DOI:
10.1039/C7RA10326C
(Paper)
RSC Adv., 2017,
7, 47709-47714
Pillar[5]arene-based fluorescent polymer for selective detection and removal of mercury ions†
Received
17th September 2017
, Accepted 5th October 2017
First published on 10th October 2017
Abstract
A novel pillar[5]arene-based thioacetohydrazone functionalized fluorescent polymer was designed and synthesized. This polymer not only contains pillar[5]arene units as the fluorophore (signal transducer) but also embedded the thioacetohydrazone group as the ionophore (cation receptor). Therefore, it displays specificity response for mercury ion over other common cations (Mg2+, Ca2+, Zn2+, Co2+, Fe3+, Pb2+, Cd2+, Ni2+, Tb3+, Cu2+, Eu3+, Fe2+, Cr3+, Ag+ and La3+) in DMSO/H2O (1
:
1, v/v). Competitive cations did not show any significant changes in emission intensity and the fluorescence spectra detection limit was 8.12 × 10−7 M, indicating the high selectivity and sensitivity of the polymer towards Hg2+. Meanwhile, this polymer can efficiently remove Hg2+ from water.
Introduction
Because of their high toxicity and bioaccumulation, heavy metal ions released into the environment can lead to a wide range of severe diseases.1 Considerable efforts are accordingly being devoted to developing new methodologies for detection and removal of specific toxic metal ions.2 For example, many types of small molecules have been designed as fluorescent sensors for selective and sensitive detection of metal ions.3 On the other hand, various absorbents, such as porous silicas,4 hydrogels,5 nanoparticles,6 and metal–organic frameworks (MOFs),7 are being tested for the possible removal of toxic ions. However, most of the developed methods can only perform either the detection or the removal tasks separately, which limits their practical applications.
Pillararenes, a new kind of macrocyclic compounds, are composed of hydroquinone units linked by methylene bridges at the para positions.8 They have novel host–guest binding properties due to being easier to functionalize by different substituents on the benzene rings,9 thus functionalized pillararenes attracted a lot of attention of scientists.10 Since it has multiple benzene ring units, it has a certain luminescent properties.11 Therefore, pillararenes are used for fluorescence detection of ions,12 but most of them are used to identify iron ions.13 In addition, these reports rarely mentioned the corresponding ion can be removed. In order to extend the application of pillararenes, it is quite necessary to design and synthesize novel pillararenes for detection and removal of ions.
Polymers have been widely used as organic light-emitting diodes (OLEDs),14 thin-film transistors, chemical sensors,15 and in various photonic and electronic devices.16 However, the pillar[5]arene-based polymer have rarely been reported.17 On the other hand, the use of pillar[5]arene-based polymers to detect and remove ions hasn't been reported.
Herein, we designed and synthesized a pillar[5]arene-based fluorescent polymer for detecting and removing the metal ions. As a proof-of-concept, a thioacetohydrazone functionalized fluorescent polymer, PP5, was designed and applied for the selective sensing and effective removal of the toxic Hg2+ (Scheme 1). Although we have previously studied the selective detection and removal of mercury ions, these sensors are small molecules.18 However, this report is the use of pillar[5]arene-based polymer for the selective detection and removal of mercury ions. Although it has previously been reported that the use of pillar[5]arene-based pseudorotaxane to detect and remove mercury ions, it is the host–guest complex (no polymer). Importantly, the real-time fluorescence response did show efficient quenching of PP5 upon the addition of Hg2+. Excellent sensitivity and selectivity toward the Hg2+ detection were verified in the presence of other competitive cations. The removal ability of PP5 was further elucidated by the effective separation of Hg2+ from water. Meanwhile, PP5 can recycle detection and removal of Hg2+.
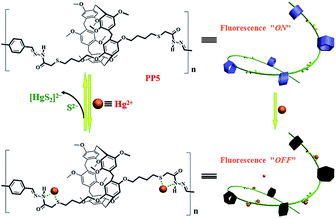 |
| Scheme 1 The pillar[5]arene-based polymer PP5 was applied for both fluorescent detection and removal of Hg2+. | |
Experimental
Materials and instruments
1,4-Dimethoxybenzene, boron trifluoride ethyl ether complex, 1,4-dibromobutane and ethyl mercaptoacetate were reagent grade and used as received. Solvents were either employed as purchased or dried by CaCl2. 1H NMR spectra were recorded on a Mercury-600BB spectrometer at 600 MHz and 13C NMR spectra were recorded on a Mercury-600BB spectrometer at 151 MHz. Chemical shifts are reported in ppm downfield from tetramethylsilane (TMS, δ scale with solvent resonances as internal standards). Melting points were measured on an X-4 digital melting-point apparatus (uncorrected). Mass spectra were performed on a Bruker Esquire 3000 plus mass spectrometer (Bruker-FranzenAnalytik GmbH Bremen, Germany) equipped with ESI interface and ion trap analyzer. The morphologies and sizes of the polymer were characterized using field emission scanning electron microscopy (FE-SEM, JSM-6701F) at an accelerating voltage of 8 kV. The X-ray diffraction analysis (XRD) was performed in a transmission mode with a Rigaku RINT2000 diffractometer equipped with graphite monochromated CuKα radiation (λ = 1.54073 Å). The infrared spectra were performed on a Digilab FTS-3000 Fourier transform-infrared spectrophotometer. Fluorescence spectra were recorded on a Shimadzu RF-5301PC spectrofluorophotometer.
Adsorption experiments
The adsorption experiments were performed at different mercury(II) concentration, corresponding adsorbent and room temperature (Table S1†). The experiments were carried out in 25 mL round-bottom flasks, with continuously stirring (usually for 5 h). The residual concentration of mercury(II) was determined by the inductively coupled plasma (ICP) analysis.
Synthetic procedures
Synthesis of 1,4-bis(4-bromobutoxy)benzene 1.
Hydroquinone (2.3 g, 20.0 mmol), K2CO3 (16.6 g, 120 mmol), KI (6.6 g, 40 mmol), 1,4-dibromobutane (34.6 g, 160 mmol) and acetone (400.0 mL) were added in a 500 mL round-bottom flask stirred at room temperature. The reaction mixture was stirred at reflux for 1.5 days. After the solid was filtered off, the solvent was evaporated and the residue was dissolved in CH2Cl2. Column chromatography (silica gel; petroleum ether
:
CH2Cl2 = 10
:
1) afforded a white solid (6.0 g, 80%). Mp 83–85 °C. 1H NMR (600 MHz, CDCl3) δ 6.83 (d, J = 0.8 Hz, 4H), 3.96 (t, J = 6.0 Hz, 4H), 3.52–3.25 (m, 4H), 2.10–1.88 (m, 8H).
Synthesis of a copillar[5]arene 2.
To a solution of 1,4-bis(4-bromobutoxy)benzene (1.90 g, 5.0 mmol) and 1,4-dimethoxybenzene (2.76 g, 20.0 mmol) in 1,2-dichloroethane (200 mL), paraformaldehyde (0.75 g, 25.0 mmol) was added under nitrogen atmosphere. Then boron trifluoride diethyl etherate (6.75 mL, 25 mmol) was added to the solution and the mixture was stirred at room temperature for 4 h and concentrated by rotary evaporation. The resultant oil was dissolved in CH2Cl2 and washed twice with H2O. The organic layer was dried over anhydrous Na2SO4 and evaporated to afford the crude product, which was isolated by flash column chromatography using petroleum ether/ethyl acetate (20
:
1, v/v) to give 2 (1.69 g, 34%) as a white solid. Mp 187–189 °C. 1H NMR (600 MHz, CDCl3) δ 6.84–6.74 (m, 10H), 3.87 (t, J = 5.9 Hz, 4H), 3.83–3.78 (m, 10H), 3.72 (t, J = 19.9 Hz, 24H), 3.33 (s, 4H), 1.94 (s, 4H), 1.84 (s, 4H). 13C NMR (151 MHz, CDCl3) δ 150.80 (s), 150.75 (s), 150.70 (s), 150.58 (s), 149.84 (s), 128.44 (s), 128.30 (s), 128.08 (s), 114.89 (s), 114.15 (s), 113.92 (s), 113.71 (s), 67.32 (s), 55.95 (d, J = 3.6 Hz), 55.76 (s), 55.70 (s), 33.34 (s), 30.55 (s), 29.75 (s), 29.48 (d, J = 5.2 Hz), 29.19 (s), 28.32 (s). ESI-MS m/z: (M + NH4)+ calcd for C51H64O10Br2N 1010.2871; found 1010.2878.
Synthesis of functionalized pillar[5]arene 3.
Copillar[5]arene 2 (0.5 g, 0.5 mmol) K2CO3 (0.28 g, 2 mmol) and ethyl mercaptoacetate (0.3 mL, 2.75 mmol) were added to acetone (80 mL). The solution was refluxed overnight. After the solid was filtered off, the solvent was evaporated and the residue was dissolved in CH2Cl2. Column chromatography (silica gel; petroleum ether
:
CH2Cl2 = 20
:
1) afforded a white solid (6.0 g, 80%). 1H NMR (600 MHz, CDCl3) δ 6.78 (dd, J = 14.3, 7.1 Hz, 10H), 3.85 (t, J = 5.8 Hz, 4H), 3.79–3.74 (m, 14H), 3.71 (s, 6H), 3.69 (s, 12H), 3.66 (s, 12H), 3.24 (s, 4H), 2.72 (t, J = 7.0 Hz, 4H), 1.87 (ddd, J = 26.5, 14.7, 7.9 Hz, 8H). 13C NMR (151 MHz, CDCl3) δ 170.88 (s), 151.71–148.89 (m), 129.38–126.85 (m), 116.55–112.46 (m), 67.71 (s), 55.73 (dd, J = 10.4, 5.9 Hz), 52.34 (s), 41.19 (s), 33.42 (s), 32.52 (s), 29.46 (s), 28.86 (s), 25.72 (s).
Synthesis of pillar[5]arene 4.
Functionalized pillar[5]arene 3 (0.5 g, 0.5 mmol) and hydrazine hydrate (0.3 mL, 6 mmol) were added to ethanol (20 mL). The solution was refluxed overnight. Then the solvent was removed by evaporation, you can afford a white solid. After white solid was washed by ethanol to obtain 4 as a white solid (0.48 g, 93%). 1H NMR (600 MHz, DMSO-d6) δ 9.11 (s, 2H), 6.78 (d, J = 3.4 Hz, 10H), 4.34 (s, 4H), 3.84 (s, 4H), 3.75–3.61 (m, 34H), 3.06 (s, 4H), 2.67 (s, 4H), 1.80 (dd, J = 29.0, 7.3 Hz, 8H). 13C NMR (151 MHz, DMSO-d6) δ 168.92 (s), 150.32 (s), 127.90 (s), 113.69 (s), 67.84 (s), 55.89 (s), 32.99 (s), 32.09 (s), 29.42 (s), 28.80 (s), 25.96 (s). ESI-MS m/z: (M + H)+ calcd for C55H71O12N4S2 1043.44; found 1043.2296.
Synthesis of polymer PP5.
To a solution of pillar[5]arene 4 (0.21 g, 0.2 mmol) in ethanol (20 mL), terephthalaldehyde (0.027 g, 0.2 mmol) and acetic acid (0.05 mmol, as a catalyst) was added at room temperature. The mixture was refluxed for 48 h, and yellow solids were precipitated. The solid was isolated by sucking filtration, washed with MeOH, dried at 80 °C under vacuum for 12 h to yield PP5 as a yellow powder (0.18 g, 75% yield). IR (powder, KBr, cm−1) 3217 (N–H), 1679 (C
O), 1620 (C
N), 834 (Ar–H).
Synthesis of the model compound M1.
To a solution of pillar[5]arene 4 (0.21 g, 0.2 mmol) in ethanol (20 mL), benzaldehyde (45 μL, 0.44 mmol) and acetic acid (0.05 mmol, as a catalyst) was added at room temperature. The mixture was refluxed for 48 h, and white powder were precipitated. The solid was isolated by sucking filtration, washed with MeOH, dried at 80 °C under vacuum for 12 h to yield M1 as a white powder (0.21 g, 85% yield). 1H NMR (600 MHz, DMSO-d6) δ 11.47 (s, 1H), 11.37 (s, 1H), 8.18 (s, 1H), 7.97 (s, 1H), 7.65 (dd, J = 17.4, 6.0 Hz, 4H), 7.49–7.26 (m, 6H), 6.75 (s, 10H), 3.81 (s, 4H), 3.63 (s, 34H), 3.29 (s, 4H), 2.71 (d, J = 5.7 Hz, 4H), 1.80 (s, 8H).
Results and discussion
The polymer PP5 shown in Scheme 1 and the synthesis details are presented in Scheme S1.† The model compound M1 (as a soluble-molecule counterpart of PP5, structure shown in Scheme S2†) also been synthesized. Their intermediate and the model compound M1 have been characterized by 1H NMR, 13C NMR, and ESI-MS (Fig. S1–S11†). This polymer not only contains pillar[5]arene units as the fluorophore (signal transducer) but also embedded the thioacetohydrazone group as the ionophore (cation receptor). These unique characteristics are expected to be beneficial for the performance in selective detection and facile removal of Hg2+. PP5 also been characterized by IR (Fig. S10†).
With the robust hydrazone linkage in its structure, PP5 is insoluble and stable in common organic solvents, such as DMF, THF, DMSO, acetone, acetonitrile, ethanol and CHCl3. Importantly, PP5 is also insoluble and very stable in water. Thermogravimetric analysis (TGA) indicates that PP5 is thermally stable up to 285 °C (Fig. S12†). The scanning electron microscopy (SEM) images showed that PP5 possessed the irregular granular morphology (Fig. S13†). A characteristic vibrational band appeared at 1620 cm−1 in the FT-IR spectrum of PP5 (Fig. S10†), indicating the successful condensation of 1,4-phthalaldehyde and 4via the formation of –C
N– bonds. Meanwhile, we also investigated the crystallinity of this polymer in the solid state using powder XRD measurements (Fig. S14†). However, the peak of the polymer is the bread peak and significantly lower than compound 4, which indicates that PP5 is amorphous.
In order to investigate the luminescence properties of PP5, the insoluble polymer PP5 be dispersed in DMSO/H2O (1
:
1, v/v), and a series of host–guest recognition experiments were carried out. PP5 toward various cations (including Mg2+, Ca2+, Zn2+, Co2+, Fe3+, Pb2+, Hg2+, Cd2+, Ni2+, Tb3+, Cu2+, Eu3+, Fe2+, Cr3+, Ag+ and La3+) were primarily investigated using fluorescence spectroscopy. In the fluorescence spectrum, the maximum emission of PP5 appeared at 470 nm while excited at λex = 340 nm in DMSO/H2O (1
:
1, v/v). When 10.0 equiv. of Hg2+ was added to the dispersed solution of PP5, the fluorescence emission band was quenched (Fig. S15†). The same tests were applied using Mg2+, Ca2+, Zn2+, Co2+, Fe3+, Pb2+, Cd2+, Ni2+, Tb3+, Cu2+, Eu3+, Fe2+, Cr3+, Ag+ and La3+ cations, and only Hg2+ have significant changes in the fluorescence spectrum, and none of those other cations induced any significant changes in the fluorescent spectrum (Fig. 1).
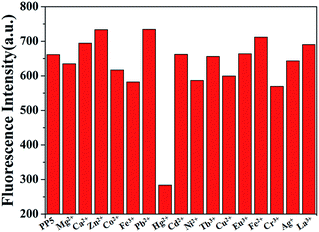 |
| Fig. 1 Fluorescence spectra responses for PP5 ([RU] = 4 × 10−5 M) and each of the various cations (4 × 10−4 M) in DMSO/H2O (1 : 1, v/v). | |
To further exploit the utility of the polymer PP5 as cation selective sensor for Hg2+, competitive experiments were carried out in the presence of 10.0 equiv. of Hg2+ and 10.0 equiv. of various cations in DMSO/H2O (1
:
1, v/v). The fluorescence selectivity was examined at an emission wavelength of 470 nm, neither of the competitive metal ions showed an appreciable influence on the Hg2+ detection (Fig. 2). These results further identified that PP5 exhibits a satisfactory selectivity toward Hg2+ detection.
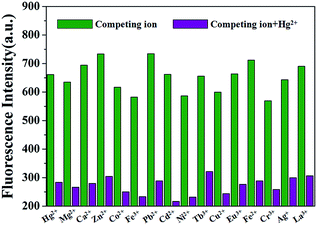 |
| Fig. 2 Fluorescence of PP5 ([RU] = 4 × 10−5 M) at 470 nm with addition of 10.0 equiv. of Hg2+ in the presence of 10.0 equiv. of other cations in DMSO/H2O (1 : 1, v/v). | |
The sensitivity of PP5 toward the Hg2+ detection was evaluated via the real-time fluorescence response. For this purpose, the stock solution of Hg(ClO4)2 was gradually added to the suspension of PP5 in DMSO/H2O (1
:
1, v/v), and the corresponding fluorescence spectra were measured immediately (Fig. 3). The detection limit of the fluorescent spectrum changes calculated on the basis of 3δ/S is 8.12 × 10−7 mol L−1 (Fig. S16†), indicating the high sensitivity of the sensor to Hg2+.
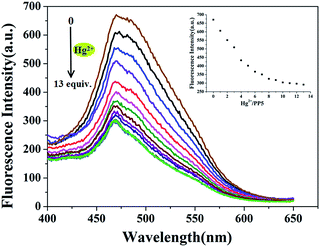 |
| Fig. 3 Fluorescence spectra of PP5 ([RU] = 4 × 10−5 M) in the presence of different concentration of Hg2+ in DMSO/H2O (1 : 1, v/v). Inset: A plot of emission at 470 nm versus number of equivalents of Hg2+. | |
To further illustrate the effective removal of Hg2+ from water, PP5 (8 mg) was suspended in a dilute aqueous solution of Hg(ClO4)2 (100 ppm in 10.0 mL). After the mixture was stirred at room temperature for 5 h. The inductively coupled plasma (ICP) analysis verified that the concentration of the residual Hg2+ in water was 3.75 ppm, that is, 96.25% of the mercury was removed by polymer PP5. Therefore, this polymer could effectively remove Hg2+ from water. In addition, the adsorption capacity of adsorbent have been calculated by the adsorption experiment data (Table S1†). The average value of adsorption capacity is 108 mg g−1 at room temperature.
On the basis of the excellent Hg2+ uptake capacity, we further explored the recycle use of PP5. Upon the simple treatment with 5 equiv. of aqueous Na2S solution to exchange the adsorbed Hg2+ out,19 the fluorescence of PP5 could be easily recovered. As shown in Fig. 4, this Hg2+ adsorption–desorption cycle could be repeated at least four times without significant loss of the sensitivity and responsiveness of PP5.
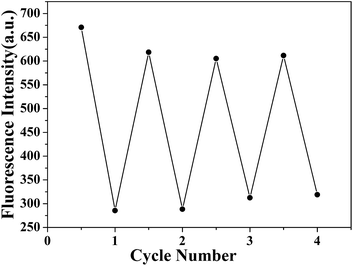 |
| Fig. 4 Recycle use of PP5 for selective detection and facile removal of the toxic Hg2+. Upon treatment in aqueous Na2S solution, PP5 was easily recovered and could be repeatedly used. | |
We used model compound M1 to study the possible mechanism by 1H NMR titration experiments because PP5 is difficult to be dissolved. Partial proton NMR spectra of M1 is shown in Fig. 5(a), and the signal assignments are depicted on the top. Upon the adsorption of Hg2+, H1 and H3 signals of M1 showed a obvious downfield shift (Fig. 5(b)). This result identified Hg2+ have the strong interaction with S and N atoms in Hg/M1. Meanwhile, after the treatment of Hg/M1 with Na2S, the 1H NMR spectrum recorded thereafter (Fig. 5(c)) closely resembles that of fresh M1. The results also illustrate the formation of complexes [HgS2]2−. Therefore, the selective detection and effective removal of Hg2+ for PP5 stems indeed from Hg2+ have the strong interaction with S and N atoms.
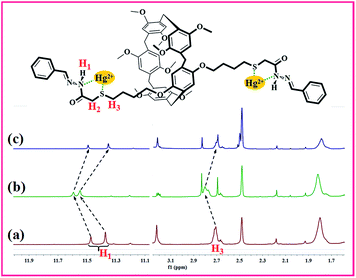 |
| Fig. 5
1H NMR spectra of M1 (a), Hg/M1 (b), and Hg/M1 after the treatment with Na2S (c). H1 and H3 signals of M1 was downfield shifted, which verified that mercury ions have a stronger coordination ability with S and N atoms. | |
The recognition mechanism of the polymer PP5 with Hg2+ was also investigated by IR spectroscopy. In the IR spectrum of PP5 (Fig. 6), the N–H bond show the stretching vibrations absorption peak at 3217 cm−1. However, after the addition of Hg2+, this peak shifts to 3448 cm−1. Meanwhile, the νC–S–C band at 955 cm−1 was also changed, which indicates that PP5 bonds to Hg2+via S and N atoms. In addition, the νC
O band at 1679 cm−1 and the νC
N band at 1620 cm−1 were almost unchanged, implying that Hg2+ does not bind to the –C
O bonds and –C
N bonds (instead, to the S atoms and the N atom of N–H groups) in PP5. According to powder XRD measurements (Fig. S14†), no clear new peaks were detected when mercury ions are added to the polymer PP5, indicating that the amorphous of the polymer is not destroyed. Therefore, the mercury ions should be coordinated with S and N atoms.
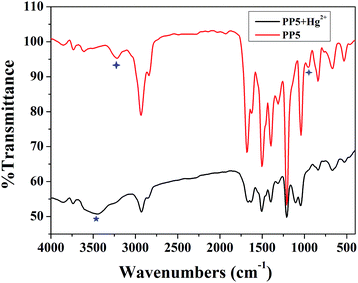 |
| Fig. 6 IR spectra of PP5 (red) and PP5–Hg2+ (black) in KBr disks. | |
Conclusions
In summary, a novel pillar[5]arene-based thioacetohydrazone functionalized fluorescent polymer has been synthesized, and it is used for fluorescence detection and removal of the toxic mercury ions. Meanwhile, this polymer exhibits high selectivity and sensitivity (8.12 × 10−7 M), and it can the efficient removal of Hg2+ from water. This research not only explored a new method for the synthesis of pillararene-based polymers but also expanded the pillararene applications about cation sensing/adsorption/removal. Thus, this good example might stimulate wide interest of scientists for further development of new pillararene-based polymers. We also expect that our research will help the environment and industry.
Conflicts of interest
There are no conflicts to declare.
Acknowledgements
This work was supported by the National Natural Science Foundation of China (NSFC) (No. 21662031; 21661028; 21574104; 21262032) and the Program for Changjiang Scholars and Innovative Research Team in University of Ministry of Education of China (IRT 15R56).
Notes and references
- R. P. Schwarzenbach, B. I. Escher, K. Fenner, T. B. Hofstetter, C. A. Johnson, U. von Gunten and B. Wehrli, Science, 2006, 313, 1072 CrossRef CAS.
-
(a) L. You, D. Zha and E. V. Anslyn, Chem. Rev., 2015, 115, 7840 CrossRef CAS;
(b) M. Vendrell, D. Zhai, J. C. Er and Y.-T. Chang, Chem. Rev., 2012, 112, 4391 CrossRef CAS PubMed;
(c) L. E. Kreno, K. Leong, O. K. Farha, M. Allendorf, R. P. Van Duyne and J. T. Hupp, Chem. Rev., 2012, 112, 1105 CrossRef CAS;
(d) J. H. Jung, J. H. Lee and S. Shinkai, Chem. Soc. Rev., 2011, 40, 4464 RSC;
(e) N. Dave, M. Y. Chan, P.-J. J. Huang, B. D. Smith and J. Liu, J. Am. Chem. Soc., 2010, 132, 12668 CrossRef CAS.
-
(a) K. P. Carter, A. M. Young and A. E. Palmer, Chem. Rev., 2014, 114, 4564 CrossRef CAS;
(b) X. Li, X. Gao, W. Shi and H. Ma, Chem. Rev., 2014, 114, 590 CrossRef CAS;
(c) H. N. Kim, W. X. Ren, J. S. Kim and J. Yoon, Chem. Soc. Rev., 2012, 41, 3210 RSC;
(d) J. Du, M. Hu, J. Fan and X. Peng, Chem. Soc. Rev., 2012, 41, 4511 RSC;
(e) D. T. Quang and J. S. Kim, Chem. Rev., 2010, 110, 6280 CrossRef CAS.
-
(a) Z. Wu and D. Zhao, Chem. Commun., 2011, 47, 3332 RSC;
(b) X. Feng, G. E. Fryxell, L.-Q. Wang, A. Y. Kim, J. Liu and K. M. Kemner, Science, 1997, 276, 923 CrossRef CAS.
-
(a) A. P. Esser-Kahn, A. T. Iavarone and M. B. Francis, J. Am. Chem. Soc., 2008, 130, 15820 CrossRef CAS;
(b) Y. Oh, C. D. Morris and M. G. Kanatzidis, J. Am. Chem. Soc., 2012, 134, 14604 CrossRef CAS.
- M. Khajeh, S. Laurent and K. Dastafkan, Chem. Rev., 2013, 113, 7728 CrossRef CAS.
-
(a) K.-K. Yee, N. Reimer, J. Liu, S.-Y. Cheng, S.-M. Yiu, J. Weber, N. Stock and Z. Xu, J. Am. Chem. Soc., 2013, 135, 7795 CrossRef CAS;
(b) C. W. Abney, J. C. Gilhula, K. Lu and W. Lin, Adv. Mater., 2014, 26, 7993 CrossRef CAS;
(c) Q.-R. Fang, D.-Q. Yuan, J. Sculley, J.-R. Li, Z.-B. Han and H.-C. Zhou, Inorg. Chem., 2010, 49, 11637 CrossRef CAS.
-
(a) T. Ogoshi, S. Kanai, S. Fujinami, T. Yamagishi and Y. Nakamoto, J. Am. Chem. Soc., 2008, 130, 5022 CrossRef CAS;
(b) D. Cao, Y. Kou, J. Liang, Z. Chen, L. Wang and H. Meier, Angew. Chem., Int. Ed., 2009, 48, 9721 CrossRef CAS;
(c) M. Xue, Y. Yang, X. Chi, Z. Zhang and F. Huang, Acc. Chem. Res., 2012, 45, 1294 CrossRef CAS;
(d) T. Ogoshi, T. Yamagishi and Y. Nakamoto, Chem. Rev., 2016, 116, 7937 CrossRef CAS.
-
(a) Z. Zhang, C. Han, G. Yu and F. Huang, Chem. Sci., 2012, 3, 3026 RSC;
(b) X.-B. Hu, L. Chen, W. Si, Y. Yu and J.-L. Hou, Chem. Commun., 2011, 47, 4694 RSC;
(c) N. L. Strutt, R. S. Forgan, J. M. Spruell, Y. Y. Botros and J. F. Stoddart, J. Am. Chem. Soc., 2011, 133, 5668 CrossRef CAS;
(d) X.-Y. Hu, X. Wu, Q. Duan, T. Xiao, C. Lin and L. Wang, Org. Lett., 2012, 14, 4826 CrossRef CAS;
(e) T.-B. Wei, J.-F. Chen, X.-B. Cheng, H. Li, B.-B. Han, H. Yao, Y.-M. Zhang and Q. Lin, Polym. Chem., 2017, 8, 2005 RSC;
(f) Y. Chen, M. He, B. Li, L. Wang, H. Meier and D. Cao, RSC Adv., 2013, 3, 21405 RSC;
(g) Y. Sun, W. Fu, C. Chen, J. Wang and Y. Yao, Chem. Commun., 2017, 53, 3725 RSC.
-
(a) W. Cheng, H. Tang, R. Wang, L. Wang, H. Meier and D. Cao, Chem. Commun., 2016, 52, 8075 RSC;
(b) Y. Cao, X. Hu, Y. Li, X. Zou, S. Xiong, C. Lin, Y. Shen and L. Wang, J. Am. Chem. Soc., 2014, 136, 10762 CrossRef CAS;
(c) X. Chi, X. Ji, D. Xia and F. Huang, J. Am. Chem. Soc., 2015, 137, 1440 CrossRef CAS;
(d) X. Wang, K. Han, J. Li, X. Jia and C. Li, Polym. Chem., 2013, 4, 3998 RSC;
(e) W.-B. Hu, W.-J. Hu, X.-L. Zhao, Y. A. Liu, J.-S. Li, B. Jiang and K. Wen, Chem. Commun., 2015, 51, 13882 RSC;
(f) K. Wang, C.-Y. Wang, Y. Zhang, S. X.-A. Zhang, B. Yang and Y.-W. Yang, Chem. Commun., 2014, 50, 9458 RSC.
-
(a) Y. Yao, X. Chi, Y. Zhou and F. Huang, Chem. Sci., 2014, 5, 2778 RSC;
(b) B. Shi, K. Jie, Y. Zhou, D. Xia and Y. Yao, Chem. Commun., 2015, 51, 4503 RSC;
(c) T.-B. Wei, J.-F. Chen, X.-B. Cheng, H. Li, B.-B. Han, Y.-M. Zhang, H. Yao and Q. Lin, Org. Chem. Front., 2017, 4, 210 RSC.
-
(a) F. Zhang, J. Ma, Y. Sun, I. Boussouar, D. Tian, H. Li and L. Jiang, Chem. Sci., 2016, 7, 3227 RSC;
(b) X. Cheng, H. Li, F. Zheng, Q. Lin, Y. Zhang, H. Yao and T. Wei, Dyes Pigm., 2016, 127, 59 CrossRef CAS;
(c) Y. Fang, X. Yuan, L. Wu, Z. Peng, W. Feng, N. Liu, D. Xu, S. Li, A. Sengupta, P. Mohapatra and L. Yuan, Chem. Commun., 2015, 51, 4263 RSC.
-
(a) P. Wei, D. Li, B. Shi, Q. Wang and F. Huang, Chem. Commun., 2015, 51, 15169 RSC;
(b) T.-B. Wei, X.-B. Cheng, H. Li, F. Zheng, Q. Lin, H. Yao and Y.-M. Zhang, RSC Adv., 2016, 6, 20987 RSC;
(c) T.-B. Wei, J.-F. Chen, X.-B. Cheng, H. Li, Q. Lin, H. Yao and Y.-M. Zhang, RSC Adv., 2016, 6, 65898 RSC;
(d) J.-F. Chen, X.-B. Cheng, H. Li, Q. Lin, H. Yao, Y.-M. Zhang and T.-B. Wei, New J. Chem., 2017, 41, 2148 RSC.
-
(a) A. Kraft, A. C. Grimsdale and A. B. Holmes, Angew. Chem., Int. Ed., 1998, 37, 402 CrossRef;
(b) U. H. F. Bunz, Chem. Rev., 2000, 100, 1605 CrossRef CAS.
-
(a) D. T. McQuade, A. E. Pullen and T. M. Swager, Chem. Rev., 2000, 100, 2537 CrossRef CAS;
(b) C. Zhu, L. Liu, Q. Yang, F. Lv and S. Wang, Chem. Rev., 2012, 112, 4687 CrossRef CAS;
(c) A. H. Hoang and M. Leclerc, J. Am. Chem. Soc., 2003, 125, 4412 CrossRef;
(d) M. Vetrichelvan, R. Nagarajan and S. Valiyaveettil, Macromolecules, 2006, 39, 8303 CrossRef CAS.
-
(a) X. Gong, M. R. Robinson, J. C. Ostrowski, D. Moses, G. C. Bazan and A. J. Heeger, Adv. Mater., 2002, 14, 581 CrossRef CAS;
(b) G. Yu, J. Gao, J. C. Hummelen, F. Wudl and A. J. Heeger, Science, 1995, 270, 1789 CrossRef CAS.
-
(a) Y. Ma, L. Chen, C. Li and K. Müllen, Chem. Commun., 2016, 52, 6662 RSC;
(b) S. Sun, X.-Y. Hu, D. Chen, J. Shi, Y. Dong, C. Lin, Y. Pan and L. Wang, Polym. Chem., 2013, 4, 2224 RSC.
-
(a) Q. Lin, P.-P. Mao, L. Liu, J. Liu, Y.-M. Zhang, H. Yao and T.-B. Wei, RSC Adv., 2017, 7, 11206 RSC;
(b) J.-F. Chen, X. Liu, J.-F. Ma, B.-B. Han, J.-D. Ding, Q. Lin, H. Yao, Y.-M. Zhang and T.-B. Wei, Soft Matter, 2017, 13, 5214 RSC.
-
(a) D. Wu, W. Huang, Z. Lin, C. Duan, C. He, S. Wu and D. Wang, Inorg. Chem., 2008, 47, 7190 CrossRef CAS;
(b) Y. Zhou, C.-Y. Zhu, X.-S. Gao, X.-Y. You and C. Yao, Org. Lett., 2010, 12, 2566 CrossRef CAS PubMed;
(c) D. M. Findlay and R. A. N. McLean, Environ. Sci. Technol., 1981, 15, 1388 CrossRef CAS.
Footnote |
† Electronic supplementary information (ESI) available: 1H NMR date and other materials. See DOI: 10.1039/c7ra10326c |
|
This journal is © The Royal Society of Chemistry 2017 |