.0884 (13.02), 150.9972 (5.59) |
178.9802 (4.86), 255.0155 (3.87), 302.0226 (3.13) |
285.0706 (1.33), 271.0036 (0.77) |
5 |
34.498 |
433.0525 |
MS2 (433.0525): 300.0073 (100), 301.0141 (50.76) |
Quercetin-3-O-arabinoside |
23
|
271.0069 (37), 255.0136 (18.57), 178.9942 (5.4) |
302.0281 (3.19), 150.9859 (2.93), 272.0103 (2.63) |
268.0544 (2.2), 254.0073 (2.17) |
MS3 (301.013): 255.0193 (55.08), 271.0109 (100) |
6 |
44.168 |
447.0665 |
MS2 (447.0683): 301.0159 (100), 300.0082 (54.02) |
Quercetin-3-O-rhamnoside |
22 and 23
|
271.0074 (23.26), 302.0208 (20.78), 255.0136 (19.18) |
178.9914 (16.84), 150.9986 (9.57), 301.3475 (3.17) |
301.1713 (3.12), 283.0073 (3.04) |
MS3 [301.0172]: 243.0067 (0.97), 254.0101 (1.01) |
254.9205 (0.78), 255.0143 (57.98), 271.0072 (100) |
271.3404 (0.87), 272.0069 (1.19) |
7 |
49.757 |
623.1179 |
MS2 [623.1174]: 299.0004 (100), 314.0243 (98.46) |
Isorhamnetin-3-O-rutinoside |
24–26
|
315.0294 (73.44), 271.0064 (71.49), 300.0065 (66.61) |
255.0134 (31.04), 243.0150 (18.13), 285.0248 (10.78) |
272.0135 (10.32), 357.0388 (8.01) |
MS3 [315.0291]: 298.9993 (100) |
271.0109 (16.81), 301.0047 (5.61), 300.0136 (3) |
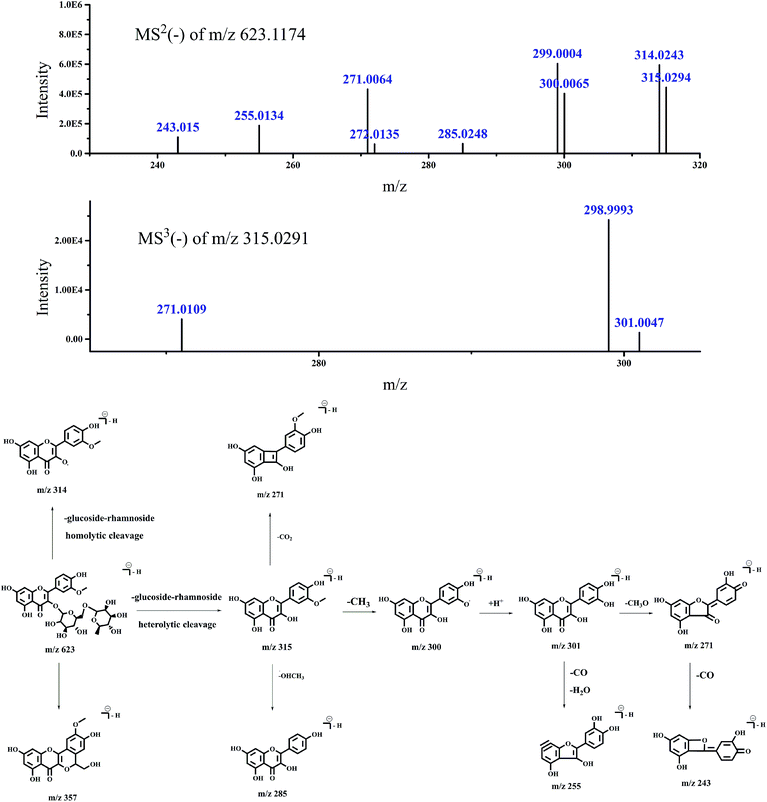 |
| Fig. 2 Accurate MSn spectra and proposed fragmentation pathways of peak 7 in Fig. 1 selected from switchgrass. | |
To demonstrate the steps for identifying flavonoid compounds, the identification of quercetin-3-O-rhamnoside of switchgrass was taken as an example. As shown in Fig. 1, the DFI at m/z 151.0037 was observed at the retention time of peak 6, and the MS1 spectra of this peak is shown in Fig. 3, which indicated that the DFI was produced by the precursor ion of m/z 447. Also, the MS2 spectra of m/z 447 in the manual mode is presented in Fig. 4. In this spectrum, the most intense ion was m/z 301.0159, which was formed by the loss of m/z 146, and other fragment ions m/z 283, 271, 255, 243, and 179 were also produced from quercetin aglycones, which indicated that the compound of m/z 447 was quercetin-3-O-rhamnoside. The fragmentation pathways are proposed in Fig. 4. The fragment ions were verified by the literature data.22,23 The proposed fragmentation pathways of other peaks of type A flavonoids are shown in Fig. 5–9.
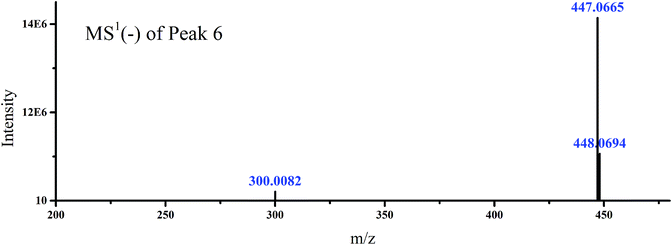 |
| Fig. 3 Accurate MS1 spectra of peak 6 in Fig. 1 selected from switchgrass. | |
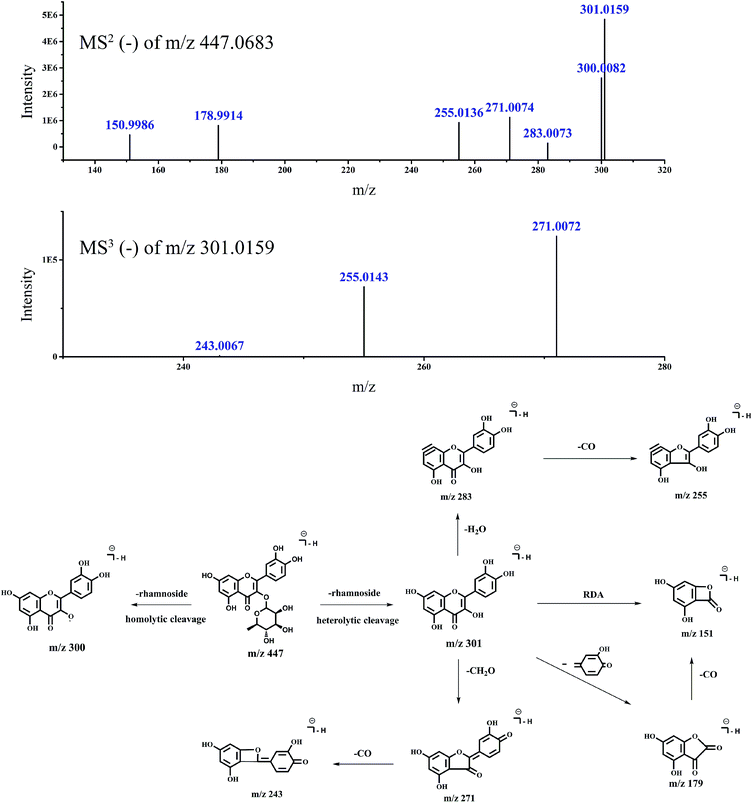 |
| Fig. 4 Accurate MSn spectra and proposed fragmentation pathways of peak 6 in Fig. 1 selected from switchgrass. | |
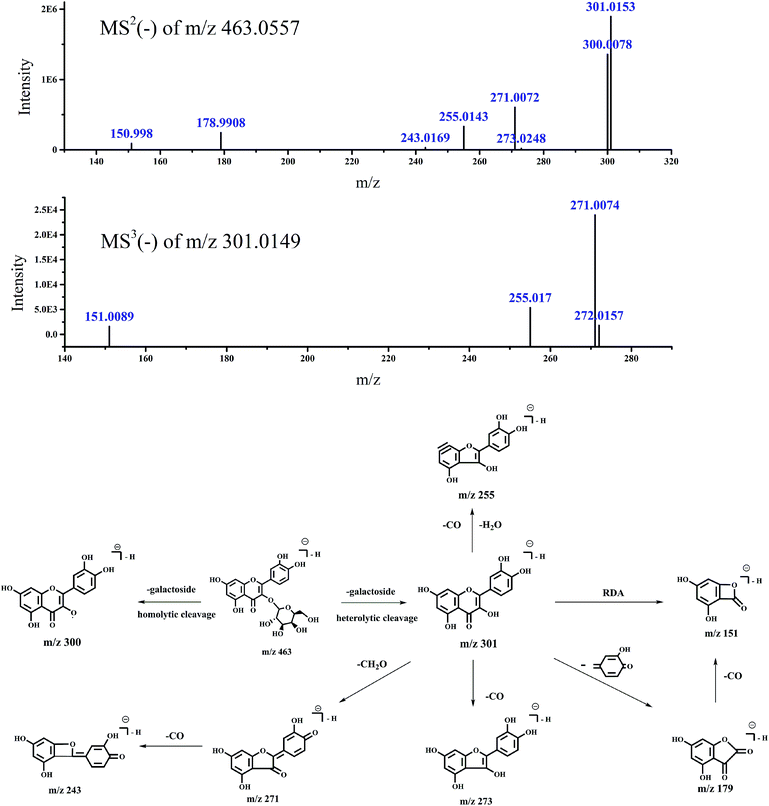 |
| Fig. 5 Accurate MSn spectra and proposed fragmentation pathways of peak 2 in Fig. 1 selected from switchgrass. | |
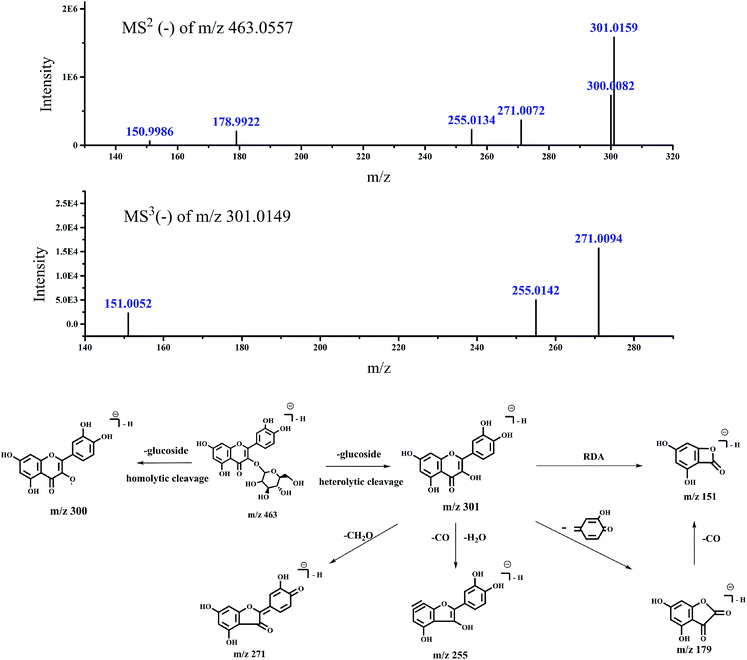 |
| Fig. 6 Accurate MSn spectra and proposed fragmentation pathways of peak 3 in Fig. 1 for m/z 463 selected from switchgrass. | |
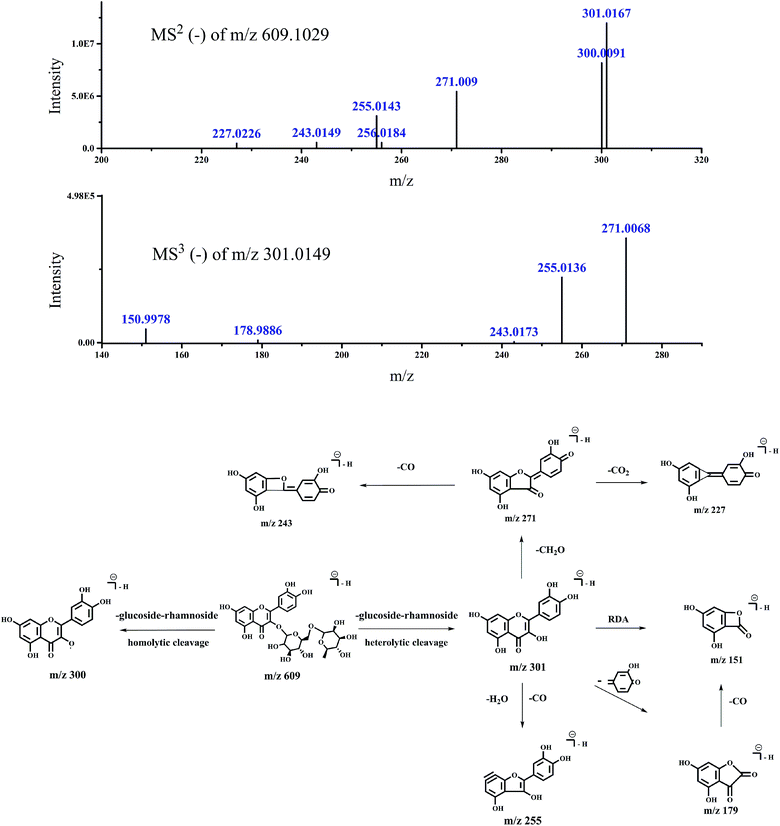 |
| Fig. 7 Accurate MSn spectra and proposed fragmentation pathways of peak 3 in Fig. 1 for m/z 609 selected from switchgrass. | |
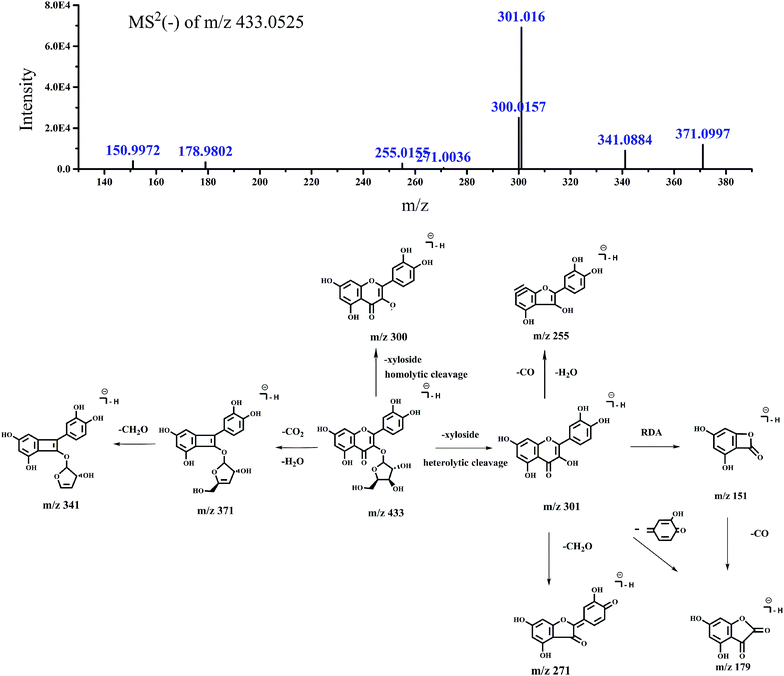 |
| Fig. 8 Accurate MSn spectra and proposed fragmentation pathways of peak 4 in Fig. 1 selected from switchgrass. | |
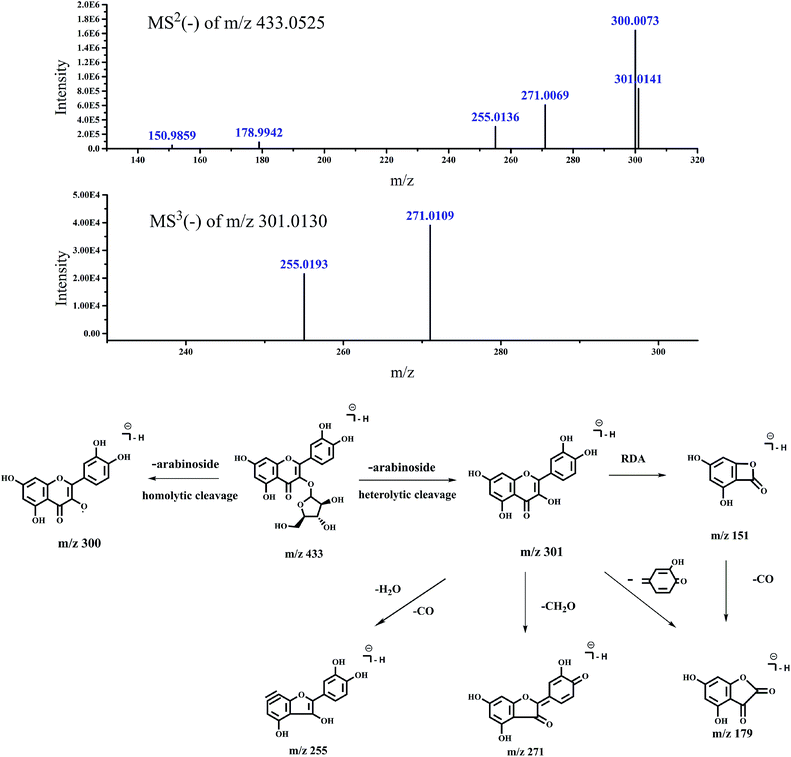 |
| Fig. 9 Accurate MSn spectra and proposed fragmentation pathways of peak 5 in Fig. 1 selected from switchgrass. | |
In addition, m/z 151.0035 was extracted in MS3 of peak 1 in Fig. 1. This fragment ion was produced from the compound of m/z 579 in the MS1 spectra. Though naringenin-7-O-rutinoside is a kind of flavonoid and has m/z 579 in the MS1 spectra, it could not produce the fragment ion of m/z 151.0037, and few radical fragment ions could be observed at 7-position glycosylation sites.18–20 It indicated that the peak 1 might not be addicted to type A flavonoids and the ion of m/z 151.0037 might be produced from other kind of compounds. After further analysis of the fragments in the manual mode of peak 1 (Table 2), the compound of m/z 579 in the MS1 spectra might be a kind of lignan (syringaresinol hexoside), which also consistent with previously reported observations.14,15 The proposed fragmentation pathways of peak 1 are shown in Fig. 10. There were also some ion fragments of flavonoids, which are the same as those of lignans, such as m/z 331, 165, and 179.14,15 The findings showed that the identification step by comparison of manual fragments played a crucial role in this strategy.
Table 2 Investigation of lignan in switchgrass
Invasive weeds |
Peak no. |
R
t (min) |
[M − H]−m/z |
ESI-MSnm/z (relative intensity %) |
Identification |
Ref. |
Switchgrass |
1 |
22.845 |
579.17 |
MS2 [579.1684]: 417.1304 (100), 418.1339 (9.17) |
Syringaresinol hexoside |
14 and 15
|
417.5202 (1.55), 402.1097 (1.01), 417.3155 (0.89) |
417.4426 (0.73), 371.1273 (0.19), 181.0396 (0.17) |
MS3 [417.1257]: 387.1001 (100) |
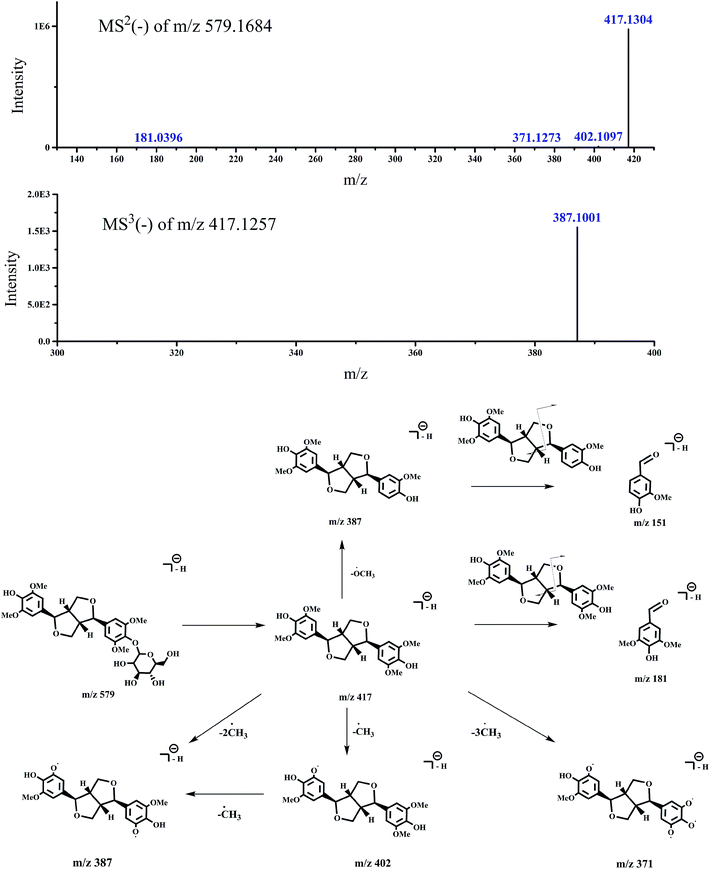 |
| Fig. 10 Accurate MSn spectra and proposed fragmentation pathways of peak 1 in Fig. 1 selected from switchgrass. | |
3.1.1. Detection and identification of three more type A and B flavonoids in M. micrantha.
This strategy was also applied for the detection of flavonoids in another invasive weed. Three more flavonoids of type A and B were detected (Fig. 11 and Table 3) in M. micrantha than in the previous report.17 It might be due to the optimization of LC conditions. The peaks did not fully separate on using a linear gradient from 35% to 67% B in 40 min.17 In this study, linear gradient elution was optimized from 35% to 40% B in 50 min. Under these new LC conditions, the peaks were separated more completely and more compounds were detected. The result indicated that LC conditions are also important in this strategy.
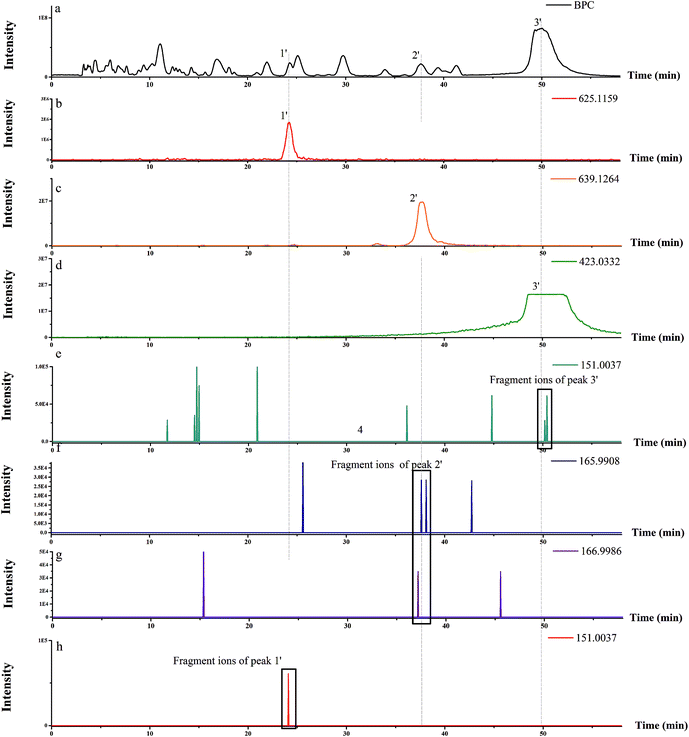 |
| Fig. 11 BPC of M. micrantha (a); EIC of the MS1 at m/z 625.1159, m/z 639.1264, and m/z 423.0332 (b, c, d, respectively); MSn fragment ion at m/z 151.0037, m/z 165.9908, and m/z 166.9986 of the M. micrantha (e, f, g, h, respectively). | |
Table 3 Investigation of type A and B flavonoids in M. micrantha
Invasive weeds |
Peak no. |
R
t (min) |
[M − H]−m/z |
ESI-MSnm/z (relative intensity %) |
Identification |
Ref. |
M. micrantha
|
1′ |
25.737 |
625.1159 |
MS2 [625.1159]: 301.0324 (100), 463.0819 (73.79) |
Quercetin-3-O-diglucoside |
27
|
300.0246 (15.59), 464.0855 (12.15), 302.0373 (6.48) |
271.0229 (3.47), 255.0266 (2.27), 301.3574 (1.03) |
301.1884 (0.92), 301.2835 (0.63), 343.0438 (0.4) |
463.2700 (0.3), 285.0381 (0.25) |
MS3 [463.08]: 301.033 (100), 300.0257 (36.77) |
302.0363 (14.56), 271.0219 (5.18), 179.0023 (4.78) |
255.0271 (3.46), 151.0059 (1.79), 300.9497 (0.64) |
2′ |
38.721 |
639.1304 |
MS2 [639.1304]: 315.0491 (100), 300.0245 (68.19) |
8-OMe kaempferol 3-O-sophoroside |
28
|
271.0227 (48.27), 323.0729 (43.78), 641.1323 (37.05) |
316.0513 (24.64), 301.0289 (22.57), 255.0272 (20.41) |
272.0295 (19.77), 221.0438 (12.54), 324.0778 (11.76) |
477.0981 (11.32) |
MS3 [315.0498]: 300.0247 (100), 271.0212 (42.77) |
272.0313 (36.22), 301.0270 (34.15), 255.0261 (22.87) |
254.0190 (7.55), 299.0182 (5.42), 165.9932 (5.41) |
256.0351 (3.17) |
3′ |
50 |
423.0463 |
MS2 [423.0392]: 343.0961 (100), 328.0611 (44.11) |
Quercetin 3′,4′,7-trimethyl ether-3-sulfate |
29
|
344.0882 (42.91), 285.0416 (15.86), 313.0374 (11.09) |
425.0407 (9.6), 343.3729 (9.43), 424.0423 (8.98) |
342.8593 (8.13) |
MS3 [343.0922]: 285.0420 (100), 328.0596 (98.56) |
313.0370 (89.43), 344.0874 (44.46), 329.0622 (27.61) |
270.0178 (13.77), 286.0436 (5.79), 327.9248 (5.1) |
314.0377 (4.92), 285.1978 (4.76) |
Using this strategy, flavonoids of type A and B were detected in these two invasive weeds. This developed approach could be widely used for studying flavonoid components in different invasive weeds.
3.1.2. Limitations of this approach and future prospects.
There is no denying that the proposed approach is highly efficient for the identification of non-target flavonoid components. In addition, compared with the identification of compounds using NMR and FTIR after isolation of each compound, the analytical time of this strategy is shorter and the procedure of structural identification is simplified. It may take one or two hours according to number of compounds to be analyzed. Nevertheless, to some extent, this method relies on LC/MS. LC/MS provides limited evidence regarding the identification of the structure and further identification is still required. Although this method improves the sensitivity of detection, the flavonoids in very low concentrations might still not be observed because of the limit of detection of the instrument. For example, for the peak 7 of switchgrass, the DFI of m/z 151.0037 could be extracted in the MS3 spectrum under the automatic mode, but it disappeared in the manual mode because of the ion accumulation of ion m/z 151.0037, which was cleaved from other precursor ions in auto mode. Even so, other fragmentation ions cleaved from this molecular ion peak suggest the pathway fragmentation, as was proved previously.24 Enhancing the detector voltage increases the signal intensity, but the voltage has a maximum value that cannot be exceeded. This method only investigated the flavonoids that produce the DFI of m/z 151.0037, 165.9908, and 166.9986. It could not extract flavonoids that produce other DFIs. In addition, these DFIs might be produced from other kind of compounds. For example, m/z 151.0037 was extracted in MS3 spectra of peak 1 in Fig. 1, but peak 1 was a kind of lignans (syringaresinol hexoside) (Table 2). Some ion fragments of flavonoids are the same as those of lignans, such as m/z 331, 165, and 179.14,15 In other words, the LC/MS-IT-TOF method can be used for the identification of structures in complex extracts, but might cause false-negative results for structures with the same ion fragments. Thus, there is a need to combine LC/MS-IT-TOF with other instruments in the future. Although the proposed strategy has limitations, it is still a valuable analytical strategy to provide rapid structural identification for natural components in a complex matrix without complicated pretreatment. In our study, several flavonoids were identified by this established method in invasive weeds, thus confirming the advantages of the proposed strategy. During the identification of flavonoids, the established method distinguishes the lignans from the flavonoids, but lignans producing the same ion fragments as flavonoids could be regarded as interference existing in the process of identification. This study provides a platform for researchers to modify the strategy gradually in the future and combine it with other separation techniques to obtain only the target compounds and identify their structure using NMR and FTIR in order to confirm the flavonoid type.
4. Conclusions
In our research, a promising strategy for fast and easy determination of flavonoid components was established and successfully applied for the identification of flavonoids in switchgrass and M. micrantha using LC/MS-IT-TOF. Using this strategy, 10 flavonoids were discovered from the two invasive weeds, including 7 flavonoids (quercetin-3-O-galactoside, quercetin-3-O-glucoside, rutin, quercetin-3-O-xyloside, quercetin-3-O-arabinoside, quercetin-3-O-rhamnoside, and isorhamnetin-3-O-rutinoside) in switchgrass and 3 flavonoids (quercetin-3-O-diglucoside, 8-OMe-kaempferol-3-O-sophoroside, and quercetin 3′,4′,7-trimethyl ether-3-sulfate) in M. micrantha, which have not been reported before. In addition, one lignan (syringaresinol hexoside) was detected. This strategy is quick, easy, and widely applicable for the determination of non-target flavonoids in invasive weeds. With the continuous and deep research, more DIFs will be found, which would expand the scope of this strategy and extend it to the identification of other non-target bioactive compounds from complex matrices.
Conflicts of interest
There are no conflicts of interest to declare.
Acknowledgements
Financial support from the National Key Research and Development Program of China (2017YFF0207800), the National Natural Science Foundation of China (NSFC, Grant No. 21075007), Program for New Century Excellent Talents in University (NCET-11-0563) and Beijing Nova Program Interdisciplinary Cooperation Project (Z161100004916045) is gratefully acknowledged.
References
- S. B. McLaughlin and L. Adams Kszos, Biomass Bioenergy, 2005, 28, 515 CrossRef.
- E. Alexopoulou, F. Zanetti, E. G. Papazoglou, M. Christou, Y. Papatheohari, K. Tsiotas and I. Papamichael, Ind. Crops Prod., 2017, 107, 446–452 CrossRef CAS.
- M. Essandoh, D. Wolgemuth, C. U. Pittman, D. Mohan and T. Mlsna, Chemosphere, 2017, 174, 49 CrossRef CAS PubMed.
- S. Zaibon, S. H. Anderson, A. L. Thompson, N. R. Kitchen, C. J. Gantzer and S. I. Haruna, Geoderma, 2017, 286, 46 CrossRef.
- Y. An, Y. Ma and J. Shui, Acta Agric. Scand., Sect. B, 2013, 63, 107 Search PubMed.
- Y. An, Y. Ma, J. Shui and W. Zhong, J. Plant Interact., 2015, 10, 142 CrossRef.
- Y. An, Y. Ma and J. Shui, Acta Agric. Scand., Sect. B, 2013, 63, 497 Search PubMed.
- J. Shui, Y. An, Y. Ma and N. Ichizen, Environ. Manage., 2010, 46, 590 CrossRef PubMed.
- E. M. Martin, S. L. Cousins, S. R. Talley, C. P. West, E. C. Clausen and D. J. Carrier, Trans. ASABE, 2011, 54, 1953 CrossRef CAS.
- N. Uppugundla, A. Engelberth, S. Vandhana Ravindranath, E. C. Clausen, J. O. Lay, J. Gidden and D. J. Carrier, J. Agric. Food Chem., 2009, 57, 7763 CrossRef CAS PubMed.
- Y. Li, M. Wang, A. Li, H. Zheng and Y. Wei, Anal. Methods, 2016, 8, 8179 RSC.
- M. Wang, Y. Lu, J. Liu, H. Li and Y. Wei, Biomed. Chromatogr., 2016, 30, 269 CrossRef CAS PubMed.
- C. de Bertoldi, M. De Leo, A. Braca and L. Ercoli, Chemoecology, 2009, 19, 169 CrossRef.
- P. C. Eklund, M. J. Backman, L. Å. Kronberg, A. I. Smeds and R. E. Sjöholm, J. Mass Spectrom., 2008, 43, 97 CrossRef CAS PubMed.
- C. B. Steingass, M. P. Glock, R. M. Schweiggert and R. Carle, Anal. Bioanal. Chem., 2015, 407, 6463 CrossRef CAS PubMed.
- C. Zheng, H. Hao, X. Wang, X. Wu, G. Wang, G. Sang, Y. Liang, L. Xie, C. Xia and X. Yao, J. Mass Spectrom., 2009, 44, 230 CrossRef CAS PubMed.
- M. Wang, A. Cao, C. Ouyang, Y. Li and Y. Wei, Anal. Methods, 2015, 7, 10207 RSC.
- A. Petsalo, J. Jalonen and A. Tolonen, J. Chromatogr. A, 2006, 1112, 224 CrossRef CAS PubMed.
- A. Lhuillier, N. Fabre, F. Moyano, N. Martins, C. Claparols, I. Fourasté and C. Moulis, J. Chromatogr. A, 2007, 1160, 13 CrossRef CAS PubMed.
- E. Hvattum and D. Ekeberg, J. Mass Spectrom., 2003, 38, 43–49 CrossRef CAS PubMed.
- E. Hvattum, Rapid Commun. Mass Spectrom., 2002, 16, 655 CrossRef CAS PubMed.
- M. A. Mohd Shukri and C. Alan, J. Trop. Agric. And Fd. Sci., 2010, 38, 221 Search PubMed.
- R. Tsao, R. Yang, J. C. Young and H. Zhu, J. Agric. Food Chem., 2003, 51, 6347 CrossRef CAS PubMed.
- L. Ding, X. Wang, S. Wang, J. Yu, Y. Qin, X. Zhang and F. Xie, J. Sep. Sci., 2015, 38, 4029 CrossRef CAS PubMed.
- A. S. Boath, D. Stewart and G. J. McDougall, Food Chem., 2012, 135, 929 CrossRef CAS PubMed.
- I. Parejo, O. Jauregui, F. Sánchez-Rabaneda, F. Viladomat, J. Bastida and C. Codina, J. Agric. Food Chem., 2004, 52, 3679 CrossRef CAS PubMed.
- P. Truchado, E. Tourn, L. M. Gallez, D. A. Moreno, F. Ferreres and F. A. Tomás-Barberán, J. Agric. Food Chem., 2010, 58, 12678 CrossRef CAS PubMed.
- F. Ferreres, R. Llorach and A. Gil-Izquierdo, J. Mass Spectrom., 2004, 39, 312 CrossRef CAS PubMed.
- P. Mann, B. Tofern, M. Kaloga and E. Eich, Phytochemistry, 1999, 50, 267 CrossRef CAS.
|
This journal is © The Royal Society of Chemistry 2018 |