DOI:
10.1039/C8QO01079J
(Research Article)
Org. Chem. Front., 2019,
6, 87-93
Photoinduced synthesis of α-trifluoromethylated ketones through the oxidative trifluoromethylation of styrenes using CF3SO2Na as a trifluoromethyl reagent without an external photoredox catalyst†
Received
8th October 2018
, Accepted 21st November 2018
First published on 22nd November 2018
Abstract
A photoinduced strategy to access α-CF3-substituted ketones through the reaction of simple styrenes with sodium trifluoromethanesulfinate (CF3SO2Na) in the absence of an external photosensitizer under LED (380–385 nm) irradiation and an air atmosphere at room temperature was developed. This reaction employs the commercially available, low cost, and easy to handle Langlois reagent (CF3SO2Na) as a CF3-radical source, and the reaction has advantages of mild conditions, a simple system and good functional group tolerance. Investigations indicated that the product acts as a photosensitizer and 1O2 coexists with O2˙− during the reaction through the energy transfer and single electron transfer process.
Introduction
The trifluoromethyl group (CF3), with electron-withdrawing nature, lipophilicity, and metabolic and chemical stability,1 is a very useful substituent in organics,2 and is prevalent in pharmaceuticals,3 materials,4 and agrochemicals.5 Hence, direct trifluoromethylation of organic compounds has received great attention.6 Particularly, the formation of α-CF3 substituted ketones is very attractive, because they can be used widely as versatile building blocks for the complex CF3-containing organic molecules. Until now, a number of trifluoromethylation reagents, such as CF3I,7 CF3SO2Cl,8 Togni's reagent,9 Langlois reagent,10 Umemoto's reagent,11 Ruppert–Prakash reagent,12 and S-(trifluoromethyl)diphenylsulfonium triflate ([Ph2SCF3]+OTf−),13 have been recommended as effective CF3-sources. Generally, the strategies on the synthesis of α-trifluoromethylated ketones14 depend on the electrophilic or radical trifluoromethylation of enolates, prepared from the corresponding aldehydes, ketones, esters and amides.15 However, the direct oxidative trifluoromethylation (keto-trifluoromethylation) of alkenes, which are the abundant and commonly used feedstocks, is a straightforward and concise route to obtain them. Some of the elegant examples are as follows. Maiti described an oxidative trifluoromethylation of unactivated olefins with CF3SO2Na as the CF3-source for the synthesis of α-CF3-substituted ketones catalyzed by AgNO3/K2S2O8 (Scheme 1a). In addition, Xiao developed an α-trifluoromethylation of styrenes with [Ph2SCF3]+OTf− in the presence of an excess amount of HOCH2SO2Na, providing α-CF3-substituted acetophenones (Scheme 1b). Recently, Cai reported an efficient method for the radical addition of olefins along with TMSCF3 to deliver various α-trifluoromethylated ketones (Scheme 1c). Most recently, the radical trifluoromethylation by photoredox catalysis has received great attention and emerged as a hot research topic because visible light as a rich and green energy source has been shown to induce a variety of organic transformations. MacMillan's group successfully developed a generation of α-CF3 ketones from silyl enol ethers by means of photoredox catalysis (Scheme 1d). Akita's group disclosed a photoredox catalytic system consisting of an iridium complex fac-[Ir(ppy)3] as an effective photoredox catalyst for the oxidative trifluoromethylation of styrenes with Togni's reagent under visible-light irradiation (Scheme 1e). Among these reactions, the involvement of a trifluoromethyl radical implied that the outcome of the reaction is significantly affected by means of generating the trifluoromethyl radical.
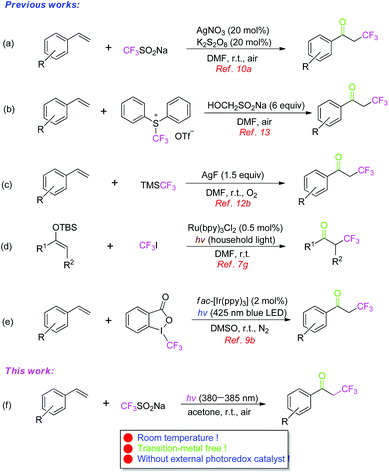 |
| Scheme 1 Synthesis of α-trifluoromethylated ketones from alkenes. | |
In general, photoredox catalysis16 as a powerful tool for organic synthesis needs a suitable photosensitizer (Ru-, Ir-, Au-complex, or organic dye). To avoid the above precious metals and expensive organic dyes used as photocatalysts, organic reactions under visible-light irradiation without the use of a photosensitizer are believed to be the direction of further research. Therefore, it is not surprising that intense research efforts have been made to develop an efficient protocol for the formation of α-CF3-substituted carbonyl compounds via photoredox catalysis without an additional photosensitizer.17 Herein, we wish to report an efficient photoinduced synthesis of α-trifluoromethylated ketones through the oxidative trifluoromethylation of styrenes with CF3SO2Na (Langlois reagent) as a commercially available, stable, and easy to handle CF3-source in the absence of an external photocatalyst under an air atmosphere, generating the corresponding products in good yields under transition-metal free and mild conditions (Scheme 1f).
At first, the reaction conditions were optimized using a model reaction of styrene (1a) with CF3SO2Na (2a), as shown in Table 1. When the model reaction was performed in the presence of Ru(bpy)3Cl2 as a photocatalyst under the irradiation of a blue LED (450–455 nm) and under an air atmosphere in acetone at room temperature for 18 h, the corresponding α-trifluoromethylated ketone 3a was provided in 24% yield (Table 1, entry 1). For the improvement of the desired product yield, a number of photocatalysts were examined. Among the tested photocatalysts, Ru(Phen)3Cl2, Mes-Acr-Me+ClO4−, fac-Ir(ppy)3, eosin Y, methylene blue and acridine red, generated 3a in 27–40% yields, and no reaction of Ru(Phen)3(PF6)2, rose bengal and fluorescein were observed (Table 1, entries 2–10). To our delight, when the model reaction was carried out in the absence of any additional photocatalyst and under LED 380–385 nm irradiation in acetone, 76% yield of 3a was obtained (Table 1, entry 11). Other solvents, such as MeCN, DME, DMSO and DMF exhibited lower reactivity, generating 3a in 17–64% yields; DCE and THF showed no reactivity in the reaction (Table 1, entries 12–17). Subsequently, the wavelength of the light source was investigated and the LED (380–385 nm) was the best one for the reaction. An optical wavelength less than 380–385 nm or more than 380–385 nm exhibited lower reactivity (Table 1, entries 18–20). It should be noted that a wavelength greater 450 nm did not show any reactivity (Table 1, entries 20 and 21). When the model reaction was performed in the absence of light irradiation (in the dark) or under a nitrogen atmosphere, no desired product 3a was observed (Table 1, entries 23 and 24). Further exploration of the reaction time indicated that 18 h is an optimal choice (Table 1, entry 25).
Table 1 Optimization of the reaction conditionsa

|
Entry |
Catalyst |
Solvent |
Light source |
Yieldb (%) |
Reaction conditions: Styrene (1a, 0.20 mmol), CF3SO2Na (2a, 0.40 mmol), solvent (2.0 mL) at room temperature under light irradiation (1.5 W) in air for 18 h.
Isolated yield.
N2 atmosphere.
For 15 h.
For 24 h. NR = no reaction.
|
1 |
Ru(bpy)3Cl2 |
Acetone |
450–455 nm |
24 |
2 |
Ru(Phen)3Cl2 |
Acetone |
450–455 nm |
27 |
3 |
Mes-Acr-Me+ClO4− |
Acetone |
450–455 nm |
40 |
4 |
fac-Ir(ppy)3 |
Acetone |
450–455 nm |
30 |
5 |
Eosin Y |
Acetone |
520–525 nm |
21 |
6 |
Methylene blue |
Acetone |
520–525 nm |
32 |
7 |
Acridine red |
Acetone |
520–525 nm |
36 |
8 |
Ru(Phen)3(PF6)2 |
Acetone |
450–455 nm |
Trace |
9 |
Rose bengal |
Acetone |
520–525 nm |
NR |
10 |
Fluorescein |
Acetone |
520–525 nm |
Trace |
11
|
—
|
Acetone
|
380–385 nm
|
76
|
12 |
—
|
CH3CN |
380–385 nm |
64 |
13 |
—
|
DME |
380–385 nm |
55 |
14 |
—
|
DMSO |
380–385 nm |
28 |
15 |
—
|
DMF |
380–385 nm |
17 |
16 |
—
|
DCE |
380–385 nm |
NR |
17 |
—
|
THF |
380–385 nm |
Trace |
18 |
—
|
Acetone |
365–370 nm |
54 |
19 |
—
|
Acetone |
410–415 nm |
38 |
20 |
—
|
Acetone |
420–425 nm |
24 |
21 |
—
|
Acetone |
450–455 nm |
Trace |
22 |
—
|
Acetone |
520–525 nm |
NR |
23 |
—
|
Acetone |
380–385 nm |
NRc |
24 |
—
|
Acetone |
Dark |
NR |
25 |
—
|
Acetone |
380–385 nm |
76d, 74e |
Based on the optimized conditions in our hand, the scope of oxidative trifluoromethylation of styrenes with CF3SO2Na was explored. First, a variety of styrene derivatives were examined under the standard conditions, indicating a broad tolerance of substituted groups on the aromatic rings. As shown in Scheme 2, styrenes with an electron-donating group (such as Me, t-Bu, or MeO) at the para-position of the benzene rings reacted with CF3SO2Na to afford the corresponding products 3b–3d in 58–65% yields. On the other hand, styrenes that possessed an electron-withdrawing group, including F, Cl, Br, F3C, Ph, CN and MeO2C on the para-position of the phenyl rings, underwent oxidative trifluoromethylation to generate the desired products 3e–3k in 56–85% yields. Besides, the yield of 3h could increase from 56% to 85% under LED 365–370 nm irradiation for 24 h. Obviously, the reactivity of styrene with electron-withdrawing groups is higher than that with electron-donating substituents in their oxidative trifluoromethylation with CF3SO2Na. Moreover, styrenes with a halogen group (F, Cl or Br) on the meta-position of benzene rings also delivered the anticipated products 3l–3n in 72–77% yields, while the substrate styrene substituted by a Me or F group on the ortho-position gave the ketones (3o–3p) in lower yields owing to the steric effect. 3,5-Dimethylstyrene reacted with CF3SO2Na under standard reaction conditions to generate the corresponding product 3q in 53% yield. In addition, the reaction of 2-vinylnaphthalene with CF3SO2Na gave the corresponding product (3r) in 57% yield. Only 45% yield of 3s was obtained when 2-vinylthiophene was employed as a starting material. It should be noted that (E)-prop-1-en-1-ylbenzene worked well with CF3SO2Na to achieve the desired product (3t) in 51% yield. When normal alkenes, such as cyclopentene, allylbenzene, cyclooctene and benzyl vinyl ether were used as the substrates in the reactions with CF3SO2Na under the optimized conditions, unfortunately, no corresponding products were obtained.
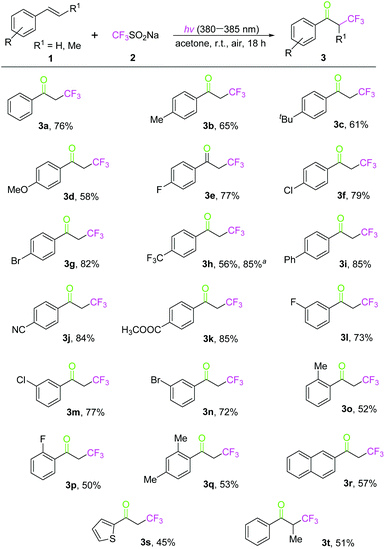 |
| Scheme 2 The scope of styrenes [reaction conditions: Styrene (1, 0.20 mmol), CF3SO2Na (2a, 0.40 mmol), acetone (2.0 mL), 1.5 W LED (380–385 nm), air, r.t., 18 h; isolated yield of the product; a under the irradiation of LED 365–370 nm for 24 h]. | |
Under the standard reaction conditions, we tried to explore the reactivity of cyclic olefins. As shown in Scheme 3, 1,2-dihydro-naphthalene and 1,2-dihydro-indene were transformed into the corresponding cyclic trifluoromethylation ketones (3u and 3v) in moderate yields.
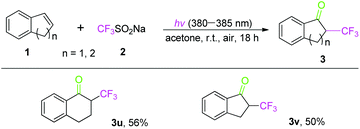 |
| Scheme 3 Substrate scope of cyclic olefins [reaction conditions: Cyclic olefin (1, 0.20 mmol), CF3SO2Na (2a, 0.40 mmol), acetone (2.0 mL), 1.5 W LED (380–385 nm), air, r.t., 18 h; isolated yield of the product]. | |
To further clarify the mechanism of this transformation, several control experiments were conducted, as shown in Scheme 4. When the model reaction was carried out in the presence of a radical scavenger 2,2,6,6-tetramethyl-1-piperidinyloxy (TEMPO, 1.5 equiv.) under the standard conditions, the oxidative trifluoromethylation was completely inhibited, suggesting that a radical process might be involved in the reaction. To trap the CF3 radical intermediate formed during the reaction, 1,1-diphenylethylene was added into the system, generating a radical coupling product 5 in 35% isolated yield.18 Additionally, in order to understand the role of oxygen in the reaction, the electron paramagnetic resonances (EPR) were recorded using 2,2,6,6-tetramethylpiperidine (TEMP) and 5,5-dimethyl-pyrroline-N-oxide (DMPO) to capture 1O2 and O2˙−, respectively, and the resulting EPR spectra are presented in Fig. S1 and S2 of the ESI.† There was no signal when DMPO was added into a solution of 1a and 2a in air-saturated acetone without light irradiation (Fig. S1a†). But, a strong characteristic signal of an O2˙− adduct with DMPO was observed when the above solution was irradiated with LED 380–385 nm (Fig. S1b†). Meanwhile, a strong signal of the 1O2 adduct with TEMP was found when the above solution was irradiated with a LED (380–385 nm), but no signal was recorded without light (Fig. S2†), implying that 1O2 coexists with O2˙− in the reaction. Moreover, ultraviolet-visible absorption spectra of the corresponding products 3 indicated that 3 can absorb light at 380–385 nm and act as photo-sensitizers (Fig. S3–S6†), avoiding the external photocatalyst. It should be noted that the α-trifluoromethylated ketone as a product could absorb 380–385 nm light, which activates O2 in air efficiently and accelerates the reaction, so the entire oxidative trifluoromethylation is self-sustaining in an autocatalytic manner (Fig. S7†). It is believed that singlet oxygen 1O2 may be generated from the interaction of O2 in air with a photoexcited product via an energy-transfer process.
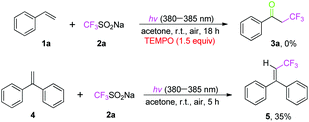 |
| Scheme 4 The control experiments. | |
On the basis of the above experimental results and relevant literature, a plausible mechanism is proposed as shown in Scheme 5. Firstly, product (P) is excited by visible light irradiation (380–385 nm) to generate the excited species (P*), which then undergoes an energy transfer process with ground-state triplet oxygen 3O2 to afford an excited-state singlet oxygen 1O2 with the regeneration of ground state P. The obtained singlet 1O2 reacts with CF3SO2Na (2a) via a single electron transfer (SET) process to generate O2˙− and a CF3SO2˙ radical, which releases SO2 and a CF3˙ radical. The generated CF3˙ reacts with substrate 1a to generate an intermediate A, which undergoes a reaction with the O2 radical anion (O2˙−) to afford the hydroperoxide intermediate B. This intermediate B then loses H2O and gives the desired product 3a. On the other hand, the intermediate B may also be transformed into the benzyl alcohol derivative C, which undergoes further oxidation to ketone 3a.19
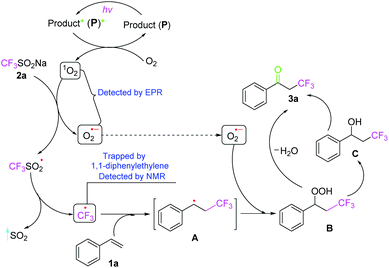 |
| Scheme 5 The proposed mechanism. | |
Conclusions
In conclusion, we have presented a novel, simple, and general strategy to access α-CF3-substituted ketones by the reaction of simple styrenes with sodium trifluoromethanesulfinate (CF3SO2Na, Langlois reagent) in the absence of an external photosensitizer under photo-irradiation (380–385 nm) and an air atmosphere at room temperature. This reaction employs the commercially available, low cost, and easy to handle Langlois reagent that serves as a CF3 radical source, and has advantages of mild conditions and good functional-group tolerance. Its detailed reaction mechanism is being studied in our laboratory.
Experimental section
General remarks
The 1H NMR, 13C NMR and 19F NMR spectra were recorded on a 400 MHz Bruker FT-NMR spectrometer (400 MHz, 100 MHz or 376 MHz, respectively). All chemical shifts are given as δ value (ppm) with reference to tetramethylsilane (TMS) as an internal standard. The peak patterns are indicated as follows: s, singlet; d, doublet; t, triplet; m, multiplet; q, quartet. The coupling constants, J, are reported in hertz (Hz). High resolution mass spectroscopy data of the product were collected on an Agilent Technologies 6540 UHD Accurate-Mass Q-TOF LC/MS (ESI). All the solvents and commercially available reagents were purchased from commercial suppliers. Products were purified by flash chromatography on 200–300 mesh silica gels, SiO2.
Typical procedure for α-trifluoromethylation of styrenes with CF3SO2Na
A 5 mL oven-dried reaction vessel equipped with a magnetic stirrer bar was charged with styrene (1a, 0.20 mmol), sodium trifluoromethanesulfinate (2a, 0.40 mmol) and acetone (2.0 mL). The reaction vessel was exposed to LED (380–385 nm, 1.5 W) irradiation at room temperature in air with stirring for 18 h. After completion of the reaction, the mixture was concentrated to yield the crude product, which was further purified by flash chromatography (silica gel, petroleum ether/ethyl acetate = 20
:
1 to 50
:
1) to give the desired product 3a.
Characterization data for all products
3,3,3-Trifluoro-1-phenylpropan-1-one (3a)10a.
White solid. 1H NMR (400 MHz, CDCl3) δ: 7.94–7.92 (m, 2H), 7.66–7.62 (m, 1H), 7.53–7.49 (m, 2H), 3.80 (q, J = 10.0 Hz, 2H). 13C NMR (100 MHz, CDCl3) δ: 189.7, 135.8, 134.2, 128.9, 128.3, 124.0 (q, J = 275.3 Hz), 42.1 (q, J = 28.1 Hz). 19F NMR (376 MHz, CDCl3) δ: −62.02.
3,3,3-Trifluoro-1-(p-tolyl)propan-1-one (3b)10a.
White solid. 1H NMR (400 MHz, CDCl3) δ: 7.82 (d, J = 8.0 Hz, 2H), 7.29 (d, J = 8.0 Hz, 2H), 3.77 (q, J = 10.0 Hz, 2H), 2.42 (s, 3H). 13C NMR (100 MHz, CDCl3) δ: 189.3 (d, J = 2.4 Hz), 145.3, 133.3, 129.5, 128.4, 124.1 (q, J = 275.2 Hz), 41.8 (q, J = 27.9 Hz), 21.6. 19F NMR (376 MHz, CDCl3) δ: −62.06.
1-(4-(tert-Butyl)phenyl)-3,3,3-trifluoropropan-1-one (3c)14a.
White solid. 1H NMR (400 MHz, CDCl3) δ: 7.88 (d, J = 8.4 Hz, 2H), 7.51 (d, J = 8.4 Hz, 2H), 3.78 (q, J = 10.0 Hz, 2H), 1.34 (s, 9H). 13C NMR (100 MHz, CDCl3) δ: 189.3 (d, J = 2.2 Hz), 158.1, 133.3, 128.6, 125.8, 124.1 (q, J = 275.9 Hz), 41.8 (q, J = 27.9 Hz), 35.1. 19F NMR (376 MHz, CDCl3) δ: −62.00.
3,3,3-Trifluoro-1-(4-methoxyphenyl)propan-1-one (3d)10a.
White solid. 1H NMR (400 MHz, CDCl3) δ: 7.91 (d, J = 9.2 Hz, 2H), 6.96 (d, J = 8.8 Hz, 2H), 3.88 (s, 3H), 3.75 (q, J = 10.0 Hz, 2H). 13C NMR (100 MHz, CDCl3) δ: 188.1 (d, J = 2.5 Hz), 164.3, 130.7, 128.9, 124.1 (q, J = 275.3 Hz), 114.0, 55.5, 41.7 (q, J = 27.8 Hz). 19F NMR (376 MHz, CDCl3) δ: −61.98.
3,3,3-Trifluoro-1-(4-fluorophenyl)propan-1-one (3e)10a.
Colorless liquid. 1H NMR (400 MHz, CDCl3) δ: 7.99–7.96 (m, 2H), 7.20–7.16 (m, 2H), 3.79 (q, J = 10.0 Hz, 2H). 13C NMR (100 MHz, CDCl3) δ: 188.2, 166.3 (d, J = 255.3 Hz), 132.3, 131.1 (d, J = 9.6 Hz), 123.9 (d, J = 275.3 Hz), 116.1 (d, J = 22.0 Hz), 42.0 (q, J = 28.2 Hz). 19F NMR (376 MHz, CDCl3) δ: −62.06, −102.98.
1-(4-Chlorophenyl)-3,3,3-trifluoropropan-1-one (3f)10a.
White solid. 1H NMR (400 MHz, CDCl3) δ: 7.88 (d, J = 8.4 Hz, 2H), 7.49 (d, J = 8.4 Hz, 2H), 3.77 (q, J = 10.0 Hz, 2H). 13C NMR (100 MHz, CDCl3) δ: 188.5, 140.9, 134.2, 129.8, 129.3, 123.8 (q, J = 275.5 Hz), 42.2 (q, J = 28.2 Hz). 19F NMR (376 MHz, CDCl3) δ: −61.98.
1-(4-Bromophenyl)-3,3,3-trifluoropropan-1-one (3g)10a.
White solid. 1H NMR (400 MHz, CDCl3) δ: 8.05 (d, J = 8.8 Hz, 2H), 7.84 (d, J = 8.4 Hz, 2H), 3.84 (q, J = 10.0 Hz, 2H). 13C NMR (100 MHz, CDCl3) δ: 188.8, 134.5, 132.3, 129.8, 129.6, 123.8 (q, J = 275.4 Hz), 42.1 (q, J = 28.3 Hz). 19F NMR (376 MHz, CDCl3) δ: −62.00.
3,3,3-Trifluoro-1-(4-(trifluoromethyl)phenyl)propan-1-one (3h)15a.
White solid. 1H NMR (400 MHz, CDCl3) δ: 8.05 (d, J = 8.0 Hz), 7.78 (d, J = 8.4 Hz), 3.85 (q, J = 10.0 Hz, 2H). 13C NMR (100 MHz, CDCl3) δ: 188.9, 138.3, 135.4 (q, J = 32.7 Hz), 128.7, 126.0 (q, J = 3.7 Hz), 123.7 (q, J = 275.4 Hz), 123.4 (q, J = 271.1 Hz), 42.4 (q, J = 28.5 Hz). 19F NMR (376 MHz, CDCl3) δ: −62.02, −63.35.
1-((1,1′-Biphenyl)-4-yl)-3,3,3-trifluoropropan-1-one (3i)14a.
White solid. 1H NMR (400 MHz, CDCl3) δ: 7.98 (d, J = 8.8 Hz, 2H), 7.69 (d, J = 8.4 Hz, 2H), 7.63–7.60 (m, 2H), 7.49–7.45 (m, 2H), 7.43–7.39 (m, 1H), 3.81 (q, J = 10.0 Hz, 2H). 13C NMR (100 MHz, CDCl3) δ: 189.2 (q, J = 2.5 Hz), 146.8, 139.4, 134.5, 129.0, 128.9, 128.5, 127.5, 127.2, 124.0 (q, J = 275.3 Hz), 42.1 (q, J = 28.1 Hz). 19F NMR (376 MHz, CDCl3) δ: −61.92.
4-(3,3,3-Trifluoropropanoyl)benzonitrile (3j)10a.
White solid. 1H NMR (400 MHz, CDCl3) δ: 8.05 (d, J = 8.4 Hz, 2H), 7.84 (d, J = 8.4 Hz, 2H), 3.84 (q, J = 10.0 Hz, 2H). 13C NMR (100 MHz, CDCl3) δ: 188.5, 138.5, 132.8, 128.7, 123.6 (d, J = 275.6 Hz), 117.5, 42.4 (q, J = 28.7 Hz). 19F NMR (376 MHz, CDCl3) δ: −61.97.
Methyl-4-(3,3,3-trifluoropropanoyl)benzoate (3k)10a.
White solid. 1H NMR (400 MHz, CDCl3) δ: 8.15–8.13 (m, 2H), 8.00–7.98 (m, 2H), 3.96 (s, 3H), 3.88 (q, J = 10.0 Hz, 2H). 13C NMR (100 MHz, CDCl3) δ: 189.3 (d, J = 2.2 Hz), 165.8, 138.7, 134.7, 129.9, 128.1, 123.8 (q, J = 275.2 Hz), 52.4, 42.3 (q, J = 28.3 Hz). 19F NMR (376 MHz, CDCl3) δ: −62.13.
3,3,3-Trifluoro-1-(3-fluorophenyl)propan-1-one (3l).
Colorless liquid. 1H NMR (400 MHz, CDCl3) δ: 7.72–7.70 (m, 1H), 7.65–7.62 (m, 1H), 7.54–7.48 (m, 1H), 7.37–7.32 (m, 1H), 3.79 (q, J = 10.0 Hz, 2H). 13C NMR (100 MHz, CDCl3) δ: 188.5, 162.9 (d, J = 247.5 Hz), 137.8, 130.7 (d, J = 7.5 Hz), 124.1 (d, J = 3.1 Hz), 123.8 (q, J = 275.3 Hz), 121.3 (d, J = 21.5 Hz), 115.1 (d, J = 22.6 Hz), 42.3 (q, J = 28.5 Hz). 19F NMR (376 MHz, CDCl3) δ: −62.13, −110.95. HRMS (EI): calcd for C9H6F4O: 206.0355, found: 206.0357.
1-(3-Chlorophenyl)-3,3,3-trifluoropropan-1-one (3m)10d.
Colorless liquid. 1H NMR (400 MHz, CDCl3) δ: 7.91–7.90 (m, 1H), 7.82–7.79 (m, 1H), 7.62–7.59 (m, 1H), 7.48–7.44 (m, 1H), 3.79 (q, J = 10.0 Hz, 2H). 13C NMR (100 MHz, CDCl3) δ: 188.5 (q, J = 2.3 Hz), 137.2, 135.4, 134.1, 130.3, 128.4, 126.4, 123.8 (q, J = 275.4 Hz), 42.2 (q, J = 28.3 Hz). 19F NMR (376 MHz, CDCl3) δ: −62.05.
1-(3-Bromophenyl)-3,3,3-trifluoropropan-1-one (3n)10d.
Yellow liquid. 1H NMR (400 MHz, CDCl3) δ: 8.05 (s, 1H), 7.84 (d, J = 8.0 Hz, 1H), 7.75 (d, J = 8.0 Hz, 1H), 7.42–7.38 (m, 1H), 3.78 (q, J = 10.0 Hz, 2H). 13C NMR (100 MHz, CDCl3) δ: 188.4, 137.4, 137.0, 131.3, 130.5, 126.8, 123.7 (q, J = 275.4 Hz), 123.3, 42.1 (q, J = 28.4 Hz). 19F NMR (376 MHz, CDCl3) δ: −62.02.
3,3,3-Trifluoro-1-(o-tolyl)propan-1-one (3o)10a.
Colorless liquid. 1H NMR (400 MHz, CDCl3) δ: 7.60–7.58 (m, 1H), 7.44–7.40 (m, 1H), 7.30–7.27 (m, 2H), 3.73 (q, J = 10.0 Hz, 2H), 2.53 (s, 1H). 13C NMR (100 MHz, CDCl3) δ: 192.7 (q, J = 2.3 Hz), 139.5, 135.9, 132.5, 132.4, 128.9, 124.0 (q, J = 275.3 Hz), 44.5 (q, J = 27.6 Hz), 21.4. 19F NMR (376 MHz, CDCl3) δ: −62.09.
3,3,3-Trifluoro-1-(2-fluorophenyl)propan-1-one (3p)14a.
White solid. 1H NMR (400 MHz, CDCl3) δ: 7.94–7.90 (m, 1H), 7.63–7.57 (m, 1H), 7.30–7.26 (m, 1H), 7.20–7.15 (m, 1H), 3.87–3.79 (m, 2H). 13C NMR (100 MHz, CDCl3) δ: 187.6–187.5 (m), 162.0 (d, J = 252.7 Hz), 135.9 (d, J = 9.2 Hz), 130.8 (d, J = 1.9 Hz), 124.9–124.8 (m), 127.9–119.7 (m), 116.8 (d, J = 23.6 Hz), 43.0–46.0 (m). 19F NMR (376 MHz, CDCl3) δ: −62.66, −109.80.
1-(2,4-Dimethylphenyl)-3,3,3-trifluoropropan-1-one (3q).
Colorless liquid. 1H NMR (400 MHz, CDCl3) δ: 7.55–7.53 (m, 1H), 7.11–7.10 (m, 2H), 3.73 (q, J = 10.0 Hz, 2H), 2.53 (s, 3H), 2.37 (s, 3H). 13C NMR (100 MHz, CDCl3) δ: 191.9 (q, J = 2.2 Hz), 143.5, 140.1, 133.4, 133.0, 129.5, 126.5, 124.1 (q, J = 275.3 Hz), 44.1 (q, J = 27.4 Hz), 21.7, 21.4. 19F NMR (376 MHz, CDCl3) δ: −62.06. HRMS (EI): calcd for C11H11F3O: 216.0762, found: 216.0768.
3,3,3-Trifluoro-1-(naphthalen-2-yl)propan-1-one (3r)10a.
White solid. 1H NMR (400 MHz, CDCl3) δ: 8.41 (s, 1H), 8.01–7.96 (m, 2H), 7.92–7.88 (m, 2H), 7.66–7.57 (m, 2H), 3.93 (q, J = 10.0 Hz, 2H). 13C NMR (100 MHz, CDCl3) δ: 189.6, 136.0, 133.2, 132.3, 130.5, 129.7, 129.2, 128.9, 127.9, 127.2, 124.1 (q, J = 275.2 Hz), 123.4, 42.2 (q, J = 28.0 Hz). 19F NMR (376 MHz, CDCl3) δ: −61.90.
3,3,3-Trifluoro-1-(thiophen-2-yl)propan-1-one (3s)10d.
Colorless liquid. 1H NMR (400 MHz, CDCl3) δ: 7.77–7.73 (m, 2H), 7.20–7.18 (m, 1H), 3.72 (q, J = 10.0 Hz, 2H). 13C NMR (100 MHz, CDCl3) δ: 143.2, 135.7, 133.4, 128.5, 123.6 (q, J = 275.6 Hz), 43.0 (q, J = 28.6 Hz). 19F NMR (376 MHz, CDCl3) δ: −61.93.
3,3,3-Trifluoro-2-methyl-1-phenylpropan-1-one (3t)10a.
Colorless liquid. 1H NMR (400 MHz, CDCl3) δ: 7.97–7.95 (m, 2H), 7.65–7.62 (m, 1H), 7.53–7.49 (m, 2H), 4.31–4.20 (m, 1H), 1.48 (d, J = 7.2 Hz, 3H). 13C NMR (100 MHz, CDCl3) δ: 194.4, 135.6, 134.0, 129.0, 128.6, 125.3 (q, J = 278.3 Hz), 44.3 (q, J = 26.4 Hz), 11.7–11.6 (m). 19F NMR (376 MHz, CDCl3) δ: −68.29.
2-(Trifluoromethyl)-3,4-dihydronaphthalen-1(2H)-one (3u)10a.
White solid. 1H NMR (400 MHz, CDCl3) δ: 8.05–8.03 (m, 1H), 7.35–7.31 (m, 1H), 7.28–7.26 (m, 1H), 3.30–3.07 (m, 3H), 2.53–2.20 (m, 2H). 13C NMR (100 MHz, CDCl3) δ: 190.2, 143.1, 134.1, 131.9, 128.7, 127.8, 127.0, 125.0 (q, J = 278.1 Hz), 50.8 (q, J = 25.4 Hz), 27.5, 23.4–23.3 (m). 19F NMR (376 MHz, CDCl3) δ: −67.53.
2-(Trifluoromethyl)-2,3-dihydro-1H-inden-1-one (3v)10a.
White solid. 1H NMR (400 MHz, CDCl3) δ: 7.82–7.80 (m, 1H), 7.69–7.65 (m, 1H), 7.54–7.52 (m, 1H), 7.46–7.42 (m, 1H), 3.50–3.30 (m, 3H). 13C NMR (100 MHz, CDCl3) δ: 196.8, 152.0, 135.8, 129.1, 128.2, 126.5, 124.8 (q, J = 276.8 Hz), 124.7, 49.7 (q, J = 27.4 Hz), 27.6 (d, J = 2.3 Hz). 19F NMR (376 MHz, CDCl3) δ: −67.76.
(3,3,3-Trifluoroprop-1-ene-1,1-diyl)dibenzene (5)17.
White solid. 1H NMR (400 MHz, CDCl3) δ: 7.38–7.37 (m, 3H), 7.35–7.29 (m, 3H), 7.25–7.23 (m, 4H), 6.12 (q, J = 8.5 Hz, 1H). 13C NMR (100 MHz, CDCl3) δ: 152.5 (q, J = 5.6 Hz), 140.1, 137.3, 129.4, 129.1 (q, J = 1.7 Hz), 128.5, 128.0, 127.9, 123.1 (q, J = 270.0 Hz), 115.5 (q, J = 33.6 Hz). 19F NMR (376 MHz, CDCl3) δ: −55.59.
Conflicts of interest
There are no conflicts to declare.
Acknowledgements
We gratefully acknowledge the National Natural Science Foundation of China (21772062 and 21572078) for financial support of this work.
Notes and references
-
(a) C. Isanbora and D. O'Hagan, J. Fluorine Chem., 2006, 127, 303 CrossRef;
(b) K. L. Kirk, Org. Process Res. Dev., 2008, 12, 305 CrossRef CAS;
(c) S. Purser, P. R. Moore, S. Swallow and V. Gouverneur, Chem. Soc. Rev., 2008, 37, 320 RSC;
(d) W. K. Hagmann, J. Med. Chem., 2008, 51, 4359 CrossRef CAS;
(e) K. L. Kirk, Org. Process Res. Dev., 2008, 12, 305 CrossRef CAS;
(f) O. A. Tomashenko and V. V. Grushin, Chem. Rev., 2011, 111, 4475 CrossRef CAS;
(g) T. Furuya, A. S. Kamlet and T. Ritter, Nature, 2011, 473, 470 CrossRef CAS;
(h) P. S. Fier and J. F. Hartwig, J. Am. Chem. Soc., 2012, 134, 5524 CrossRef CAS;
(i) E. P. Gillis, K. J. Eastman, M. D. Hill, D. J. Donnelly and N. A. Meanwell, J. Med. Chem., 2015, 58, 8315 CrossRef CAS.
-
(a) N. A. Meanwell, J. Med. Chem., 2011, 54, 2529 CrossRef CAS;
(b) M. Cametti, B. Crousse, P. Metrangolo, R. Milani and G. Resnati, Chem. Soc. Rev., 2012, 41, 31 RSC;
(c) Y. Zhou, J. Wang, Z. Gu, S. Wang, W. Zhu, J. L. Aceña, V. A. Soloshonok, K. Izawa and H. Liu, Chem. Rev., 2016, 116, 422 CrossRef CAS.
-
(a)
R. Filler and L. M. Yagupolskii, Organofluorine Compounds in Medicinal Chemistry & Biomedical Applications, Elsevier, New York, 1993 Search PubMed;
(b) K. Müller, C. Faeh and F. Diederich, Science, 2007, 317, 1881 CrossRef;
(c) J. Wang, M. Sanchez-Roselló, J. L. Aceña, C. Pozo, A. E. Sorochinsky, S. Fustero, V. A. Soloshonok and H. Liu, Chem. Rev., 2014, 114, 2432 CrossRef CAS PubMed.
-
T. Hiyama, Organofluorine Compounds: Chemistry and Applications, Springer, Berlin, 2000 Search PubMed.
-
R. E. Banks and C. J. Tatlow, Organofluorine Chemistry, Principles and Commercial Applications, Springer, New York, 1994 Search PubMed.
-
(a) H. Song, Q. Han, C. Zhao and C. Zhang, Green Chem., 2018, 20, 1662 RSC;
(b) W. Li, W. Xu, J. Xie, S. Yu and C. Zhu, Chem. Soc. Rev., 2018, 47, 654 RSC.
-
(a) Y. Itoh and K. Mikami, Org. Lett., 2005, 7, 4883 CrossRef CAS;
(b) Y. Itoh and K. Mikami, Tetrahedron, 2006, 62, 7199 CrossRef CAS;
(c) Y. Itoh and K. Mikami, J. Fluorine Chem., 2006, 127, 539 CrossRef CAS;
(d) V. Petrik and D. Cahard, Tetrahedron Lett., 2007, 48, 3327 CrossRef CAS;
(e) Y. Tomita, Y. Itoh and K. Mikami, Chem. Lett., 2008, 37, 1080 CrossRef CAS;
(f) T. Kino, Y. Nagase, Y. Ohtsuka, K. Yamamoto, D. Uraguchi, K. Tokuhisa and T. Yamakawa, J. Fluorine Chem., 2010, 131, 98 CrossRef CAS;
(g) P. V. Pham, D. A. Nagib and D. W. C. MacMillan, Angew. Chem., Int. Ed., 2011, 50, 6119 CrossRef CAS;
(h) N. Iqbal, S. Choi, E. Kim and E. J. Cho, J. Org. Chem., 2012, 77, 11383 CrossRef CAS;
(i) N. Iqbal, J. Jung, S. Park and E. J. Cho, Angew. Chem., Int. Ed., 2014, 53, 539 CrossRef CAS.
-
(a) D. A. Nagib and D. W. C. MacMillan, Nature, 2011, 480, 224 CrossRef CAS;
(b) H. Jiang, Y. Cheng, Y. Zhang and S. Yu, Eur. J. Org. Chem., 2013, 5485 CrossRef CAS;
(c) S. W. Oh, Y. R. Malpani, N. Ha, Y.-S. Jung and S. B. Han, Org. Lett., 2014, 16, 1310 CrossRef CAS;
(d) D. B. Bagal, G. Kachkovskyi, M. Knorn, T. Rawner, B. M. Bhanage and O. Reiser, Angew. Chem., Int. Ed., 2015, 54, 6999 CrossRef CAS.
-
(a) X. Liu, F. Xiong, X. Huang, L. Xu, P. Li and X. Wu, Angew. Chem., Int. Ed., 2013, 52, 6962 CrossRef CAS;
(b) R. Tomita, Y. Yasu, T. Koike and M. Akita, Angew. Chem., Int. Ed., 2014, 53, 7144 CrossRef CAS;
(c) L. Li, Q.-Y. Chen and Y. Guo, J. Org. Chem., 2014, 79, 5145 CrossRef CAS.
-
(a) A. Deb, S. Manna, A. Modak, T. Patra, S. Maity and D. Maiti, Angew. Chem., Int. Ed., 2013, 52, 9747 CrossRef CAS;
(b) A. Maji, A. Hazra and D. Maiti, Org. Lett., 2014, 16, 4524 CrossRef CAS PubMed;
(c) Q. Lu, C. Liu, Z. Huang, Y. Ma, J. Zhang and A. Lei, Chem. Commun., 2014, 50, 14101 RSC;
(d) Z. Hang, Z. Li and Z.-Q. Liu, Org. Lett., 2014, 16, 3648 CrossRef CAS;
(e) L. Zhang, Z. Li and Z.-Q. Liu, Org. Lett., 2014, 16, 3688 CrossRef CAS;
(f) Y. Yang, Y. Liu, Y. Jiang, Y. Zhang and D. A. Vicic, J. Org. Chem., 2015, 80, 6639 CrossRef CAS;
(g) X.-L. Yu, J.-R. Chen, D.-Z. Chen and W.-J. Xiao, Chem. Commun., 2016, 52, 8275 RSC;
(h) L. Zhu, L.-S. Wang, B. Li, B. Fu, C.-P. Zhang and W. Li, Chem. Commun., 2016, 52, 6371 RSC;
(i) L. Li, Xi. Mu, W. Liu, Y. Wang, Z. Mi and C.-J. Li, J. Am. Chem. Soc., 2016, 138, 5809 CrossRef CAS PubMed;
(j) H.-T. Qin, S.-W. Wu, J.-L. Liu and F. Liu, Chem. Commun., 2017, 53, 1696 RSC.
-
(a) Y. Yasu, T. Koike and M. Akita, Angew. Chem., Int. Ed., 2012, 51, 9567 CrossRef CAS;
(b) Y. Yasu, T. Koike and M. Akita, Org. Lett., 2013, 15, 2136 CrossRef CAS;
(c) S. Mizuta, S. Verhoog, K. M. Engle, T. Khotavivattana, M. O'Duill, K. Wheelhouse, G. Rassias, M. M′edebielle and V. Gouverneur, J. Am. Chem. Soc., 2013, 135, 2505 CrossRef CAS;
(d) J.-J. Dai, C. Fang, B. Xiao, J. Yi, J. Xu, Z.-J. Liu, X. Lu, L. Liu and Y. Fu, J. Am. Chem. Soc., 2013, 135, 8436 CrossRef CAS;
(e) Y. Yasu, Y. Arai, R. Tomita, T. Koike and M. Akita, Org. Lett., 2014, 16, 780 CrossRef CAS.
-
(a) M. Oishi, H. Kondo and H. Amii, Chem. Commun., 2009, 1909 RSC;
(b) Y. Wu, G. Lu, T. Yuan, Z. Xu, L. Wan and C. Cai, Chem. Commun., 2016, 52, 13668 RSC.
- C.-P. Zhang, Z.-L. Wang, Q.-Y. Chen, C.-T. Zhang, Y.-C. Gu and J.-C. Xiao, Chem. Commun., 2011, 47, 6632 RSC.
-
(a) P. Novak, A. Lishchynskyi and V. V. Grushin, J. Am. Chem. Soc., 2012, 134, 16167 CrossRef CAS;
(b) Z. He, R. Zhang, M. Hu, L. Li, C. Ni and J. Hu, Chem. Sci., 2013, 4, 3478 RSC;
(c) Y.-F. Wang, G. H. Lonca and S. Chiba, Angew. Chem., Int. Ed., 2014, 53, 1067 CrossRef CAS;
(d) I. Saidalimu, E. Tokunaga and N. Shibata, ACS Catal., 2015, 5, 4668 CrossRef CAS;
(e) X. Su, H. Huan, Y. Yuan and Y. Li, Angew. Chem., Int. Ed., 2017, 56, 1338 CrossRef CAS;
(f) T. Kawamoto, R. Sasaki and A. Kamimura, Angew. Chem., Int. Ed., 2017, 56, 1342 CrossRef CAS;
(g) B. Jiang, X. Zhang and C. Yang, Org. Chem. Front., 2018, 5, 1724 RSC.
-
(a) T. Umemoto and S. Ishihara, J. Am. Chem. Soc., 1993, 115, 2156 CrossRef CAS;
(b) T. Umemoto and K. Adachi, J. Org. Chem., 1994, 59, 5692 CrossRef CAS;
(c) J. C. Blazejewski, M. P. Wilmshurst, M. D. Popkin, C. Wakselman, G. Laurent, D. Nonclercq, A. Cleeren, Y. Ma, H. S. Seo and G. Leclercq, Bioorg. Med. Chem., 2003, 11, 335 CrossRef CAS;
(d) Y. Itoh and K. Mikami, Org. Lett., 2005, 7, 649 CrossRef CAS;
(e) K. Mikami, Y. Tomita, Y. Ichikawa, K. Amikura and Y. Itoh, Org. Lett., 2006, 8, 4671 CrossRef CAS PubMed;
(f) V. Petrik and D. Cahard, Tetrahedron Lett., 2007, 48, 3327 CrossRef CAS;
(g) K. Sato, T. Yuki, A. Tarui, M. Omote, I. Kumadaki and A. Ando, Tetrahedron Lett., 2008, 49, 3558 CrossRef CAS;
(h) K. Sato, M. Higashinagata, T. Yuki, A. Tarui, M. Omote, I. Kumadaki and A. Ando, J. Fluorine Chem., 2008, 129, 51 CrossRef CAS;
(i) K. Sato, T. Yuki, R. Yamaguchi, T. Hamano, A. Tarui, M. Omote, I. Kumadaki and A. Ando, J. Org. Chem., 2009, 74, 3815 CrossRef CAS;
(j) V. Matoušek, A. Togni, V. Bizet and D. Cahard, Org. Lett., 2011, 13, 5762 CrossRef.
-
(a) D. Ravelli, M. Fagnoni and A. Albini, Chem. Soc. Rev., 2013, 42, 97 RSC;
(b) D. P. Hari and B. König, Angew. Chem., Int. Ed., 2013, 52, 4734 CrossRef CAS;
(c) C. K. Prier, D. A. Rankic and D. W. C. MacMillan, Chem.
Rev., 2013, 113, 5322 CrossRef CAS;
(d) J. Xie, H. Jin and A. S. K. Hashmi, Chem. Soc. Rev., 2017, 46, 5193 RSC.
-
(a) H. Tan, H. Li, W. Ji and L. Wang, Angew. Chem., Int. Ed., 2015, 54, 8374 CrossRef CAS;
(b) W. Ji, H. Tan, M. Wang, P. Li and L. Wang, Chem. Commun., 2016, 52, 1462 RSC;
(c) J. Xie, J. Li, T. Wurm, V. Weingand, H.-L. Sung, F. Rominger, M. Rudolph and A. S. K. Hashmi, Org. Chem. Front., 2016, 3, 841 RSC;
(d) S. Yang, P. Li and L. Wang, Org. Lett., 2017, 19, 3386 CrossRef CAS;
(e) S. Yang, H. Tan, W. Ji, X. Zhang, P. Li and L. Wang, Adv. Synth. Catal., 2017, 359, 443 CrossRef CAS;
(f) L. Zhao, P. Li, X. Xie and L. Wang, Org. Chem. Front., 2018, 5, 1689 RSC;
(g) W. Ji, P. Li, S. Yang and L. Wang, Chem. Commun., 2017, 53, 8482 RSC.
- C. Shen, J. Xu, B. Ying and P. Zhang, ChemCatChem, 2016, 8, 3560 CrossRef CAS.
- H. Yi, C. Bian, X. Hu, L. Niu and A. Lei, Chem. Commun., 2015, 51, 14046 RSC.
Footnote |
† Electronic supplementary information (ESI) available. See DOI: 10.1039/c8qo01079j |
|
This journal is © the Partner Organisations 2019 |