DOI:
10.1039/C7RA08607E
(Paper)
RSC Adv., 2017,
7, 43950-43956
Fluorescent triazolium for sensing fluoride anions in semi-aqueous solution†
Received
3rd August 2017
, Accepted 6th September 2017
First published on 12th September 2017
Abstract
A new pyrene-appended triazolium has been synthesized and explored as a highly efficient receptor for fluoride anions. In an CH3CN–H2O competitive solution, this probe shows an exclusive fluorescence turn-off sensing of F− over other anions. 1H NMR titrations and density functional theory (DFT) calculations revealed that the triazolium (CH)+, sulfonamide NH and pyrenyl CH donors were involved in hydrogen bonding interactions with a F− anion to form a stable 1–F− complex. In addition, the probe was successfully applied to the detection of F− from inorganic origins in the solid state.
Introduction
The development of chemosensors for the sensing and recognition of fluoride anions has been of growing interest for the past few decades due to its crucial role in biosystems.1–3 Fluoride is an essential element for the body. Appropriate fluoride ingestion can prevent dental cavities and osteofluorosis.4,5 However, chronic exposure to high levels of fluoride causes severe diseases such as dental and skeletal fluorosis, kidney and gastric disorders, and urolithiasis in humans.6,7 Therefore, sensitive and accurate detection of fluoride anions in environmental and biological system is in high demand.
Until now, many analytical methods including inductively coupled plasma, atomic emission spectrometry, atomic absorption spectroscopy, electrochemical methods, absorption and emission spectroscopy have been reported for detection of fluoride anion.8–12 Among them, the fluorescent technique has been attracted particular attention for its low-cost, high sensitivity and easy operation.13–17 Thus, many fluorescent fluoride probes based on different interaction mechanisms, including hydrogen bonding,18–23 Lewis acid complexation24–28 and desilylation reaction,29–33 have been developed. Owing to the smallest size and high hydration enthalpy (−505 kJ mol−1) of fluoride anion in aqueous solution,34 the complexation and sensing of fluoride anion in aqueous media is mainly limited in the fluoride anion-induced chemical reactions systems. Hydrogen bonding type sensors are only capable of sensing F− in pure organic solvent, or in the presence of a small amount of water, which often suffer interference from alkaline anions such as AcO− and H2PO4−.35–38 In addition, most of these probes can detect tetrabutylammonium fluoride (TBAF) rather than inorganic fluoride such as KF.39,40
Recently, the click derived triazolium has attracted much attention in anions supramolecular chemistry because of its specific (C–H)+⋯ anion hydrogen bond interaction.41–43 Several groups, including ours, have used triazolium as a platform for anions recognition.44–51 Thus, a series of triazolium-based probe for fluorescence sensing of F−, H2PO4− and other anions have been well established in organic solution. However, systems for F− recognition with high selectivity in aqueous solution were still rare. Herein, we report a new fluorescence triazolium 1 (Scheme 1) for anions recognition. With the pyrene as the fluorescence signal moiety, the triazolium (CH)+ and sulfonamide NH as the hydrogen bonding donors, probe 1 shows an exclusive fluorescence turn-off sensing of inorganic fluoride anion KF in CH3CN–H2O (9
:
1, v/v) solution. The binding mechanism between probe 1 and F− was investigated in detail by various spectral techniques (UV-vis, fluorescence and 1H NMR) and DFT calculations.
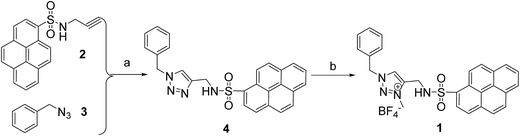 |
| Scheme 1 Synthesis route of 1. (a) CuSO4·5H2O, NaVc, DMF, 2 h, rt. (b) (Me3O)BF4, DCM, rt, 3 days. | |
Results and discussion
The synthetic route of the target probe 1 was shown in Scheme 1. The precursors 1-N-(2-propynyl)pyrene sulfonamide (2) and benzyl azide (3) were first prepared by the literature methods.50,52 Click reaction compounds 2 and 3, the neutral triazole 4 was then synthesized. Alkylation of 4 with (MeO)3BF4 in CH2Cl2 solution obtained the triazolium probe 1 with high yield (82% yield). The chemical structure of 1 was fully characterized by 1H NMR, 13C NMR, TOF-ESI-MS and the X-ray single crystal analysis.
The single crystals of 1 were obtained by slow diffusion hexane into its dichloromethane solution. In the X-ray crystal structure of 1 (Fig. 1), the whole structure of the probe adopts a fold conformation. The pyrene ring is roughly parallel to (4.3(2)°) the triazolium ring, but is nearly vertical (71.4(3)°) to the benzene ring. The two anions binding sites, sulfonamid NH and triazolium (CH)+, show an anti conformation in the solid state of 1, and are involved in hydrogen bonding with the BF4− counter anions (C9–H9⋯F3, 2.265 Å, 178.2°; N4–H4⋯F4, 2.452 Å, 134.2°, symm code: 2 − x, 1 − y, 1 − z). In addition, π–π interaction (3.435 Å) between the intermolecular pyrene rings is found in the crystal packing structure (Fig. S1†), which is further stabilized the X-ray structure of 1 in the solid state.
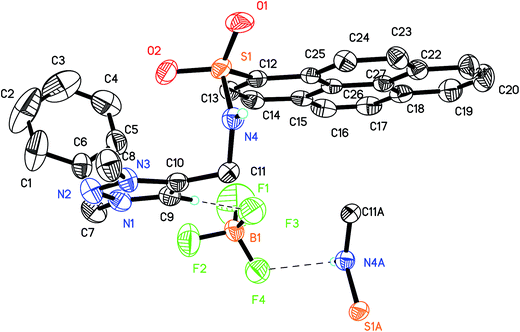 |
| Fig. 1 X-ray structure of 1. All the hydrogen atoms except sulfonamide NH and triazolium (CH)+ were omitted for clarity. | |
The recognition ability of 1 toward various anions (F−, Cl−, Br−, I−, AcO−, H2PO4−, CN−, NO3−) in their potassium salts was firstly investigated by emission spectra in CH3CN–H2O (9
:
1, v/v) solution. With excitation at 360 nm, probe 1 showed a strong structured pyrene-based monomer emission at 382 nm (Fig. 2). Upon the addition of F− (6 equiv.), the emission of probe 1 exhibited a large quenching effect (90% quenching), which can be easily observed from strong blue emission to dark quenching by the naked eyes. The large emission changes of 1 upon addition F− may be attributed to the strong hydrogen bonding interaction between 1 and F−. Thus, the forming 1–F− complex enhances photoinduced electron transfer quenching (PET) from the electron rich F− anion to the excited state of the pyrene fluorophore.53,54 In contrast, the addition of other anions induced the emission of 1 barely changes, including the basic AcO− and H2PO4− anions, which often show interference of the F− probes. Thus, probe 1 can be used as a fluorescence sensor for F− in semi-aqueous solution.
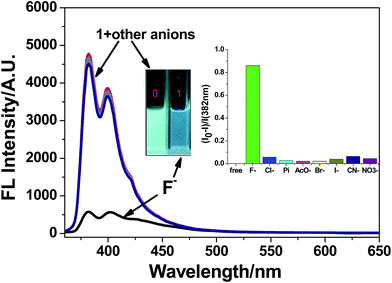 |
| Fig. 2 Fluorescence spectra of 1 (2.0 × 10−5 M) before and after addition 6 equiv. of anions (the form of potassium salts)in CH3CN–H2O (9 : 1, v/v) solution, λexi = 360 nm. Inset: the bar graph of 1 at 382 nm upon addition of anions. | |
Fig. 3 shows the fluorescence spectra changes of 1 with the incremental addition of KF. With increasing the concentration of F−, the pyrene-based emission of 1 at 382 nm gradually decreases, and was saturated when about 6.0 equivalence of F− was added. The binding data between 1 and F− was investigated by the emission titration data. A 1
:
1 binding stoichiometry of the 1–F− complex was determined from the Job's plot (Fig. S2†). Using the Benesi–Hildebrand equation,55 the binding constant Ka of the 1–F− was calculated to be 1.6 × 103 M (R = 0.998, Fig. S3†). Importantly, the fluorescence intensity of 1 at 382 nm versus the concentrations of F− was in a linear manner with the concentration range from 0 μM to 12 μM (Fig. S4†). A detection limit of 39 nM for F− was estimated for 1, which is much lower than the maximum limit of fluoride ion in drinking water (1.5 ppm, 79 μM) suggested by the World Health Organization (WHO).56
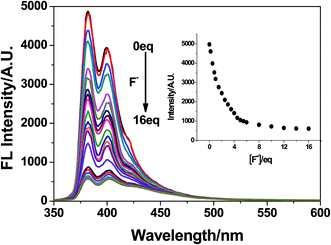 |
| Fig. 3 Fluorescence spectra of 1 (2.0 × 10−5 M, λex = 360 nm) upon the addition of F− in CH3CN–H2O (9 : 1, v/v) solution. Inset: fluorescence titration curve of 1 at 382 nm versus the equivalence of F−. | |
The UV-vis spectra changes of 1 before and after addition of anions were also investigated. Probe 1 shows typical pyrene-based bands at 348 nm, 357 nm and 378 nm. Upon the addition of F−, the intensity of these bands decreases with a ∼2 nm blue shift (Fig. 4), indicating a strong interaction between 1 and F−. Four clear isosbestic points at 331 nm, 363 nm, 376 nm and 380 nm were observed upon addition of F−, which imply that only one complex species forms during the complexation. In contrast, the addition of other anions induced no UV-vis spectra changes (Fig. S5†), even with a large amount. These results are well in accordance with the emission titration results.
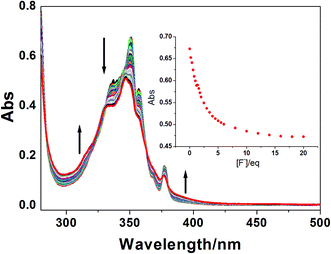 |
| Fig. 4 UV-vis spectra of 1 (2.0 × 10−5 M) upon addition various amount of F− in CH3CN–H2O (9 : 1, v/v) solution. Inset: UV-vis titration curve of 1 upon the addition of F− at 349 nm. | |
The exclusive UV-vis and emission spectra response of 1 toward the strong basic anion F− may be attributed to the existing multiple hydrogen bonding between 1 and F−. Therefore, the 1H NMR titration experiments of 1 with progressive addition of F− were carried out both in CD3CN and CD3CN–D2O (9
:
1, v/v) solutions (Fig. 5). For solubility problem, TBAF was used instead of KF here. In CD3CN solution, the two typical anion binding donors, triazolium proton Ha and sulfonamide proton Hb in 1, were located in 7.03 ppm and 6.49 ppm, respectively. Upon the addition of F−, the triazolium (CH)+ proton leaded about a 0.13 ppm down-filed shift (from 7.03 ppm to 7.16 ppm, when 4.0 equiv. F− was added), while the sulfonamide proton disappeared quickly, implying strong hydrogen bonding between these two donors and F− anion. Interesting, a large down-filed shift was also observed in the α-position proton of the pyrene ring (Hc, from 8.89 ppm to 9.19 ppm, Δδ = 0.30 ppm), indicating that this CH proton may also be an additional anion binding donor for the electron withdrawing group sulfonamide. Neutral CH⋯anion hydrogen bond was often observed in the aryl CH group when electron withdrawing substituents were present.57
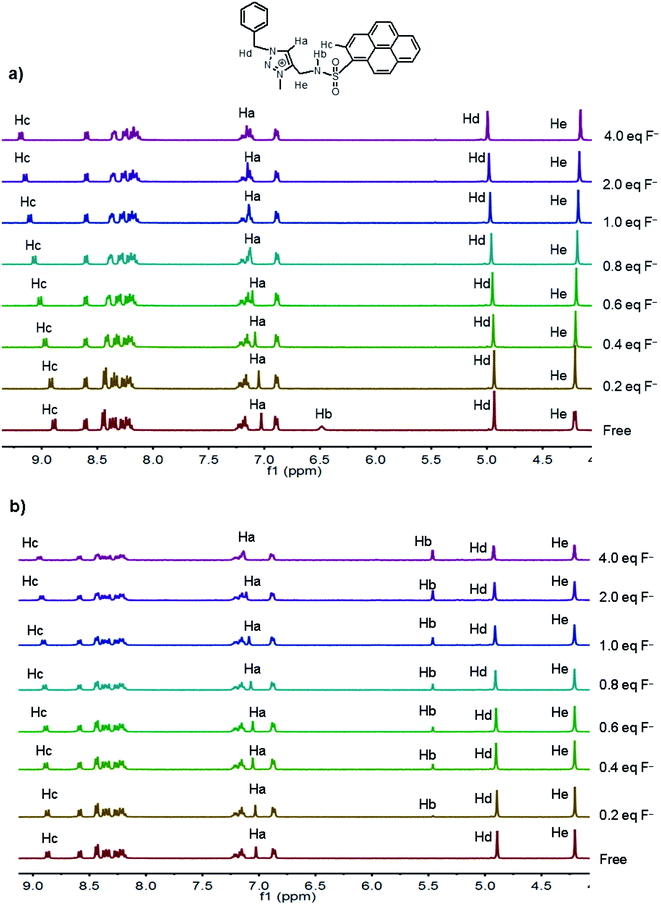 |
| Fig. 5 1H NMR spectra of 1 (4.0 mM) before and after addition various amount of TBAF (0–4.0 equiv.) in CD3CN (a) and CD3CN–D2O (9 : 1, v/v) (b) solutions. | |
The 1H NMR titrations of 1 to F− in CD3CN–D2O (9
:
1, v/v) solution were a little different from that in pure CD3CN solution. The sulfonamide proton Hb of free probe 1 disappeared for the deuterium exchange with D2O. Upon the addition of F−, this proton was observed at 5.46 ppm and increased with increasing the concentration of F−. Simultaneously, an obvious down-filed shift was found in protons Ha (Δδ = 0.14 ppm) and Hc (Δδ = 0.06 ppm) with addition of F−. These results revealed that the three protons, NH, (CH)+ and pyrenyl CH donors, still show strong hydrogen bonding interaction with F− in this competitive solvent, as that in pure CD3CN solution.
The multiple hydrogen bonding interactions between 1 and F− were further confirmed by the DFT calculations. The optimized structure of 1–F− complex in gas phase is shown in Fig. 6. In the presence of F−, the sulfonamid and triazolium donors prefers a syn conformation rather than anti conformation by rotation to form an electropositive cavity for the anions. According to the suggested geometry cutoffs for D–H⋯A hydrogen bond definition,58,59 the sulfonamide proton, the triazolium proton, the α-position pyrene proton and even the α-position phenyl proton are involved hydrogen bonding chelating with F− anion, with the H⋯F− hydrogen bonding distance values of 1.53 Å, 1.54 Å, 1.95 Å and 2.28 Å, respectively. According to the H⋯F− bond distance, the anions binding ability of these donors follows the order, NH ∼ (CH)+ > pyrenyl CH > phenyl CH, which is in accordance with 1H NMR titration results. Thus, these multiple hydrogen bonding interactions make 1 tightly bind with F− anion, even in CD3CN–D2O competitive solvent condition.
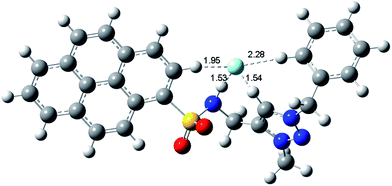 |
| Fig. 6 Calculated structure (B3LYP/6-31G*) of 1·F− complex. | |
The competition experiments were then carried out to investigate the selectivity and anti-interference of the probe 1 for F− anion detection. The fluorescence changes of 1 at 382 nm by adding various anions (Cl−, Br−, I−, NO3−, H2PO4−, CN− and AcO−) and then addition of F− in CH3CN–H2O (90
:
10 v/v) solution are shown in Fig. 7. There is no significant changes in the fluorescence spectra compared with that observed by the addition of F− alone, indicating that probe 1 exhibits excellent selectivity toward fluoride anion and good anti-interference ability against other coexisting anions.
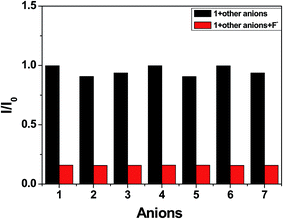 |
| Fig. 7 The fluorescence intensity of 1 at 382 nm upon addition 6.0 equivalence of other anions (black bar) and then addition F− (red bar) in CH3CN–H2O (90 : 10, v/v) solution. (1) Cl−, (2) H2PO4−, (3) AcO−, (4)Br−, (5)CN−, (6)I−, (7)NO3−. | |
Furthermore, the potential practical applicability of probe 1 in solid state for detection of F− was also investigated according to the method in the literature.60,61 A CH3CN solution of 1 (0.5 mM) was dropped onto the Whatman filter paper and dried in the air, a strong blue fluorescent spot was observed, indicating the probe has a good disperse in the filter paper matrix, and then suppress the aggregation-caused quenching (ACQ) of the probe. However, when a spot of F− solution was added to the test kits (Fig. 8a), the strong blue spot change to the dark quenching. However, the addition of other competitive anions leads no response of the fluorescence. In addition, a thin layer chromatography (TLC) plate experiment also investigated by the literature methods (Fig. 8b). The same fluorescence response from strong blue emission to dark quenching was also observed upon interaction with F−. Thus, probe 1 can detect F− in solid state not only by solution-coated TLC, but also using paper strips.
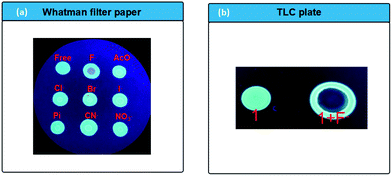 |
| Fig. 8 (a) Photographic image of anion (10−2 M) detection by 1 on filter paper tested by simple drop and dry from solutions of 1 and anions, consecutively. The sample was irradiated by UV-light (wavelength 365 nm). (b) Fluorescence image (under 365 nm UV light) of probe 1 adsorbed on a TLC plate with a spot of KF solution on probe 1. | |
Conclusion
In conclusion, we have been successfully designed and synthesized a pyrene based anion receptor 1 bearing triazolium and sulfonamide donors. Probe 1 shows fluorescence turn-off sensing of inorganic F− (KF) in CH3CN–H2O competitive solution, and can detect it at the parts per million level. The 1H NMR titration experiments proposed that the triazolium (CH)+, the sulfonamide NH and even the pyrenyl CH donors play an important role to form a stable 1
:
1 stoichiometry 1–F− complex, which is further confirmed by the DFT calculations. Furthermore, this probe was successfully applied as a solid state optical sensor for detection of F−. Considering the good selectivity and sensitivity of probe 1 for the detection of F− in aqueous system, these novel findings will give important information for designing more sophisticated sensors for fluoride anion.
Experimental section
General instrumentations and reagents
All the starting materials for synthesis were commercially available and used as received. The solvents used for UV-vis and emission measurements were purified by standard procedures. 1-N-(2-Propynyl)pyrene sulfonamide (2) and benzyl azide (3) were prepared by the literature method.50,52
UV-vis spectra were recorded on a Hitachi UV-3010 spectrophotometer. Emission spectra were recorded on a Hitachi F-4500 spectrophotometer. The excitation and emission slit width (5 nm) and the scan rate (250 mV s−1) were kept constant for all of the experiments. NMR spectra were recorded using a Varian instrument (400 MHz) in DMSO-d6 (Brucker, Switzerland; 1H NMR 400 MHz, 13C NMR 101 MHz). HR MS were recorded using LC/MSD TRAP XCT Plus (1200 Agilent). The anions are potassium salts.
Synthesis of 4
A mixture of 1-N-(2-propynyl)pyrene sulfonamide 2 (127 mg, 0.45 mmol), benzyl azide 3 (67 mg, 0.5 mmol), CuSO4·5H2O (25 mg, 0.1 mmol) and sodium ascorbate (40 mg, 0.2 mmol) were dissolved in 4 mL DMF solution. The mixture was stirred at room temperature for 4 h under nitrogen atmosphere. Then 30 mL H2O was added to get the precipitate. The solid was filtered, and purified by silica gel column chromatography using CH2Cl2
:
CH3OH (95
:
5, v/v) as the eluent to get 4 as a white powder (176 mg, yield 87%). 1H NMR (400 MHz, DMSO-d6, δ ppm): 8.93 (s, 1H), 8.64 (s, 1H), 8.58–8.17 (m, 8H), 7.64 (d, J = 9.1 Hz, 1H), 7.21 (s, 3H), 7.02 (s, 2H), 5.24 (d, J = 9.2 Hz, 2H), 4.5 (s, 2H), 4.10 (s, 2H). 13C NMR (101 MHz, CDCl3/CD3OD, δ ppm): 134.0, 133.7, 132.6, 132.4, 131.6, 130.8, 126.8, 57.47, 41.82. HR-MS (APCI) m/z: calcd for C26H20N4O2S: 453.1384; found 453.1379.
Synthesis of 1
Under a nitrogen atmosphere, 275 mg (0.5 mmol) 4 and 300 mg (2.0 mmol) (Me3O)BF4 was dissolved in 10 mL CH2Cl2 and stirred at room temperature for three days. Then 2 mL MeOH was added to quench the reaction. The organic solvent was removed under a vacuum pressure. The solid residue was then purified by silica gel column chromatography (CH3OH
:
CH2Cl2 = 1
:
9, v/v) to give 1 as a yellow solid (171 mg, 82% yield). 1H NMR (400 MHz, DMSO-d6, δ ppm): 8.93 (s, 1H), 8.64 (s, 1H), 8.58–8.17 (m, 8H), 7.64 (d, J = 9.1 Hz, 1H), 7.21 (s, 3H), 7.04 (s, 1H), 7.02 (s, 2H), 5.24 (d, J = 9.2 Hz, 2H), 4.5 (s, 2H), 4.10 (s, 2H). 13C NMR (101 MHz, DMSO-d6, δ ppm): 144.1, 136.1, 134.3, 132.9, 130.9, 130.4, 129.8, 129.0, 128.4, 128.2, 127.6, 127.5, 127.3, 127.2, 127.1, 52.9, 38.3. HR-MS (APCI): m/z: calcd for [C27H23N4O2S]+: 467.1542; found 467.1527.
General UV-vis and emission spectra measurements in solution
Stock solutions of anions in their potassium salts (0.1 M) were prepared in aqueous solution. Stock solution of receptor 1 (0.1 M) was prepared in CH3CN solution. The concentration of 1 in the UV-vis and emission titrations was 2.0 × 10−5 M in CH3CN–H2O (9
:
1, v/v) solution. During the titration, the anions stock solutions were added into a solution of 1 by a micro injector. The whole volume of the final system can be considered constant because the volume of anions solution added is negligible compared to that of receptor's solution.
Calculation of the association constant and the detection limit (LOD)
The binding constant of 1–F− was obtained from the fluorescence titration data using the Benesi–Hildebrand equation:
where I0 is the intensity of fluorescence of 1 without F−, I is the intensity with a particular concentration of F−, I′ is the intensity of the fully complexed form at the highest concentration of F−, and K is the binding constant.
The LOD was calculated using the formula 3δ/k using the emission titration data, where δ was the standard deviation of blank (10 samples) and k was the slope between intensity difference versus sample concentration.
Detection of F− on solid state under UV light
The CH3CN solution of 1 (0.5 mM) was first dropped to a piece of Whatman filter paper, and dried at room temperature for 4 h. The obtained fluorescence spots was then dropped anions (0.5 mM in aqueous solution) using a micropipette. After drying the strips under vacuum, the results were observed with 365 nm.
Thin-layer chromatography (TLC, spectrochem GF254 silica gel-coated plates) photo was also obtained by the similar method of Whatman filter paper.
Calculation method
Geometries of 1 and 1–F− complexation were optimized by DFT (B3LYP) method. A 6-31G(d, p) was selected for C, H, O, S and N atoms. No imaginary frequencies were available after vibration analysis of the optimized structures, which implied that the optimized structure was at the real minimum on the potential energy surfaces (PES). All the calculations were performed using Gaussian 09 program package.
Crystallography
Single-crystal X-ray diffraction measurements were collected on a Brucker CCD diffractometer using a graphite amonochromated Mo Kα radiation (λ = 0.71069 Å) at 296(2) K. Intensity data were collected in the variable ω-scan mode. The structures were solved by direct methods and refined on F2 by using full-matrix least-squares methods with the SHELXL-97 program package. All non-hydrogen atoms were located in successive difference Fourier syntheses and refined with anisotropic thermal factors. The hydrogen atoms were introduced geometrically.
Conflicts of interest
There are no conflicts to declare.
Acknowledgements
This work was supported by the National Nature Science Foundation of China (no. 21462027 and 21762028).
References
- Y. Zhou, J. F. Zhang and J. Yoon, Chem. Rev., 2014, 114, 5511–5571 CrossRef CAS PubMed.
- M. Cametti and K. Rissanen, Chem. Soc. Rev., 2013, 42, 2016–2038 RSC.
- N. Busschaert, C. Caltagirone, R. W. Van and P. A. Gale, Chem. Rev., 2015, 115, 8038–8155 CrossRef CAS PubMed.
- J. R. Farley, J. E. Wergedal and D. J. Baylink, Science, 1983, 222, 330–332 CAS.
- M. Kleerekoper, Endocrinol. Metab. Clin. North Am., 1998, 27, 441–452 CAS.
- P. P. Singh, M. K. Barjatiya, S. Dhing, R. Bhatnagar, S. Kothari and V. Dhar, Urol. Res., 2001, 29, 238–244 CrossRef CAS PubMed.
- K. L. Kirk, Biochemistry of the elemental halogens and inorganic halides, NewYork Plenum Press, 1991 Search PubMed.
- M. M. Bayón, A. R. Garcia, A. J. I. García and S. M. Alfredo, Analyst, 1999, 124, 27–31 RSC.
- G. Heike, W. Bernhard and W. E. Jürgen, Spectrochim. Acta, Part B, 2010, 65, 864–869 CrossRef.
- V. Sunitha and B. M. Reddy, Int. J. Curr. Res. Acad. Rev., 2014, 2, 159–166 CAS.
- A. M. Bond and T. A. O'Donnell, Anal. Chem., 1968, 40, 560–563 CrossRef CAS.
- U. Nina, Anal. Chim. Acta, 2000, 417, 201–209 CrossRef.
- L. E. Santos-Figueroa, M. E. Moragues, E. Climent, A. Agostini, R. Martínez-Máñez and F. Sancenón, Chem. Soc. Rev., 2013, 42, 3489–3513 RSC.
- X. Li, X. Gao, W. Shi and H. Ma, Chem. Rev., 2014, 114, 590–659 CrossRef CAS PubMed.
- L. Gai, J. Mack, H. Lu, T. Nyokong, Z. Li, N. Kobayashi and Z. Shen, Coord. Chem. Rev., 2015, 285, 24–51 CrossRef CAS.
- P. A. Gale and C. Caltagirone, Coord. Chem. Rev., 2017 DOI:10.1016/j.ccr.2017.05.003.
- J. Wu, B. Kwon, W. Liu, E. V. Anslyn, P. Wang and J. S. Kim, Chem. Rev., 2015, 115, 7893–7943 CrossRef CAS PubMed.
- P. Ashokkumar, H. Weihoff, W. Kraus and K. Rurack, Angew. Chem., Int. Ed., 2014, 53, 2225–2229 CrossRef CAS PubMed.
- Q.-Y. Cao, M. Li, L. Zhou and Z.-W. Wang, RSC Adv., 2014, 4, 4041–4046 RSC.
- V. Amendola, G. Bergamaschi, M. Boiocchi, L. Fabbrizzi and L. Mosca, J. Am. Chem. Soc., 2013, 135, 6345–6355 CrossRef CAS PubMed.
- S. Biswas, M. Gangopadhyay, S. Barman, J. Sarkar and N. P. Singh, Sens. Actuators, B, 2016, 222, 823–828 CrossRef CAS.
- J. Liu, X. He, J. Zhang, T. He, L. Huang, J. Shen and S. Yin, Sens. Actuators, B, 2015, 208, 538–545 CrossRef CAS.
- X. Zheng, W. Zhu, D. Liu, H. Ai, Y. Huang and Z. Lu, ACS Appl. Mater. Interfaces, 2014, 6, 7996–8000 CAS.
- F. Cheng, E. M. Bonder and F. Jakle, J. Am. Chem. Soc., 2013, 135, 17286–17289 CrossRef CAS PubMed.
- S. Madhu and M. Ravikanth, Inorg. Chem., 2014, 53, 1646–1653 CrossRef CAS PubMed.
- M. Hirai and F. P. Gabbaï, Chem. Sci., 2014, 5, 1886–1893 RSC.
- I. S. Ke, M. Myahkostupov, F. N. Castellano and F. P. Gabbaï, J. Am. Chem. Soc., 2012, 134, 15309–15311 CrossRef CAS PubMed.
- C. R. Wade, A. E. J. Broomsgrove, S. Aldridge and F. P. Gabbaï, Chem. Rev., 2010, 110, 3958–3984 CrossRef CAS PubMed.
- K. Dhanunjayarao, V. Mukundam and K. Venkatasubbaiah, Sens. Actuators, B, 2016, 232, 175–180 CrossRef CAS.
- D. Kim, S. Singha, T. Wang, E. Seo, J. H. Lee, S. J. Lee and K. H. Ahn, Chem. Commun., 2012, 48, 10243–10245 RSC.
- M. E. Jun, B. Roy and K. H. Ahn, Chem. Commun., 2011, 47, 7583–7601 RSC.
- L. Li, Y. Ji and X. Tang, Anal. Chem., 2014, 86, 10006–10009 CrossRef CAS PubMed.
- X. Chen, T. Leng, C. Wang, Y. Shen and W. Zhu, Dyes Pigm., 2017, 141, 299–305 CrossRef CAS.
- J. L. Sessler, P. A. Gale and W. S. Cho, Anion Receptor Chemistry, RSC Publishing, 2006 Search PubMed.
- V. B. Bregović, N. Basarić and K. Mlinarić-Majersk, Coord. Chem. Rev., 2015, 295, 80–124 CrossRef.
- Y.-L. Xu, C.-T. Li, Q.-Y. Cao, B.-Y. Wang and Y. Xie, Dyes Pigm., 2017, 139, 681–687 CrossRef CAS.
- N. Kumari, S. Jha and S. Bhattacharya, J. Org. Chem., 2011, 76, 8215–8222 CrossRef CAS PubMed.
- L. Zhou, X. T. Fan, Y. D. Xu and Q.-Y. Cao, New J. Chem., 2015, 39, 8087–8092 RSC.
- G. Sivaraman and D. Chellappa, J. Mater. Chem. B, 2013, 1, 5768–5772 RSC.
- T. Anand, G. Sivaramana, M. Iniya, A. Siva and D. Chellappa, Anal. Chim. Acta, 2015, 876, 1–8 CrossRef CAS PubMed.
- B. Schulze and U. S. Schubert, Chem. Soc. Rev., 2014, 43, 2522–2571 RSC.
- R. Tepper, B. Schulze, M. Jäger, C. Friebe, D. H. Scharf, H. Görls and U. S. Schubert, J. Org. Chem., 2015, 80, 3139–3150 CrossRef CAS PubMed.
- Y. Hua and A. H. Flood, Chem. Soc. Rev., 2010, 39, 1262–1271 RSC.
- B. Sui, B. Kim, Y. Zhang, A. Frazer and K. D. Belfield, ACS Appl. Mater. Interfaces, 2013, 5, 2920–2923 CAS.
- R. K. Chhatra, A. Kumar and P. S. Pandey, J. Org. Chem., 2011, 76, 9086–9089 CrossRef PubMed.
- B. Schulze, C. Friebe, M. D. Hager, W. Günther, U. Köhn, B. O. Jahn and U. S. Schubert, Org. Lett., 2010, 12, 2710–2713 CrossRef CAS PubMed.
- A. Caballero, F. Zapata, L. González, P. Molina, I. Alkorta and J. Elguero, Chem. Commun., 2014, 50, 4680–4682 RSC.
- Z.-C. Wang, Z. Wang, Q.-Y. Cao, S. Lin and Z.-W. Wang, ChemPlusChem, 2016, 81, 406–413 CrossRef CAS.
- Q.-Y. Cao, Y.-M. Han, P.-S. Yao, W. F. Fu, Y. Xie and J.-H. Liu, Tetrahedron Lett., 2014, 55, 248–251 CrossRef CAS.
- J.-Z. Ge, Z. Liu, Q.-Y. Cao, Y. Chen and J. H. Zhu, Chem.–Asian J., 2016, 11, 687–690 CrossRef CAS PubMed.
- Q.-Y. Cao, T. Pradhan, M. H. Lee, K. No and J. S. Kim, Analyst, 2012, 137, 4454–4457 RSC.
- S. B. Nallapati, B. Y. Sreenivas, R. Bankala, K. V. L. Parsa, S. Sripelly, K. Mukkanti and M. Pal, RSC Adv., 2015, 5, 94623–94628 RSC.
- R. M. Duke, E. B. Veale, F. M. Pfeffer, P. E. Kruger and T. Gunnlaugsson, Chem. Soc. Rev., 2010, 39, 3936–3953 RSC.
- T. Gunnlaugsson, H. D. P. Ali, M. Glynn, P. E. Kruger, G. M. Hussey, F. M. Pfeffer, C. M. G. dos Santos and J. Tierney, J. Fluoresc., 2005, 15, 287–299 CrossRef CAS PubMed.
- N. J. Turro, Modern molecular photochemistry, Benjamin Cummings Publishing Co. Inc., Menlo Park, CA, 1978 Search PubMed.
- H. T. Dean, F. A. Arnold Jr, P. Jay and J. W. Knutson, Public Health Rep., 1950, 1403 CrossRef CAS.
- J. J. Cai and J. L. Sessler, Chem. Soc. Rev., 2014, 43, 6198–6213 RSC.
- T. Steiner and G. R. Desiraju, Chem. Commun., 1998, 891–892 RSC.
- T. Steiner, Angew. Chem., Int. Ed., 2002, 41, 48–76 CrossRef CAS.
- Y. C. Wu, J. P. Huo, L. Cao, S. Ding, L. Y. Wang, D. Cao and Z. Y. Wang, Sens. Actuators, B, 2016, 237, 865–875 CrossRef CAS.
- J. F. Xiong, J. X. Li, G. Z. Mo, J. P. Huo, J. Y. Liu, X. Y. Chen and Z. Y. Wang, J. Org. Chem., 2014, 79, 11619–11630 CrossRef CAS PubMed.
Footnote |
† Electronic supplementary information (ESI) available. CCDC 1566968. For ESI and crystallographic data in CIF or other electronic format see DOI: 10.1039/c7ra08607e |
|
This journal is © The Royal Society of Chemistry 2017 |