DOI:
10.1039/C7RA11212B
(Paper)
RSC Adv., 2017,
7, 55074-55080
Fabrication and evaluation of Rb2Co(H2P2O7)2·2H2O/waterborne polyurethane nanocomposite coating for corrosion protection aspects
Received
11th October 2017
, Accepted 27th November 2017
First published on 4th December 2017
Abstract
Here we investigate the influence of new acidic pyrophosphate (Rb2Co(H2P2O7)2·2H2O) (DP) incorporation in waterborne polyurethane (WBPU) coatings on the corrosion protection efficiency of WBPU coatings for carbon steel in 3.5% NaCl solution. We used potentiodynamic polarization, electrochemical frequency modulation (EFM) and pull-off adhesion and O2 gas permeability measurements to measure the corrosion protection efficiency of the WBPU coating. The dispersion of the DP nano-particles into the WBPU matrix was characterized via TEM observations. We find that the corrosion resistance and mechanical properties of the WBPU coating increase with incorporation of DP nano-particles into the WBPU coating.
1. Introduction
Carbon steel is the most important alloy used in petroleum pipelines. However, its main drawback is corrosion.1 The integration between cathodic protection and organic coatings can establish long-term protection for petroleum pipelines.2
Polyurethane coatings are used in a broad range of construction applications under different conditions, especially on cathodically protected surfaces.3,4
Waterborne polyurethane coatings are being progressively used instead of coating with organic solvent to minimize the release of volatile organic solvents.5 Waterborne polyurethane has been vastly used as an effective coating for protecting metallic surfaces from corrosion.6
Polyurethane has hydroscopic susceptibility and lacunar structure which make its coating permeable to aggressive agents.7 These drawbacks accelerate the corrosion of the metallic substrate over time.8 In this status, the incorporation of nanoparticles in organic coatings is a favorable approach for enhancing corrosion resistance of polyurethane coatings.9,10 The improvement of the corrosion resistance and mechanical properties of organic coating using inorganic materials is a promising approach for coating system.11–13
Recent works showed that inorganic materials improves the corrosion resistance of organic coatings by enlarging the route of corrosive ions and water in the coating to reach the metal/coating interface and enhance the barrier effects of coating matrix.14,15
In our previous works the effects of new inorganic materials on corrosion resistance and mechanical properties of organic coating were studied.16–20 We proved in these works that the nanoparticles of inorganic materials improve the corrosion resistance and mechanical properties of organic coating. As to continue these investigations, the current work intends to study the effect of the new acidic pyrophosphate Rb2Co(H2P2O7)2·2H2O (DP) incorporation in waterborne polyurethane (WBPU) coatings on the corrosion protection efficiency of WBPU coating.
Acidic metal pyrophosphates are characterized by their biological uses. Moreover, they are used as additive in fertilizers, or even in medicine.21 In acidic pyrophosphates, various oxoanions (HP2O7)3−, (H2P2O7)2− and (H3P2O7)− are involved in the molecular structure. These oxoanions are interconnected by strong hydrogen bonds, leading to various geometries (i.e. chains, ribbons, layers or three-dimensional network).21
The practical tests have been conducted on samples of carbon steel immersed in salt solution (3.5% NaCl) and measured by means of electrochemical and mechanical methods.
2. Experimental method
2.1. Materials and chemicals
A carbon steel panel with the following chemical composition: (wt%); 0.06 C; 0.02 Cu; 0.7 Mn; 0.005 P; 0.012 Ni; 0.06 Si; 0.015 Cr; 0.004 Mo; 0.001 S; 0.002 V and Fe (bal.), was used as a metallic substrate. The metallic substrates used in the electrochemical experiment were mechanically cut into 3.5 cm × 2.5 cm × 0.3 cm dimensions, then abraded with emery papers up to 1200 grit, and washed with absolute ethanol and acetone.22 The clean metallic substrates were dried at room temperature and stored in a moisture free desiccator before coating.
Waterborne polyurethane (the solid content is 37 wt%) and isocyanate based hardener were purchased from DOW Chemical Company and Bayer Company, respectively.
The aggressive environment used was 3.5 wt% NaCl solution. All chemicals were of AR grade and the solutions were prepared using deionized water.
2.2. Synthesis of Rb2Co(H2P2O7)2·2H2O
Single crystals of Rb2Co(H2P2O7)2·2H2O have been prepared in solution dissolving stoechiometrical amounts of Rb2CO3 (1 mmol) and CoCl2·6H2O (0.5 mmol) in a solution made of K4P2O7 in water (1 mmol). The mixture, to which few drops of concentrated HCl (2 M) were added, has been stirred for 4 h, and the resulting solution was stored at room temperature. After one week, well shaped pink crystals deposited, which have been filtered off and washed with a mixture ethanol–water (80
:
20).
2.3. Preparation of WBPU/DP nano-particles coating
Crystals of the DP were thoroughly grounded and sieved as to get nanoparticles, these were added into the waterborne polyurethane in different concentrations to obtain 0.2%, 0.5% and 1.0% by weight in the final coating formula. DP nano-particles were completely dispersed in WBPU coatings by mechanical stirring at 1500 rpm for 40 min, followed by ultrasonication process for about 15 min. Isocyanate based hardener was added to the coating formula at 1
:
3 ratio to prepare a homogeneous and stable coating formula.
There are limitations of adding nano-particles above 1.0%, because of homogeneity and to slow down nano-particles re-aggregation after mechanical stirring removal.
2.4. Characterization
A single crystal was selected and X-ray diffraction (XRD) data were collected on an Oxford Diffraction XCALIBUR four-circles X-ray diffractometer using graphite monochromatized MoKα radiation (λ = 0.7173 Å) equipped with a SAPPHIR CCD two-dimensional detector.
A transmission electron microscope (Model: Jeol-Jem 1200EX II equipped with TEM) was used to determine the size of DP nano-particles and to evaluate the dispersion and distribution of DP nano-particles in WBPU.
2.5. Preparation of the coated substrates
The prepared coatings were applied on clean carbon steel panels by conventional spraying process. The thickness of dry coating film (measured by hand held micrometer, B.C. Ames Co.) was in the range of 65 ± 10 μm. The coated substrates were stored in a moisture free desiccator before testing.
2.6. Electrochemical experiments
The Corrosion resistance property of coatings was conducted using potentiodynamic polarization and EFM measurements.
The electrochemical set-up composed of three electrodes layout in a glass cell, with a Pt counter electrode and a saturated calomel reference electrode (SCE). The experiments were conducted in a 100 ml volume cell at 298 K using a temperature control water bath.
All electrochemical measurements were carried out with ACM instruments Potentiostat/Galvanostat (Gill AC Serial no. 947).
For potentiodynamic polarization experiments, open circuit potential (OCP) of system after immersion for 2 h and 7 days in corrosive solution was recorded. Then, the Tafel plot was plotted by sweeping the applied potential from −0.25 to +0.25 V with respect to OCP with a constant sweep rate of 1.0 mV s−1. EFM measurements were implemented by applying potential perturbation signal with amplitude of 10 mV with two sine waves of 2 and 5 Hz.
All electrochemical experiments were performed in triplicate to guarantee reproducibility of the results and the mean values were recorded.
2.7. Pull-off adhesion experiments
Pull-off adhesion testers (PAT model GM01/6.3 kN) was used to determine the adhesion strength of WBPU coatings coatings after 10 days of immersion in 3.5 wt% NaCl solution. All the experiments were repeated three times, and good reproducibility of the results was observed.
2.8. O2 gas barrier property experiments
O2 gas barrier property of prepared coating was determined at 298 K using Oxygen Permeation Analyzers (model 8501, Illinois Instruments, Inc.). The measurements were occurred according ASTM D3985 standard test methods.
3. Results and discussion
3.1. Crystal structure
Rb2Co(H2P2O7)2·2H2O is isostructural to Rb2M(H2P2O7)2·2H2O (M = Mg, Zn).21 It crystallizes in the triclinic system (P\
). Its crystal structure might be desribed as a 3D framework made of corners and/or edges sharing [RbO7], [CoO4(H2O)2] and [H2P2O7] polyedra, Fig. 1 depicts a projection of the crystal structure onto (010). In another view, [CoO4(H2O)2] is coordinated by two bidentate [H2P2O7] pyrophosphate groups to form the phosphocobaltate [Co(H2P2O7)2·2H2O] entity. These entities are linked through Rb–O interactions, and intricate H-bonds from the hydroxyl groups, to extend tridimentionally. Fig. 2 shows the coordination scheme around the metals in the framework of the title compound.
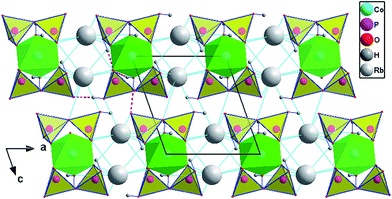 |
| Fig. 1 Rb2Co(H2P2O7)2·2H2O, a projection of the framework onto crystallographic plane (010), H-bonds as dashed lines. | |
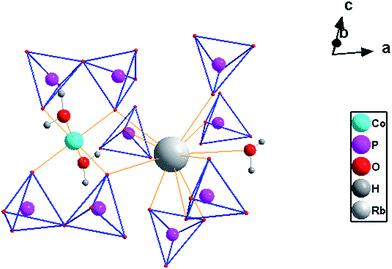 |
| Fig. 2 Metal coordination in Rb2Co(H2P2O7)2·2H2O, for clarity only one tetrahedron from [H2P2O7] is presented around Rb. | |
Rb2Co(H2P2O7)2·2H2O crystallizes in the triclinic system (P\
) with the cell parameters (Å, °): 6.980(1), 7.370(1), 7.816(1), 81.74(1), 70.35(1), 86.34(1); V = 374.68(9) Å3, Z = 2.
Further details on the crystal structure investigations of the title compound might be obtained from the Inorganic Crystal Structure Database, FIZ, Hermann von Helmholtz Platz 1, 76344 Eggenstein-Leopoldshafen, Germany; fax: (+49) 7247 808 132; http://www2.fiz-karlsruhe.de/icsd_home.html, on quoting the depository number CSD 421807.
3.2. TEM observation
The TEM image of DP nano-particles is shown in Fig. 3. The TEM analysis demonstrates that the particle size of DP nano-particles was found to be around 70 nm.
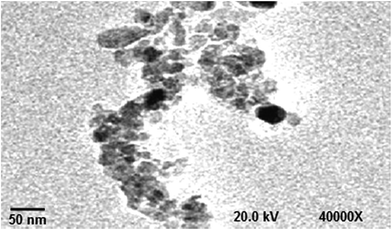 |
| Fig. 3 TEM image of DP nano-particles. | |
The TEM image of WBPU/DP nano-particles nanocomposite (see Fig. 4) confirms that the DP nano-particles were uniformly dispersed in the WBPU matrix.
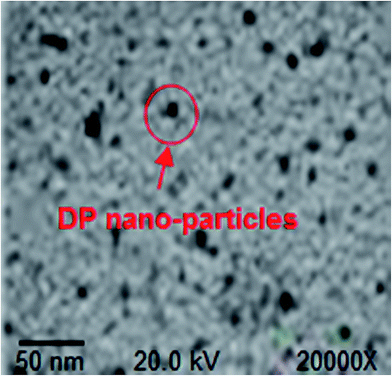 |
| Fig. 4 TEM image of WBPU/DP nano-particles nanocomposite. | |
3.3. Corrosion resistance property of WBPU coating in the presence of Rb2Co(H2P2O7)2·2H2O
3.3.1 Polarization measurements. Potentiodynamic polarization test (Tafel plot) was employed to study the effect of the incorporation of Rb2Co(H2P2O7)2·2H2O nanoparticles with the coating on the corrosion resistance property of WBPU coatings after exposure to the saline solution (3.5% NaCl) (Fig. 5). The polarization curves were obtained after stabilization for 2 h and the electrochemical parameters (corrosion potential Ecorr and corrosion current density jcorr) obtained from polarization curves are presented in Table 1.
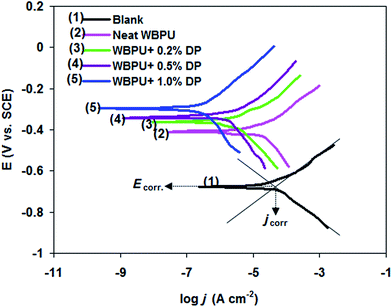 |
| Fig. 5 Tafel plots for carbon steel coated by waterborne polyurethane (WBPU) coating containing pyrophosphate (DP) after 2 h of immersion in 3.5% NaCl solution at 298 K. | |
Table 1 Electrochemical parameters and the corresponding protection efficiency for carbon steel coated by waterborne polyurethane (WBPU) coating containing pyrophosphate (DP) immersed in 3.5% NaCl solution at 298 K
Samples |
Ecorr V (vs. SCE) |
jcorr μA cm−2 |
ηj% |
Bare carbon steel |
−0.676 |
63.09 |
— |
Neat WBPU |
−0.411 |
13.08 |
79.26 |
WBPU + 0.2% DP |
−0.363 |
4.43 |
92.97 |
WBPU + 0.5% DP |
−0.342 |
2.28 |
96.38 |
WBPU + 1.0% DP |
−0.292 |
0.25 |
99.60 |
The Ecorr of bare carbon steel is about −0.676 with jcorr of about 63.09 μA cm−2. In the case of neat WBPU coating, Ecorr shifts to more positive potentials (−0.411 V) and jcorr decreases to 13.08 μA cm−2, confirming that the coated carbon steel substrate by neat WBPU coating is still susceptible to corrosion to some extent. On the other hand, WBPU + DP coated substrate exhibits more positive Ecorr with a remarkable decrease of jcorr (Table 1). The noble shift of Ecorr accompanying with decrease of jcorr, suggest that the incorporation of different concentrations of DP nano-particles into WBPU coating improves the corrosion resistance of the mild carbon steel substrate remarkably.23
The protection efficiency (ηj%) of the coatings was defined as:24,25
|
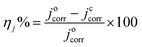 | (1) |
where
jocorr and
jccorr are the corrosion current densities in the absence and presence of coatings, respectively.
The calculated ηj% values obtained from polarization measurements are listed in Table 1. The protection efficiency ηj% of the WBPU + DP coatings was found to be significantly increased as compared to neat WBPU (Table 1). The ηj% of the WBPU + DP coatings was increased as the percentage of DP nano-particles increased. Coated carbon steel sample with WBPU + 1.0% DP, showed the highest the corrosion resistance (ηj% = 99.60).
In addition, coatings have been modified with nano-particles to achieve desirable properties such as increased adhesion and cohesion.26 Here, cohesion reflects the strength and fracture resistance of the coating material, while adhesion indicates the interfacial bond strength of coatings or adhesives.27 This improvement in the corrosion resistance of WBPU coating in the presence of DP nano-particles is attributed to; DP nano-particles are well dispersed in WBPU coating, which restrict the diffusion of the corrosive ions and water through the WBPU coating film.28,29 Moreover, the large surface area and the small size of DP nano-particles, absorb more WBPU coating on its surface which reinforces the density of the WBPU coating films, that way the transport paths for the corrosive ions and water to pass through the WBPU coating become longer and consequently inhibiting the corrosion process.30
The effect of long time immersion of WBPU + 1.0% DP coating was shown in Fig. 6. It is observed that after immersion of the coated carbon steel by WBPU + 1.0% DP, there is a slightly increase in the magnitude of the corrosion current (from 0.25 μA cm−2 to 2.9 μA cm−2) with increasing the immersion time from 2 h to 7 days. This is referring to the high corrosion resistance nature of the WBPU + 1.0% DP coating.
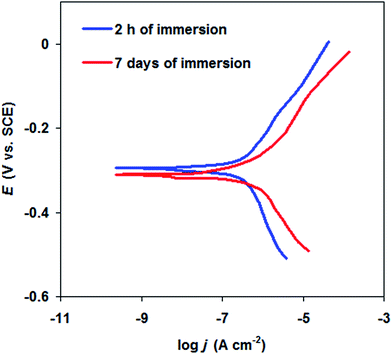 |
| Fig. 6 Tafel plots for carbon steel coated by WBPU + 1.0% DP after 2 h and 7 days of immersion in 3.5% NaCl solution at 298 K. | |
3.3.2 Electrochemical frequency modulation. Electrochemical Frequency Modulation (EFM) is a powerful non-destructive method for measuring the corrosion rate of coating system. The EFM technique was used for monitoring of the corrosion rate of various types of corroding systems within reasonable time without prior knowledge of Tafel parameters by applying a small polarizing signal.The EFM intermodulation spectra of uncoated and coated carbon steel by WBPU coating containing DP nano-particles immersed in 3.5% NaCl solution at 298 K are shown in Fig. 7. The parameters obtained from EFM spectra (jcorr(EFM) and the causality factors CF2, CF3) are listed in Table 2.
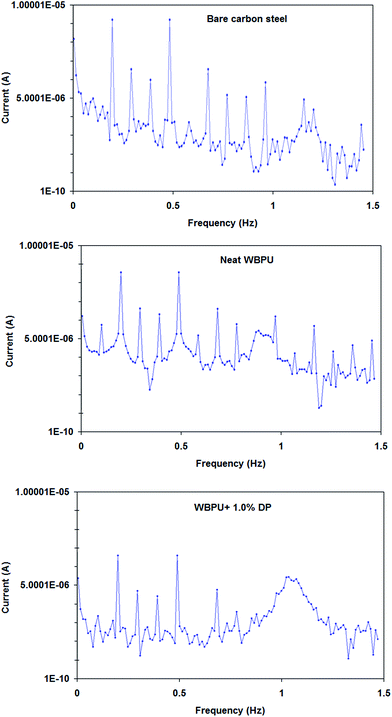 |
| Fig. 7 EFM intermodulation spectra of uncoated and coated carbon steel by WBPU coating containing DP nano-particles immersed in 3.5% NaCl solution at 298 K. | |
Table 2 Electrochemical kinetic parameters obtained by EFM technique, and the corresponding protection efficiency for carbon steel coated by waterborne polyurethane (WBPU) coating containing pyrophosphate (DP) immersed in 3.5% NaCl solution at 298 K
Samples |
CF2 |
CF3 |
jcorr(EFM) μA cm−2 |
ηEFM% |
Bare carbon steel |
1.77 |
2.44 |
69.32 |
— |
Neat WBPU |
1.84 |
2.67 |
19.38 |
72.04 |
WBPU + 0.2% DP |
1.89 |
2.84 |
7.94 |
88.54 |
WBPU + 0.5% DP |
1.91 |
2.75 |
4.85 |
93.00 |
WBPU + 1.0% DP |
1.93 |
2.82 |
1.99 |
97.12 |
It seems that the values of the causality factors CF2, CF3 for uncoated and coated carbon steel substrates are around the theoretical values (2 and 3), signifying the accuracy of the obtained results.31
According to Table 2, all coated carbon steel show higher jcorr(EFM) than uncoated substrates; meanwhile, WBPU coatings containing DP nano-particles show significantly greater jcorr(EFM) values. From EFM data, the protection efficiency (ηEFM%) of the coatings can be calculated using eqn (2):32
|
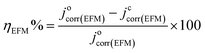 | (2) |
where
jocorr(EFM) and
jccorr(EFM) are the corrosion current densities obtained by EFM data in the absence and presence of coatings respectively.
The calculated ηEFM% values are listed in Table 2. According to these results, DP nano-particles tend to agglomerate in WBPU matrix by increasing DP wt%. The WBPU coating with 0.1 wt% DP nano-particles shows the best corrosion protection performance (97.12%). This is consistent with the finding that obtained from polarization measurements.
3.4. Mechanical property of WBPU coating in the presence of Rb2Co(H2P2O7)2·2H2O
The mechanical property of WBPU coating in the absence and presence of DP nano-particles is conducted using pull-off adhesion measurements and the results are presented in Fig. 8. The average pull-off adhesion strength of WBPU coatings enhances by incorporation of different amount of DP nano-particles. The average adhesion strength of the WBPU coating without DP nano-particles was 3.2 MPa, while average adhesion strengths of 6.6 MPa, 8.8 and 10.5 MPa were obtained at 0.2 wt%, 0.5% wt% and 1.0 wt% of DP nano-particles, respectively.
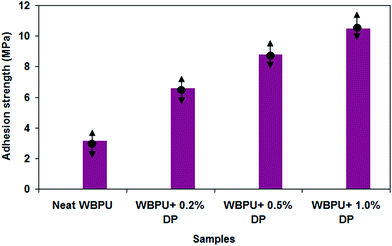 |
| Fig. 8 Pull-off adhesion of the coatings of WBPU-coated carbon steel in the absence and presence of different concentrations of DP nano-particles. | |
The adhesion strength of WBPU highly depends on the chain extenders and their content.33 Neat WBPU coating adheres to carbon steel surface via chemical adhesion supported by mechanical interlocking adhesion. The reactive functional groups of WBPU coating such as NH and CO groups tend to adhere to carbon steel surface via formation of chemically bond.34 In these applications the formation of covalent chemical bonds occurs across the interface. Coating systems containing reactive functional groups tend to adhere more strongly to metal surface containing similar reactive functional groups.23
The incorporation of DP nano-particles is also believed to diminish the porosity of the WBPU coating.35,36 Moreover, the DP nano-particles can suppresses the coating cracking when the WBPU coating is exposed to an external stress and it will result in the improvement of the coating flexibility. This causes better adhesion of WBPU coatings containing DP nano-particles than neat WBPU.
3.5. O2 gas barrier property of WBPU coating in the presence of Rb2Co(H2P2O7)2·2H2O
O2 gas permeability of WBPU coating in the absence and presence of DP nano-particles at 298 K was presented in Table 3. The incorporation of DP nano-particles into WBPU coating causes a significant decrease in the permeation of oxygen across the coating thickness. The best barrier performance was recorded for the coating with the highest concentration of Rb2Co(H2P2O7)2·2H2O (i.e. WBPU + 1.0% DP). It has been explained on the basis that the incorporation of DP nano-particles into WBPU coating can create a tortuous path for O2 gas molecules. This causes an increase of the total path of the gas.37 This results support the corrosion protection data.
Table 3 O2 gas permeability of WBPU coating in the absence and presence of Rb2Co(H2P2O7)2·2H2O at 298 K
Coating type |
O2 permeability (cc m−2 day) |
Neat WBPU |
48.3 |
WBPU + 0.2% DP |
13.4 |
WBPU + 0.5% DP |
5.5 |
WBPU + 1.0% DP |
4.0 |
4. Conclusions
The effect of nano-particles of new acidic pyrophosphates (Rb2Co(H2P2O7)2·2H2O) on the corrosion protection efficiency of WBPU coatings for carbon steel in saline solution (3.5% NaCl) was assessed by electrochemical and mechanical methods. Potentiodynamic polarization and EFM curves proved that the addition of DP nano-particles had improved the corrosion protection performance of WBPU coating. Increment of the DP nano-particles concentration exhibited a significant effect on the coating performance. The superior the corrosion protection efficiency of the WBPU coating was achieved by the addition of 1.0 wt% of DP nano-particles. Further, WBPU coating containing DP nano-particles showed the highest pull-off adhesion strength, demonstrating the enhanced mechanical performance of WBPU coating with the addition of DP nano-particles.
Conflicts of interest
There are no conflicts to declare.
References
- M. A. Deyab, The influence of different variables on the electrochemical behavior of mild steel in circulating cooling water containing aggressive anionic species, J. Solid State Electrochem., 2009, 13, 1737–1742 CrossRef CAS.
- A. S. Ismail, Nano-sized aluminum coatings from aryl-substituted imidazolium cation based ionic liquid, Egypt. J. Pet., 2016, 25, 525–530 CrossRef.
- S. Huang, J. Xiao, Y. Zhu and J. Qu, Synthesis and properties of spray-applied high solid content two component polyurethane coatings based on polycaprolactone polyols, Prog. Org. Coat., 2017, 106, 60–68 CrossRef CAS.
- J. V. Nardeli, D. V. Snihirova, C. S. Fugivara, M. F. Montemor, E. R. P. Pinto, Y. Messaddecq and A. V. Benedetti, Localised corrosion assessement of crambe-oil-based polyurethane coatings applied on the ASTM 1200 aluminum alloy, Corros. Sci., 2016, 82, 422–435 CrossRef.
- J. Li, J. Cui, J. Yang, Y. Li, H. Qiu and J. Yang, Reinforcement of graphene and its derivatives on the anticorrosive properties of waterborne polyurethane coatings, Compos. Sci. Technol., 2016, 129, 30–37 CrossRef CAS.
- J. Y. Kwon and H. D. Kim, Preparation and properties of acid-treated multiwalled carbon nanotube/waterborne polyurethane nanocomposites, J. Appl. Polym. Sci., 2005, 96, 595–604 CrossRef CAS.
- Y. Li, Z. Yang, H. Qiu, Y. Dai, Q. Zheng, J. Li and J. Yang, Self-aligned graphene as anticorrosive barrier in waterborne polyurethane composite coatings, J. Mater. Chem. A, 2014, 2, 14139–14145 CAS.
- O. C. Compton, S. Kim, C. Pierre, J. M. Torkelson and S. T. Nguyen, Crumpled grapheme nanosheets as highly effective barrier property enhancers, Adv. Mater., 2010, 22, 4759–4763 CrossRef CAS PubMed.
- G. Christopher, M. A. Kulandainathan and G. Harichandran, Biopolymers nanocomposite for material protection: Enhancement of corrosion protection using waterborne polyurethane nanocomposite coatings, Prog. Org. Coat., 2016, 99, 91–102 CrossRef CAS.
- M. A. Deyab, Effect of carbon nano-tubes on the corrosion resistance of alkyd coating immersed in sodium chloride solution, Prog. Org. Coat., 2015, 85, 146–150 CrossRef CAS.
- P. Li, X. He, T.-C. Huang, K. L. White, X. Zhang, H. Liang, R. Nishimura and H.-J. Sue, Highly effective anti-corrosion epoxy spray coatings containing self-assembled clay in smectic order, J. Mater. Chem. A, 2015, 3, 2669–2676 CAS.
- P. Sambyal, G. Ruhi, R. Dhawan and S. K. Dhawan, Designing of smart coatings of conducting polymer poly(aniline-co-phenetidine)/SiO2 composites for corrosion protection in marine environment, Surf. Coat. Technol., 2016, 303, 362–371 CrossRef CAS.
- J.-M. Yeh, S.-J. Liou, C.-Y. Lai, P.-C. Wu and T.-Y. Tsai, Enhancement of Corrosion Protection Effect in Polyaniline via the Formation of Polyaniline–Clay Nanocomposite Materials, Chem. Mater., 2001, 13, 1131–1136 CrossRef CAS.
- K. Qi, Y. Sun, H. Duan and X. Guo, A corrosion-protective coating based on a solution-processable polymer-grafted graphene oxide nanocomposite, Corros. Sci., 2015, 98, 500–506 CrossRef CAS.
- A. Yabuki and M. Sakai, Self-healing coatings of inorganic particles using a pH-sensitive organic agent, Corros. Sci., 2011, 53, 829–833 CrossRef CAS.
- M. A. Deyab, R. Ouarsal, A. M. Al-Sabagh, M. Lachkar and B. El Bali, Enhancement of corrosion protection performance of epoxy coating by introducing new hydrogenphosphate compound, Prog. Org. Coat., 2017, 107, 37–42 CrossRef CAS.
- M. A. Deyab, G. Mele, A. M. Al-Sabagh, E. Bloise, D. Lomonaco, S. E. Mazzetto and C. D. S. Clemente, Synthesis and characteristics of alkyd resin/M-Porphyrins nanocomposite for corrosion protection application, Prog. Org. Coat., 2017, 105, 286–290 CrossRef CAS.
- M. A. Deyab, K. Eddahaoui, R. Essehli, S. Benmokhtar, T. Rhadfi, A. De Riccardis and G. Mele, Influence of newly synthesized titanium phosphates on the corrosion protection properties of alkyd coating, J. Mol. Liq., 2016, 216, 699–703 CrossRef CAS.
- M. A. Deyab, A. De Riccardis and G. Mele, Novel epoxy/metal phthalocyanines nanocomposite coatings for corrosion protection of carbon steel, J. Mol. Liq., 2016, 220, 513–517 CrossRef CAS.
- M. A. Deyab, A. A. Nada and A. Hamdy, Comparative study on the corrosion and mechanical properties of nano-composite coatings incorporated with TiO2 nano-particles, TiO2 nano-tubes and ZnO nano-flowers, Prog. Org. Coat., 2017, 105, 245–251 CrossRef CAS.
- R. Essehli, B. El Bali, M. Lachkar and G. Cruciani, Two new acidic diphosphates Rb2M(H2P2O7)2·2H2O (M= Zn and Mg): Crystal structures and vibrational study, J. Alloys Compd., 2010, 492, 358–362 CrossRef CAS.
- M. A. Deyab and S. T. Keera, Cyclic voltammetric studies of carbon steel corrosion in chloride-formation water solution and effect of some inorganic salts, Egypt. J. Pet., 2012, 21, 31–36 CrossRef CAS.
- S. Pourhashem, M. R. Vaezi, A. Rashidi and M. R. Bagherzadeh, Exploring corrosion protection properties of solvent basedepoxy-graphene oxide nanocomposite coatings on mild steel, Corros. Sci., 2017, 115, 78–92 CrossRef CAS.
- C. Xing, Z. Zhang, L. Yu, L. Zhangb and G. A. Bowmaker, Electrochemical corrosion behavior of carbon steel coated by polyaniline copolymers micro/nanostructures, RSC Adv., 2014, 4, 32718–32725 RSC.
- K.-C. Chang, S.-T. Chen, H.-F. Lin, C.-Y. Lin, H.-H. Huang, J.-M. Yeh and Y.-H. Yu, Effect of clay on the corrosion protection efficiency of PMMA/Na+-MMT clay nanocomposite coatings evaluated by electrochemical measurements, Eur. Polym. J., 2008, 44, 13–23 CrossRef CAS.
- M. K. Madhup, N. K. Shah and N. R. Parekh, The Effect of Zinc Oxide Nanoparticles on Cohesive and Adhesive Bond of Epoxy/Amine Coating on Carbon Steel Substrate, J. Appl. Chem., 2017, 10, 47–58 Search PubMed.
- R. Lacombe, Adhesion measurement methods: theory and practice, CRC Press, Boca Raton, 2006 Search PubMed.
- B. A. Bhanvase and S. H. Sonawane, New approach for simultaneous enhancement of anticorrosive and mechanical properties of coatings: Application of water repellent nano CaCO3–PANI emulsion nanocomposite in alkyd resin, Chem. Eng. J., 2010, 156, 177–183 CrossRef CAS.
- L. Ejenstam, M. Tuominen, J. Haapanen, J. M. Mäkelä, J. Pan, A. Swerin and P. M. Claesson, Appl. Surf. Sci., 2015, 357, 2333–2342 CrossRef CAS.
- L. H. Yang, F. C. Liu and E. H. Han, Effects of P/B on the properties of anticorrosive coatings with different particle size, Prog. Org. Coat., 2005, 53, 91–99 CrossRef CAS.
- E. B. Ituen, A. O. James and O. Akaranta, Fluvoxamine-based corrosion inhibitors for J55 steel in aggressive oil and gas well treatment fluids, Egypt. J. Pet., 2017, 26, 745–756 CrossRef.
- M. A. Amin, M. A. Ahmed, H. A. Arida, T. Arslan, M. Saracoglu and F. Kandemirli, Monitoring corrosion and corrosion control of iron in HCl by non-ionic surfactants of the TRITON-X series – Part II. Temperature effect, activation energies and thermodynamics of adsorption, Corros. Sci., 2011, 53, 540–548 CrossRef CAS.
- S. Mohammadi, F. A. Taromi, H. Shariatpanahi, J. Neshati and M. Hemmati, Electrochemical and anticorrosion behavior of functionalized graphitenanoplatelets epoxy coating, J. Ind. Eng. Chem., 2014, 20, 4124–4139 CrossRef CAS.
- M. M. Rahman, M. H. Zahir and H. D. Kim, Synthesis and Properties of Waterborne Polyurethane (WBPU)/Modified Lignin Amine (MLA) Adhesive: A Promising Adhesive Material, Polymers, 2016, 8, 318–329 CrossRef.
- P. A. Sørensen, S. Kiil, K. Dam-Johansen and C. E. Weinell, Anticorrosive coatings: a review, J. Coat. Technol. Res., 2009, 6, 135–176 CrossRef.
- Y. Hao, F. Liu, H. Shi, E. Han and Z. Wang, The influence of ultra-fine glass fibers on the mechanical and anticorrosion properties of epoxy coatings, Prog. Org. Coat., 2011, 71, 188–197 CrossRef CAS.
- M. Oner, A. A. Çol, C. Pochat-Bohatierb and M. Bechelany, Effect of incorporation of boron nitride nanoparticles on the oxygen barrier and thermal properties of poly(3-hydroxybutyrate-cohydroxyvalerate), RSC Adv., 2016, 6, 90973–90981 RSC.
|
This journal is © The Royal Society of Chemistry 2017 |