DOI:
10.1039/C7RA08304A
(Paper)
RSC Adv., 2017,
7, 49194-49203
Peptide GEQQQQPGM derived from rice α-globulin reduces the risk of atherosclerosis in hamsters by improving vascular endothelial cells injury†
Received
27th July 2017
, Accepted 6th October 2017
First published on 23rd October 2017
Abstract
A previous study has confirmed that oral administration of rice α-globulin (100 mg per day per kg bodyweight) efficiently prevents atherosclerosis disease in male apolipoprotein E-deficient (ApoE−/−) mice. However, the key peptide in rice α-globulin after gastrointestinal digestion and absorption as well as its anti-atherosclerosis mechanism still remain unclear. We obtained the absorbable part in the simulated digestive products of rice α-globulin by everting the intestinal sacs followed by purification using gel filtration chromatography and high performance liquid chromatography. The peptide GEQQQQPGM was confirmed to be one of the key fragments in rice α-globulin by LC-MS/MS (liquid chromatography-mass spectrometry/mass spectrometry). We investigated the hypocholesterolemic effect of the peptide in hamsters fed a hypercholesterolemic diet. The synthetic peptide GEQQQQPGM was orally administrated to hamsters at a dose of 100 mg per kg bodyweight, and deionized water was orally administrated to the normal and control hamsters for 30 days. In the peptide group, plasma total and LDL cholesterol concentrations and atherogenic index were significantly lower than those in the control group. To investigate the anti-atherosclerosis mechanism of the peptide, the effects of the GEQQQQPGM peptide derived from rice α-globulin on vascular endothelial cells injury were studied. The cell model was established using 10 ng mL−1 of tumor necrosis factor-α (TNF-α). The study was performed on HUVECs that were pretreated with 50 μg mL−1 of GEQQQQPGM. The cell viability and indicators of inflammation and oxidative stress were measured. The decreased viability of the HUVECs induced by TNF-α was significantly increased by GEQQQQPGM. The peptide obviously reduced the levels of monocyte chemoattractant protein-1 (MCP-1), intercellular adhesion molecule-1 (ICAM-1), and vascular cell adhesion molecule-1 (VCAM-1). It also effectively affected the antioxidant indices such as it decreased the levels of nitric oxide (NO), inducible nitric oxide synthase (iNOS), malondialdehyde (MDA), and reactive oxygen species (ROS), whereas increased the activities of glutathione peroxidase (GSH-Px) and superoxide dismutase (SOD). Furthermore, GEQQQQPGM inhibited nuclear translocation and expression of p65 protein by decreasing the inhibitor of κB kinase α (IKKα) protein and up-regulating the inhibitor of nuclear factor κB kinase (IκB) protein. These results demonstrated that GEQQQQPGM reduced TNF-α-induced vascular endothelial cell injury by inhibiting the inflammatory response and oxidative stress, thereby reducing the risk of atherosclerosis.
1. Introduction
Vascular endothelial dysfunction is considered as an important risk factor for the development of atherosclerosis, which is significantly involved in the pathogenesis of cardiovascular diseases.1,2 Inflammatory response and oxidative stress are the major causes of reduced endothelial nitric oxide (NO) availability in injured vascular endothelial cells, which can be triggered by the tumor necrosis factor-α (TNF-α).3,4 TNF-α, as the most common pro-inflammatory cytokine in atherosclerosis, induces inflammation and oxidative stress, thereby regulating the expression of some cell molecules in vascular endothelial cells.5,6 Therefore, a TNF-α-induced cell injury model is widely used to investigate the protective effects of food functional components on vascular endothelial cells.
Numerous studies on experimental animals and humans have proven that the intake of food proteins, such as rice, soybean, and oat proteins, can significantly reduce the risk of atherosclerosis by lowering plasma lipids.7–9 Food proteins are digested into amino acids and peptides, and some of these peptides can be directly absorbed by the body such that to prevent lifestyle diseases such as atherosclerosis, hypertension, and hyperlipidemia.10,11
Matsui et al. reported that the oral administration of the dipeptide WH (10 or 100 mg per day per kg bodyweight) derived from soybean globulin inhibited progressive atherosclerotic lesions in ApoE−/− mice without any alteration of the lipid profiles.12 They further confirmed that WH improved atherosclerosis by inhibiting the phosphorylation of voltage-dependent L-type Ca2+ channels in rat vascular smooth muscle cells.13 Similarly, the tripeptide HGI and HGK derived from soybean glycinin have been reported to suppress the intracellular Ca2+ concentration in vascular smooth muscle cells.14 Huang et al. reported that the tripeptide IRW derived from egg protein reduced the TNF-α-induced inflammatory response and oxidative stress and reduced the levels of superoxide ions in human umbilical vein endothelial cells (HUVECs).6
Endothelial dysfunction in atherosclerosis generates reactive oxygen species (ROS) that can be detoxified by anti-oxidative enzymes.7 Moreover, pro-inflammatory nuclear factor κB (NF-κB) has been recognized as a major transcription factor that mediates the key steps in the development of atherosclerosis. The tripeptide IRW, as a signal transduction messenger, reduced the production of ROS by inhibiting the inflammatory activation of NF-κB.6
A previous study has confirmed that the oral administration of rice α-globulin (100 mg per day per kg bodyweight) efficiently reduces the en face lesion area in the aorta and the lesion size in the aortic root and thus prevents atherosclerosis disease in male apolipoprotein E-deficient (ApoE−/−) mice without any alteration of the plasma lipid levels.15 We speculate that there may be functional peptide fragments in rice α-globulin and these peptides play a role in protecting vascular cell injury. However, the key peptide in rice α-globulin after gastrointestinal digestion and absorption together with its anti-atherosclerosis mechanism still remains unclear.
2. Materials and methods
2.1. Materials
TNF-α, fetal calf serum (FBS), and penicillin-streptomycin antibiotics were purchased from Invitrogen-Gibco (USA). A protein quantitative kit (BCA) was purchased from Sainobo Co., Ltd. (Beijing, China). A cell counting kit-8 (CCK-8) was purchased from Dojindo Co. Ltd. (Shanghai, China). The assay kits for NO, iNOS, ROS, SOD, GSH-PX, and MDA were purchased from Beyotime Biotechnology Co., Ltd. (Shanghai, China).
2.2. Preparation of the peptide
The α-globulin digestion experiments were carried out using a pepsin trypsin sequential digestion in vitro digestion model. First, the protein was digested using pepsin in HCl at pH 2.0 and 37 °C for 2 h. The pH value was adjusted to 5.3 using a 0.09 mol L−1 NaHCO3 solution. After trypsin was added, the pH value was adjusted to 7.8 using a 1 mol L−1 NaOH solution. The sample was digested at 37 °C for 2 h. The sample was heated at 100 °C for 10 min to terminate the reaction. After centrifugation at 2000 × g for 20 min, the supernatant was obtained and frozen for storage.
The peptide absorption experiments were carried out using everted intestinal sacs in vitro. Male hamsters (four-week-old) were obtained from Vital River Lab Animal Technology Co, Ltd. (Beijing, China). The hamsters were fasted for 16 h and then anesthetized. After the small intestine was removed, it was washed with Krebs-Ringer solution (118.4 mmol L−1 NaCl, 4.7 mmol L−1 KCl, 1.2 mmol L−1 KH2PO4, 1.2 mmol L−1 MgSO4·7H2O, 2.5 mmol L−1 CaCl2·2H2O, 11.7 mmol L−1 C6H12O6, and 26.5 mmol L−1 NaHCO3) to remove the contents. The small intestine was everted with glass, and its lower part was tightened. Then, 2 mL of Krebs-Ringer solution was added to the small intestine, and it was placed in a test tube at 37 °C. The test tube contained Krebs-Ringer solution and was incorporated with a mixture of gases (95% O2 and 5% CO2, 2 mL min−1). After adding the protein digestive juice, the absorption experiments were carried out at 37 °C for 90 min and then the samples were taken from the test tubes.
The 30 mg mL−1 absorbed product was preliminarily separated by a glass chromatography column (Sephadex G-15: 1.6 × 50 cm, Bio-Rad, Hercules, CA, USA) using ultrapure water at a flow rate of 1 mL min−1. The elution peaks were obtained at 220 nm (UV detector) and freeze-dried. Next, the 20 mg mL−1 samples were purified using RP-HPLC (Agilent Prostar 218, Agilent Co., USA). The chromatographic conditions were as follows: Innoval C18 (21.2 × 250 mm, 10 μm); sample volume = 1 mL; flow rate = 5 mL min−1; and detection wavelength = 220 nm.
After this, the amino acid sequence of the peptides was determined by LC-mass spectrometry (MS)/MS (Agilent 1100, Agilent Co., USA; LCQ Decaxp, Thermo Co., USA). The LC-MS conditions were as follows: chromatographic column (Zorbax SB-C18: 2.1 × 150 mm); mobile phase A: water (0.1% TFA) and B: acetonitrile (0.1% TFA); gradient: 0–40 min, 5–95% B; 40–50 min; 95–5% B; injection volume = 20 μL; and flow rate = 0.2 mL min−1. The MS conditions were as follows: ESI spray voltage = 4.5 kV; capillary temperature = 300 °C; positive ion detection; MS/MS dynamic scanning was data-dependent scan; dynamic elimination times = 1 and 0.5 min; collision energy for MS 2 = 35%; and collision energy and scanning range: m/z 900–1400 and m/z 1400–2000, respectively.
The LC-MS/MS data were compared using the fragment ion calculator software. After entering the target amino acid sequence in rice α-globulin, the following parameters were set up: mass type and selected MONO; charge state = +1; and ion type = B and Y. The calculated results were compared via the two stage mass spectrometry, and the matching results were positive; otherwise, the peptide segment was not detected.
The peptides in α-globulin were prepared using solid-phase synthesis. The peptide was synthesized according to the sequence obtained from China Peptides Co. Ltd. (Shanghai, China), and its purity (>95%) was verified using LC-MS/MS.
2.3. Animals and diets
In this study, four-week-old male hamsters (bodyweight = 100 ± 3 g) were obtained from Vital River Lab Animal Technology Co, Ltd. (Beijing, China) and kept in cages in an air-conditioned room. The peptide and α-globulin were orally administrated to the hamsters at a dose of 5 mg in 1 mL of deionized water and 50 mg in 1 M NaCI, respectively, and deionized water was orally administrated to the control hamsters. The hamsters were fasted for 4 h and then sacrificed by removal of the whole blood from abdominal aorta. Acetonitrile (containing 0.1% formic acid) was added to 500 μL of plasma and allowed to stand for 30 min at 4 °C. The plasma was centrifuged at 8000 × g at 4 °C for 20 min, and then, supernatant was obtained (with filtration through a 0.45 μm membrane).
Male hamsters (four-week-old) were obtained from Vital River Lab Animal Technology Co, Ltd. (Beijing, China) and kept in cages in an air-conditioned room (temperature = 21–23 °C; humidity = 55 ± 5%; and 12 hours light/dark cycle). After 3 days of acclimation, the hamsters were divided into 3 groups (normal, control, and GEQQQQPGM) of 7 hamsters. The peptide was orally administrated to the hamsters at a dose of 100 mg per kg bodyweight, and deionized water was orally administrated to the normal and control hamsters at 10:00 each day for 30 days. The composition of the experimental diet in the normal group was prepared according to the AIN-93G (American Institute of Nutrition. 1993) standard diet. The composition of the experimental diet in the control and GEQQQQPGM groups was prepared according to AIN-93G with some modification. The composition (in g kg−1) of the diet was 200 casein, 397 corn starch, 70 soybean oil, 10 cholesterol, 50 lard, 2 bile salt, 38.486 sucrose, 50 cellulose, 3 DL-methionine, 132 maltodextrin-10, 0.014 t-butylhydroquinone, 2.5 choline bitartrate, 35 mineral mix, and 10 vitamin mix. The experimental diet was prepared by Beijing Nuokangyuan Biotechnology Co., Ltd. (Beijing, China). The food intake was noted and monitored every day to ensure the similar content of diet in all the groups. The bodyweight of the animals was obtained every three days. The hamsters were fasted for 16 h and then sacrificed by removal of the whole blood from the abdominal aorta. The plasma lipid concentration was analyzed using an automatic chemistry analyzer (Hitachi, Tokyo, Japan).
All experiments were carried out according to the P. R. China legislation and approved by the Bioethics Committee of the Institute of Medicinal Plant Development, Chinese Academy of Medical Sciences and Peking Union Medical College.
2.4. Cell culture
HUVECs (Lot: 63505670), endothelial cell growth Kit-BBE, and vascular cell basal medium were acquired from the ATCC (Manassas VA, USA). The medium containing 10% FBS, Kit-BBE, and penicillin–streptomycin (1
:
1000) were prepared according to the method of ATCC. The cells were cultured in a humidified atmosphere containing 5% CO2 at 37 °C. The cells were treated with 0.25% trypsin/1 mM EDTA after approximately 80% confluence. The HUVECs were serum-starved overnight prior to stimulation. The DMEM with 10% of FBS medium was used for the cell subcultures.
2.5. Analysis of the cell viability
The cell viability was analyzed using the CCK-8 kit. Cells were seeded in 96-well plates (1 × 104 per well, 100 μL per well) for the CCK-8 assay. The cells were pretreated with the peptide (25, 50, 100, and 200 μg mL−1) for 1 h. In the presence of the peptide, the cells were stimulated with or without TNF-α (10 ng mL−1) for 18 h. Then, the CCK-8 solution (10 μL per well) was added followed by incubation at 37 °C for 2 h. Since the absorbance is proportional to the number of viable cells, the cell viability has been calculated by the absorbance at 450 nm using a microplate reader (Micro Reader 4, Hyperion, USA).
2.6. ELISA analysis
HUVECs were plated at a density of 1 × 105 cells per mL in 6-well plates and pretreated with or without 50 μg mL−1 of peptide for 2 h before stimulation with 10 ng mL−1 of TNF-α. To determine the content of iNOS, the cells were digested and fragmented by repeating freeze-thaw three times (at −80 °C for 5 min and at 37 °C for 5 min). The GSH-PX and SOD activities in the cells were measured using an ELISA assay kit. The MDA content was measured using a commercially available kit.
2.7. Measurement of the NO production
Nitrite and nitrate as the stable products were converted from NO and used to determine the NO content in the HUVECs. The cells were plated at a density of 1 × 105 cells per mL in 6-well plates and pretreated with or without 50 μg mL−1 of peptide for 2 h before stimulation with 10 ng mL−1 of TNF-α. The nitrite and nitrate concentrations in the HUVECs were measured using Griess reagent. The cell supernatant was added to 96-wells (50 μL per well) followed by addition of 50 μL of Griess reagent I and II. The nitrite and nitrate concentrations were determined using a microplate reader at 540 nm, and the NO content was calculated.
2.8. Western blotting
After pre-incubation with GEQQQQPGM (50 μg mL−1) for 18 h, the cells (1 × 107 cells per mL) were lysed using RIPA buffer containing trypsin inhibitor, centrifuged at 8000 × g for 20 min, and quantified using the BCA protein assay. The proteins in the cell lysate were resolved on SDS-PAGE and transferred to the membranes. The target proteins were immunoblotted with primary antibodies and then secondary goat anti rabbit IgG HRP (H + L) antibodies conjugated to horseradish peroxidase. The antibody bindings were revealed using an ECL plus Western blotting detection reagent kit (GE Healthcare, Buckinghamshire, UK). The band density was measured by densitometry and quantified using the Image J software. MCP-1 antibody (#2027), ICAM-1 antibody (#4915), VCAM-1 (E1E8X) rabbit mAb (#13662), IKKα antibody (#2682), and IκB-α antibody (#4812) were used to detect the protein expression.
2.9. Measurement of ROS production
After pre-incubation with GEQQQQPGM (50 μg mL−1) for 18 h, the HUVECs were incubated in TNF-α (10 ng mL−1) for 2 h followed by incubation with DCFH-DA (20 μM) for 30 min. Then, the cells were observed and imaged using a fluorescence microscope (ECLIPSE TE-2000-S, Nikon Co., Japan). The mean fluorescence intensity was measured using the Image J software (n = 6).
2.10. Measurement of NF-κB activation and nuclear translocation
Cells were immunofluorescence stained to detect the main subunit of NF-κB, p65, such that to confirm whether NF-κB was activated or not. Confluent cell monolayers in 96-well plates were pretreated with 50 μg mL−1 peptide for 6 h prior to incubation with 10 ng mL−1 TNF-α for 1 h. The cells were fixed, permeabilized, and immunostained for p65 (anti-rabbit Cy3) and nucleus (DAPI). Using the fluorescence microscope, it was observed that NF-κB exhibited red fluorescence, whereas the nucleus exhibited blue fluorescence.
2.11. Statistical analysis
Data are expressed as the mean with the standard error (SE) and have been analyzed by Tukey–Kramer multiple comparison post hoc test for the animal experiment. In the cell experiment, all data are presented as the mean value ± standard deviation (SD). The data are expressed as the fold change over the untreated control. One-way analysis of variance with an appropriate post test was used for the determination of statistical significance. Statistical significance was defined as P < 0.05. All statistical analysis was performed using the SPSS software.
3. Results
3.1. Identification of the peptide in rice α-globulin after gastrointestinal digestion and absorption
The absorbable part in the simulated digestive products of rice α-globulin was obtained by everting intestinal sacs and purified by gel filtration chromatography and high performance liquid chromatography. After this, the amino acid sequence of the peptides was measured and compared with that of α-globulin to identify the specific sequence of rice α-globulin. As the result, GEQQQQPGM was confirmed to be one of the key fragments in rice α-globulin (Fig. 1). Moreover, further serum tests showed that after the α-globulin and peptide were administrated, the peptide GEQQQQPGM was able to enter the plasma (ESI 1,† m/z 1002.2).
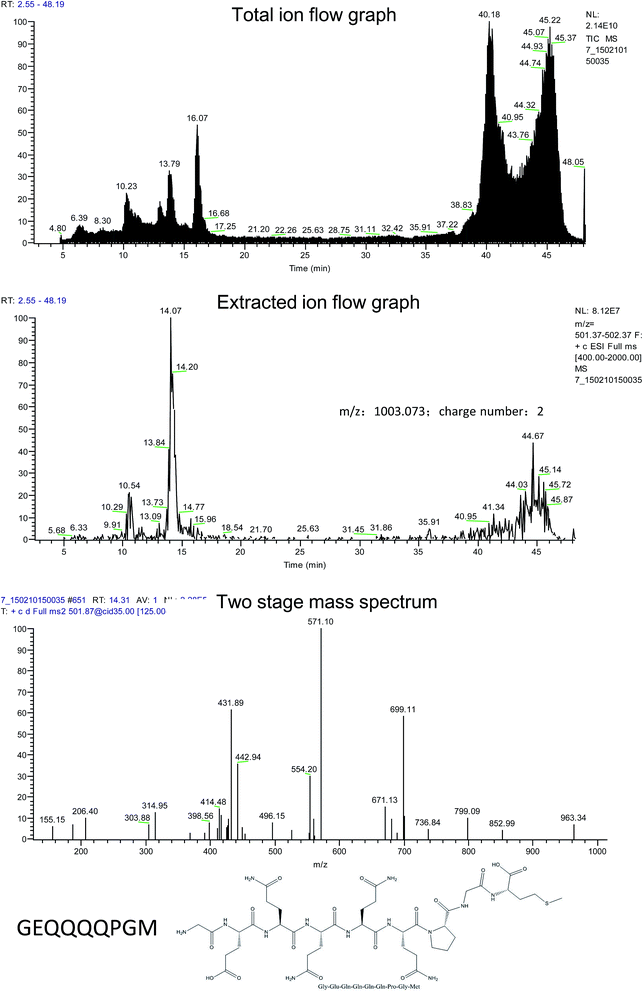 |
| Fig. 1 The amino acid sequence of the peptides was determined by LC-mass spectrometry (MS)/MS. | |
3.2. Metabolism-related indicators in hamsters
Metabolism-related indicators were measured in the 3 groups (Table 1). The initial bodyweight, bodyweight gain, food intake, liver weight, plasma HDL cholesterol, and triacylglycerol were not significantly different among the 3 groups. The hamsters orally administrated with GEQQQQPGM have significantly lower plasma total and LDL cholesterol concentrations than the control group (P < 0.05). Furthermore, the atherogenic index was reduced in the GEQQQQPGM group when compared with that in the control group (P < 0.05).
Table 1 The effects of oral administration of GEQQQQPGM on the metabolic parameters of hamsters fed with a hypercholesterolemic diet
|
Normal |
Control |
GEQQQQPGM |
The mean ± SD were determined from 7 hamsters per group. The different superscript letters a, b and c indicate the significant differences at P < 0.05 (Tukey–Kramer's multiple comparison post hoc test). |
Initial bodyweight (g) |
108.7 ± 2.4 |
108.6 ± 2.2 |
108.9 ± 2.3 |
Final bodyweight (g) |
121.1 ± 2.8 |
121.6 ± 2.7 |
121.7 ± 3.0 |
Bodyweight gain (g) |
12.4 ± 1.4 |
13.0 ± 1.5 |
12.8 ± 1.6 |
Food intake (g day−1) |
7.85 ± 0.22 |
7.88 ± 0.22 |
7.86 ± 0.20 |
Liver weight (g) |
6.02 ± 0.34 |
6.06 ± 0.29 |
6.05 ± 0.23 |
Plasma lipids (mmol L−1) |
|
|
|
Total cholesterol |
3.28 ± 0.23c |
6.85 ± 0.27a |
5.09 ± 0.25b |
LDL cholesterol |
1.47 ± 0.15c |
2.75 ± 0.14a |
1.98 ± 0.16b |
HDL cholesterol |
1.56 ± 0.17 |
1.62 ± 0.18 |
1.71 ± 0.14 |
Triglycerides |
2.01 ± 0.53 |
2.40 ± 0.64 |
2.47 ± 0.47 |
Atherogenic index |
2.28 ± 0.31c |
5.85 ± 0.33a |
4.09 ± 0.34b |
3.3. GEQQQQPGM increased cell viability in TNF-α-injured HUVECs
To evaluate whether the peptide GEQQQQPGM protects endothelial cells from TNF-α-induced injury, the HUVEC viability was measured (Fig. 2). GEQQQQPGM (200 μg mL−1) had no significant effect on the cell viability in normal HUVECs; however, the viability of the HUVECs was significantly reduced by the TNF-α treatment (69.8 ± 6.3%) when compared with that in the control (100%) (P < 0.01). The reduction of HUVEC viability induced by TNF-α was significantly increased by GEQQQQPGM at a dose of 50 μg mL−1 (103.7 ± 7.0%) (P < 0.01). The pretreatment with GEQQQQPGM obviously restored the cell viability in TNF-α-injured HUVECs, and the most effective dose was 50 and 100 μg mL−1 (103.7 ± 7.0% and 105.6 ± 6.1%) (P < 0.01).
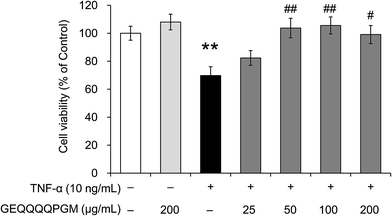 |
| Fig. 2 GEQQQQPGM increased the cell viability in TNF-α-injured HUVECs. Confluent HUVECs were pretreated with different concentrations of GEQQQQPGM for 1 h prior to incubation with 10 ng mL−1 TNF-α for 6 h. Cell apoptosis was analyzed using CCK-8 according to the standard operation instructions. The cell viability levels are expressed as the fold over the control. The bars represent the mean value ± SE, n = 6. * indicates P < 0.05, compared to the control; # and ## indicate P < 0.05 and P < 0.01, respectively, compared to TNF-α alone. | |
3.4. Regulation of MCP-1, VCAM-1, and ICAM-1 expression with GEQQQQPGM in TNF-α-injured HUVECs
The MCP-1 concentration was measured, and the results are shown in Fig. 3A. The peptide had no significant effect on the MCP-1 concentration in the HUVECs, whereas the TNF-α-injured HUVECs showed a significantly higher MCP-1 concentration than the control and GEQQQQPGM groups (P < 0.01). The HUVECs treated with both TNF-α and GEQQQQPGM showed significantly lower MCP-1 concentrations than the TNF-α-injured HUVECs (P < 0.01). As shown in Fig. 3B and C, the protein expression of VCAM-1 and ICAM-1 in the HUVECs were greatly increased by TNF-α treatment, whereas GEQQQQPGM suppressed the TNF-α-induced up-regulation significantly (P < 0.01). In addition, the peptide had no significant effect on the protein expression of VCAM-1 and ICAM-1 in the TNF-α-injured HUVECs.
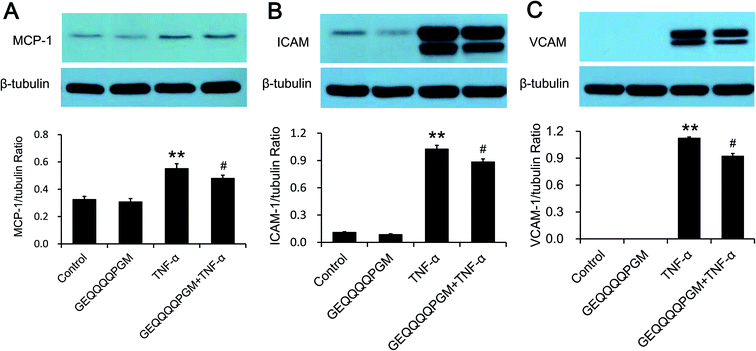 |
| Fig. 3 The regulation of VCAM-1, ICAM-1 and MCP-1 protein expression with GEQQQQPGM in TNF-α-injured HUVECs. Confluent HUVECs were pretreated with GEQQQQPGM (25 μg mL−1) for 18 h prior to incubation with 5 ng mL−1 TNF-α for 6 h. The protein expressions of MCP-1 (A), ICAM-1 (B) and VCAM-1 (C) were measured by Western blot analysis. The bars represent the mean value ± SE, n = 6. Representative Western blots are shown. ** indicates P < 0.01; # indicates P < 0.05, compared to the control. | |
3.5. Regulation of the NO and iNOS concentrations with GEQQQQPGM in TNF-α-injured HUVECs
The NO and iNOS concentrations are shown in Table 2. The concentrations of NO and iNOS in the TNF-α-injured HUVECs were significantly higher than those in the control and GEQQQQPGM groups (P < 0.01). The peptide had no significant effect on the NO and iNOS concentrations in the normal HUVECs; however, GEQQQQPGM decreased the NO and iNOS concentrations significantly (P < 0.05) in the TNF-α-injured HUVECs.
Table 2 The effects of GEQQQQPGM on the NO and iNOS concentrations and oxidative stress indexes in the TNF-α-injured HUVECsa
|
Control |
GEQQQQPGM |
TNF-α |
GEQQQQPGM + TNF-α |
The means ± SD were determined from 6 separate experiments. * and ** indicate P < 0.05 and P < 0.01, respectively, compared to the control; # and ## indicate P < 0.05 and P < 0.01, compared to TNF-α alone. |
NO (μmol L−1) |
21.8 ± 2.9 |
23.7 ± 2.4 |
44.7 ± 3.2** |
35.0 ± 3.6# |
iNOS (pg mL−1) |
463 ± 27 |
438 ± 25 |
658 ± 26** |
572 ± 18# |
MDA (mmol L−1) |
1.20 ± 0.06 |
0.68 ± 0.05** |
1.71 ± 0.08** |
0.85 ± 0.06## |
GSH-Px (U per mg protein) |
83.6 ± 3.1 |
86.7 ± 3.7 |
65.7 ± 3.4** |
78.2 ± 4.9# |
SOD (U per mg protein) |
258 ± 8 |
260 ± 11 |
215 ± 9** |
246 ± 10## |
3.6. The effects of GEQQQQPGM on the oxidative stress indexes in TNF-α-injured HUVECs
As shown in Table 2, the HUVECs treated with TNF-α showed significantly lower the GSH-Px and SOD activities and higher MDA content when compared with the control and GEQQQQPGM groups (P < 0.01). GEQQQQPGM did not affect the activities of GSH-Px and SOD, and the MDA content in the normal HUVECs; however, it increased the GSH-Px (P < 0.01) and SOD (P < 0.01) activities and decreased the MDA content (P < 0.05) significantly in the TNF-α-injured HUVECs.
3.7. The effects of GEQQQQPGM on the ROS in TNF-α-injured HUVECs
As shown in Fig. 4, basal ROS production in the HUVECs was reduced by GEQQQQPGM alone (P < 0.05) without the TNF-α treatment. The peptide GEQQQQPGM markedly inhibited the increase in TNF-α-induced ROS production (P < 0.01).
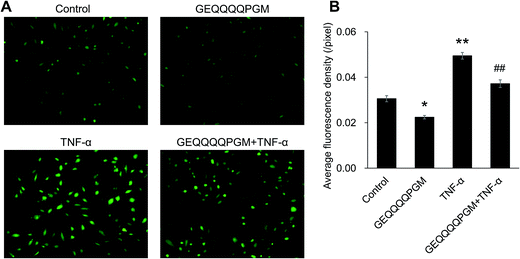 |
| Fig. 4 The effect of GEQQQQPGM on oxidative stress in the TNF-α-injured HUVECs. Confluent HUVECs were pretreated with GEQQQQPGM (25 μg mL−1) for 18 h prior to incubation with 10 ng mL−1 for 6 h. The fluorescence intensity was measured using Image J software. The bars represent the mean value ± SE, n = 6. * and ** indicate P < 0.05 and P < 0.01, respectively, compared to the control; ## indicates P < 0.01, compared to TNF-α alone. (B) Represents the statistical results of (A). | |
3.8. GEQQQQPGM inhibited the nuclear translocation of p65 protein in TNF-α-injured HUVECs
The p65 protein in the HUVECs was observed by staining (Fig. 5). The stimulation of TNF-α caused the nuclear translocation of p65 protein. Moreover, pretreatment with GEQQQQPGM significantly inhibited the TNF-α induced-nuclear localization of p65 protein.
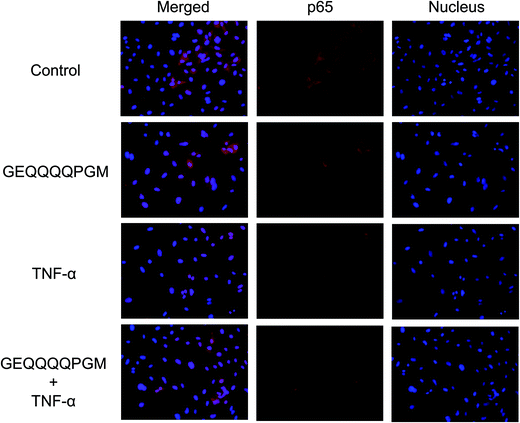 |
| Fig. 5 The effects of GEQQQQPGM on p65 translocation in the TNF-α-injured HUVECs. Confluent HUVECs were pretreated with GEQQQQPGM (25 μg mL−1) for 6 h prior to incubation with 10 ng mL−1 TNF-α for 1 h. The nucleus and p65 protein were dyed using blue and red fluorescence, respectively (n = 6). | |
3.9. GEQQQQPGM inhibited the IKK-NF-κB pathway in TNF-α-injured HUVECs
To confirm the effects of GEQQQQPGM on the IKK-NF-κB pathway, the protein expression of p65, IKKα, and IκBα in the TNF-α-injured HUVECs was evaluated. As shown in Fig. 6, the protein expressions of p65 and IKKα were greatly increased by the TNF-α treatment, whereas GEQQQQPGM suppressed the TNF-α-induced up-regulation significantly (P < 0.01). The protein expression of IκBα was greatly reduced by the TNF-α treatment, whereas GEQQQQPGM also suppressed the TNF-α-induced down-regulation significantly (P < 0.05).
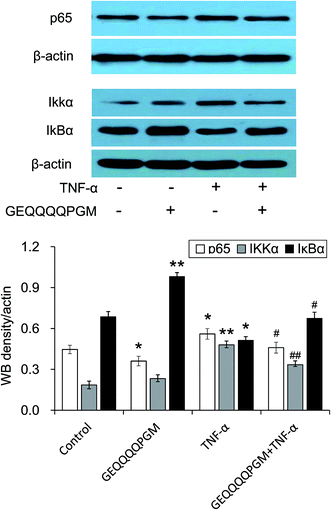 |
| Fig. 6 GEQQQQPGM inhibited the IKK-NF-κB pathway in TNF-α-injured HUVECs. Confluent HUVECs were pretreated with GEQQQQPGM (25 μg mL−1) for 6 h prior to incubation with 10 ng mL−1 TNF-α for 1 h. The protein expressions of nuclear p65, IKK, and IκBα were measured by Western blot analysis. The bars represent the mean value ± SE, n = 6. * and ** indicate P < 0.05 and P < 0.01, respectively, compared to the control; # and ## indicates P < 0.01, compared to TNF-α alone. | |
4. Discussion
It has been widely reported that the intake of food proteins can significantly reduce the lesion of atherosclerosis that is closely involved in the pathogenesis of CVDs.1,2,9 Moreover, functional peptides, such as IRW, WH, HGI, and HGK, derived from food proteins have been reported to prevent atherosclerosis by improving the function of vascular cells.6,13,14 Previous animal experiments have indicated that rice α-globulin (100 mg per day per kg body weight) can efficiently prevent atherosclerosis in ApoE−/− mice; however, the mechanism is not clear.15 In the present study, GEQQQQPGM was confirmed to be one of the key fragments in rice α-globulin after gastrointestinal digestion and absorption, and the peptide was able to enter the plasma (Fig. 1 and ESI 1†). Moreover, we demonstrated that orally administrated GEQQQQPGM had significantly lower plasma total and LDL cholesterol concentrations and atherogenic index in hypercholesterolemic hamsters fed with a hypercholesterolemic diet (P < 0.05) (Table 1). This result indicated that GEQQQQPGM as one of the functional peptide fragments in rice α-globulin was directly responsible for its anti-atherogenic effect. This was consistent with a previous report on the anti-atherogenic effect of peptides stating that oral administration of the dipeptide WH derived from soybean globulin inhibited progressive atherosclerotic lesions in ApoE−/− mice at 100 mg per day per kg bodyweight.12
To clarify the mechanism(s) of the anti-atherogenic effect, in the present study, we determined the effect of the peptide GEQQQQPGM digested and absorbed from rice α-globulin in the human body on TNF-α-induced HUVECs injury. As a result, the viability of the TNF-α-injured HUVECs was significantly increased by GEQQQQPGM at the doses of 50, 100, and 200 μg mL−1; this showed that the peptide could play a role in protecting vascular cell injury (Fig. 2). This result suggests that GEQQQQPGM, as one of the functional peptide fragments in rice α-globulin, reduces the atherosclerosis risk due to its protective effects on vascular endothelial cell injury. Similarity, the tripeptide IRW derived from egg protein reduced the TNF-α-induced cell injury by reducing the inflammatory response, oxidative stress, and the levels of superoxide ions in HUVECs.6
TNF-α stimulation can cause an inflammatory response and oxidative stress in vascular endothelial cell injury; this leads to the up regulation of MCP-1, ICAM-1, and VCAM-1 expression.16 In the present study, MCP-1, ICAM-1, and VCAM-1 were over expressed in the TNF-α-injured HUVECs, which was consistent with the previous studies.6,17 The peptide significantly suppressed the up-regulation of these cell molecules in the HUVECs treated with TNF-α (Fig. 3). Moreover, the TNF-α induced the excessive synthesis of NO as an indicator of inflammation by the up-regulation of iNOS expression in the HUVECs; this led to endothelial cell injury and atherosclerotic plaque formation.18–20 In this study, GEQQQQPGM significantly lowered the NO and iNOS concentrations in the TNF-α-injured HUVECs (Table 2). These results showed that GEQQQQPGM improved TNF-α-induced vascular endothelial cell injury by inhibiting the inflammatory response.
Endothelial cell injury in atherosclerosis leads to the mitochondrial overproduction of ROS, which are traditionally regarded as the toxic by-products of aerobic metabolism because the stability of ROS, such as superoxide anion, hydrogen peroxide, and hydroxyl radicals, is very important for redox balance.21–23 Our results showed that GEQQQQPGM pretreatment significantly inhibited ROS production in the TNF-α-injured HUVECs (Fig. 4). In addition, the peptide significantly reduces the MDA content, which is an important index for evaluating the degree of lipid peroxidation (Table 2). It has been widely reported that ROS can be detoxified by GSH-Px and SOD as the anti-oxidative enzymes.5 In the present study, we demonstrated that GEQQQQPGM significantly increased the GSH-Px and SOD activities in TNF-α-injured HUVECs (Table 2). Therefore, the protective effect of GEQQQQPGM could be partially attributed to the amelioration of oxidative stress in TNF-α-induced HUVEC injury. A similar mechanism for improving TNF-α-induced vascular endothelial cell injury has also been observed in the previous studies on the peptide IRW derived from egg protein.6
Numerous studies have confirmed that the mechanism of a self-amplifying signal in the inflammatory response is iNOS regulated by NF-κB in endothelial cells.20,24 Moreover, NF-κB is the main target for ROS, and its activation has been linked to endothelial dysfunction.25,26 Therefore, the IKK-NF-κB pathway was involved in the pathogenesis of atherosclerosis via regulation of the transcription of inflammation and oxidative stress genes. In this pathway, activated IKKα leads to phosphorylation and degradation of IκBα. Then, the p65 proteins are induced and released from the cytosol and migrate into the cell nucleus, where they interact with the promoter regions of various proteins that are up-regulated during inflammation.27 In the present study, we investigated whether GEQQQQPGM exerted beneficial effects on the IKK-NF-κB pathway. The results show that GEQQQQPGM can obviously inhibit the TNF-α-induced nuclear localization of p65 protein (Fig. 5). In addition, the peptide exerted a beneficial effect on the protein expression of p65, IKKα, and IκBα in the TNF-α-injured HUVECs (Fig. 6). This finding showed that GEQQQQPGM reduced the inflammatory response and oxidative stress by inhibiting the inflammatory activation of NF-κB.
5. Conclusions
In conclusion, the present study suggested that GEQQQQPGM, as one of functional peptide fragments in rice α-globulin, was directly responsible for its anti-atherogenic effect. GEQQQQPGM reduces the atherosclerosis risk due to its protective effects on vascular endothelial cell injury. Moreover, this study clearly shows that GEQQQQPGM at a dose of 50 μg mL−1 reduced 10 ng mL−1 of TNF-α-induced inflammatory response and oxidative stress in HUVECs by inhibiting the inflammatory activation of NF-κB, thus preventing vascular endothelial cell injury. In addition, our results indicated that the peptide GEQQQQPGM as one of functional peptide fragments was directly responsible for the improvement of rice α-globulin in cardiovascular disease.
Conflicts of interest
There are no conflicts to declare.
Abbreviations
HUVECs | Human umbilical vein endothelial cells |
TNF-α | Tumor necrosis factor-α |
CCK-8 | Cell counting kit-8 |
ICAM-1 | Intercellular adhesion molecule-1 |
VCAM-1 | Vascular cell adhesion molecule-1 |
MCP-1 | Monocyte chemoattractant protein-1 |
ROS | Reactive oxygen species |
NO | Nitric oxide |
iNOS | Inducible nitric oxide synthase |
SOD | Superoxide dismutase |
GSH-PX | Glutathione peroxidase |
MDA | Malondialdehyde |
GFC | Gel filtration chromatography |
HPLC | High performance liquid chromatography |
LC-MS | Liquid chromatography-mass spectrometry |
NF-κB | Nuclear factor κB |
IKK | Inhibitor of nuclear factor κB kinase |
IκB | Inhibitor of NF-κB |
FBS | Fetal calf serum |
EDTA | Ethylenediaminetetraacetic acid |
PMSF | Phenylmethanesulfonyl fluoride |
Acknowledgements
This work was supported by the National Natural Science Foundation of China (Grant No. 31401505).
References
- T. Watanabe, K. Sato, F. Itoh, Y. Noguchi, K. Fujimoto, T. Koyama and M. Shichiri, Emerging roles for vasoactive peptides in diagnostic and therapeutic strategies against atherosclerotic cardiovascular diseases, Curr. Protein Pept. Sci., 2013, 14, 472–480 CrossRef CAS PubMed.
- A. Berezin, A. Zulli, S. Kerrigan, D. Petrovic and P. Kruzliak, Predictive role of circulating endothelial-derived microparticles in cardiovascular diseases, Clin. Biochem., 2015, 48, 562–568 CrossRef CAS PubMed.
- E. Mannarino and M. Pirro, Endothelial injury and repair: a novel theory for atherosclerosis, Angiology, 2008, 59, 69–72 CrossRef PubMed.
- X. Chen, S. Pang, J. Lin, J. Xia and Y. Wang, Allicin prevents oxidized low-density lipoprotein-induced endothelial cell injury by inhibiting apoptosis and oxidative stress pathway, BMC Complementary Altern. Med., 2016, 16, 1–6 Search PubMed.
- H. Chai, Q. Wang, L. Huang, T. Xie and Y. Fu, Ginsenoside Rb1 inhibits tumor necrosis factor-alpha-induced vascular cell adhesion molecule-1 expression in human endothelial cells, Biol. Pharm. Bull., 2008, 31, 2050–2056 CAS.
- W. Huang, S. Chakrabarti, K. Majumder, Y. Jiang, S. T. Davidge and J. Wu, Egg-derived peptide IRW inhibits TNF-alpha-induced inflammatory response and oxidative stress in endothelial cells, J. Agric. Food Chem., 2010, 58, 10840–10846 CrossRef CAS PubMed.
- W. Ni, Y. Tsuda, S. Takashima, H. Sato, M. Sato and K. Imaizumi, Anti-atherogenic effect of soya and rice-protein isolate, compared with casein, in apolipoprotein E-deficient mice, Br. J. Nutr., 2003, 90, 13–20 CrossRef CAS PubMed.
- L. Yang, G. Han, Q. H. Liu, Q. Wu, H. J. He, C. Z. Cheng and Y. J. Duan, Rice protein exerts a hypocholesterolemic effect through regulating cholesterol metabolism-related gene expression and enzyme activity in adult rats fed a cholesterol-enriched diet, Int. J. Food Sci. Nutr., 2013, 64, 836–842 CrossRef CAS PubMed.
- L. T. Tong, L. Guo, X. Zhou, J. Qiu, L. Liu, K. Zhong and S. Zhou, Effects of dietary oat proteins on cholesterol metabolism of hypercholesterolaemic hamsters, J. Sci. Food Agric., 2016, 96, 1396–1401 CrossRef CAS PubMed.
- B. H. Sarmadi and A. Ismail, Antioxidative peptides from food proteins: a review, Peptides, 2010, 31, 1949–1956 CrossRef CAS PubMed.
- S. A. Ingersoll, S. Ayyadurai, M. A. Charania, H. Laroui, Y. Yan and D. Merlin, The role and pathophysiological relevance of membrane transporter PepT1 in intestinal inflammation and inflammatory bowel disease, Am. J. Physiol., 2012, 302, 484–492 Search PubMed.
- T. Matsui, M. Sato, M. Tanaka, Y. Yamada, S. Watanabe, Y. Fujimoto, K. Imaizumi and K. Matsumoto, Vasodilating dipeptide Trp-His can prevent atherosclerosis in apo E-deficient mice, Br. J. Nutr., 2010, 103, 309–313 CrossRef CAS PubMed.
- Y. Kobayashi, T. Fukuda, M. Tanaka and T. Matsui, The anti-atherosclerotic di-peptide, Trp-His, inhibits the phosphorylation of voltage-dependent L-type Ca2+ channels in rat vascular smooth muscle cells, FEBS Open Bio, 2012, 2, 83–88 CrossRef PubMed.
- T. Kumrungsee, Z. Q. Wang, S. Matsumura, T. Saiki, M. Tanaka and T. Matsui, Identification of peptides from soybean protein, glycinin, possessing suppression of intracellular Ca2+ concentration in vascular smooth muscle cells, Food Chem., 2014, 152, 218–224 CrossRef CAS PubMed.
- L. T. Tong, Y. Fujimoto, N. Shimizu, M. Tsukino, T. Akasaka, Y. Kato, W. Iwamoto, S. Shiratake, K. Imaizumi and M. Sato, Rice alpha-globulin decreases serum cholesterol concentrations in rats fed a hypercholesterolemic diet and ameliorates atherosclerotic lesions in apolipoprotein E-deficient mice, Food Chem., 2012, 132, 194–200 CrossRef CAS PubMed.
- Z. Zhou, Y. Liu, A. D. Miao and S. Q Wang, Protocatechuic aldehyde suppresses TNF-alpha-induced ICAM-1 and VCAM-1 expression in human umbilical vein endothelial cells, Eur. J. Pharmacol., 2005, 513, 1–8 CrossRef CAS PubMed.
- L. Yang, R. M. Froio, T. E. Sciuto, A. M. Dvorak, R. Alon and F. W. Luscinskas, ICAM-1 regulates neutrophil adhesion and transcellular migration of TNF-alpha-activated vascular endothelium under flow, Blood, 2005, 106, 584–592 CrossRef CAS PubMed.
- B. Y. Wang, H. K. Ho, P. S. Lin, S. P. Schwarzacher, M. J. Pollman, G. H. Gibbons and J. P. Cooke, Regression of atherosclerosis: role of nitric oxide and apoptosis, Circulation, 1999, 99, 1236–1241 CrossRef CAS PubMed.
- M. C. Assis, M. C. Plotkowski, I. M. Fierro, C. Barja-Fidalgo and M. S. Freitas, Expression of inducible nitric oxide synthase in human umbilical vein endothelial cells during primary culture, Nitric Oxide, 2002, 7, 254–261 CrossRef.
- N. Ruimi, R. D. Petrova, R. Agbaria, S. Sussan, S. P. Wasser, A. Z. Reznick and J. Mahajna, Inhibition of TNF alpha-induced iNOS expression in HSV-tk transduced 9L glioblastoma cell lines by Marasmius oreades substances through NF-kappa B- and MAPK-dependent mechanisms, Mol. Biol. Rep., 2010, 37, 3801–3812 CrossRef CAS PubMed.
- T. Y. Aw, Molecular and cellular responses to oxidative stress and changes in oxidation-reduction imbalance in the intestine, Am. J. Clin. Nutr., 1999, 70, 557–565 CAS.
- K. Irani, Oxidant signaling in vascular cell growth, death, and survival: a review of the roles of reactive oxygen species in smooth muscle and endothelial cell mitogenic and apoptotic signaling, Circ. Res., 2000, 87, 179–183 CrossRef CAS PubMed.
- J. Shen, S. Shen, U. N. Das and G. Xu, Effect of essential fatty acids on glucose-induced cytotoxicity to retinal vascular endothelial cells, Lipids Health Dis., 2012, 11, 1–10 CrossRef PubMed.
- S. S. Deshpande, P. Angkeow, J. Huang, M. Ozaki and K. Irani, Rac1 inhibits TNF-alpha-induced endothelial cell apoptosis: dual regulation by reactive oxygen species, FASEB J., 2000, 14, 1705–1714 CrossRef CAS PubMed.
- G. Gloire, S. Legrandpoels and J. Piette, NF-kappa B activation by reactive oxygen species: fifteen years later, Biochem. Pharmacol., 2006, 72, 1493–1505 CrossRef CAS PubMed.
- E. Melgarejo, M. A. Medina, F. Sanchez-Jimenez and J. L. Urdiales, Monocyte chemoattractant protein-1: a key mediator in inflammatory processes, Int. J. Biochem. Cell Biol., 2009, 41, 998–1001 CrossRef CAS PubMed.
- R. G. Baker, M. S. Hayden and S. Ghosh, NF-kappa B, inflammation, and metabolic disease, Cell Metab., 2011, 13, 11–22 CrossRef CAS PubMed.
Footnotes |
† Electronic supplementary information (ESI) available. See DOI: 10.1039/c7ra08304a |
‡ Equal contributors. |
|
This journal is © The Royal Society of Chemistry 2017 |
Click here to see how this site uses Cookies. View our privacy policy here.