DOI:
10.1039/C7RA07635E
(Paper)
RSC Adv., 2017,
7, 44054-44058
Surface plasmon resonance based competitive immunoassay for Cd2+†
Received
11th July 2017
, Accepted 7th September 2017
First published on 12th September 2017
Abstract
In this study, a label-free, specific and sensitive surface plasmon resonance (SPR) based competitive immunoassay was used for detecting Cd2+ in water samples. The standard curve of the method for Cd2+ was constructed in the concentration range of 0–1000 ng mL−1. The values of IC50 and the LOD of the assay were found to be 23.58 and 1.25 ng mL−1. No cross-reactivity was found with other metal ions except Hg2+. The proposed method provides an alternative method for sensitive, specific and real-time determination of Cd2+ in real aqueous samples.
1. Introduction
Cadmium is one of the most toxic heavy metals for human beings: it has great environmental and health impact and all cadmium compounds have been classified as human carcinogens.1,2 Dietary intake of cadmium from water and crops in polluted environments results in various human diseases.3 Therefore, monitoring cadmium in water samples is urgently needed to protect human health and safety.
Up to now, cadmium can be determined by some classical and modern analytical methods, such as atomic absorption spectroscopy (AAS),4 inductively coupled plasma mass spectrometry (ICP-MS),5 Nonthermal Optical Emission Spectrometry,6 graphite furnace atomic absorption spectrometry (GF-AAS),7 and miniature dielectric barrier discharge optical emission spectrometric (DBD-OES).8 These methods are sensitive and accurate, but there are some disadvantages for these approaches to detect the cadmium, such as longer consumption times, increased technical expertise and tedious sample pre-treatments, and some labeling methods are not suitable in some cases, because labeling materials may occupy important binding sites or cause steric hindrance, resulting in false information.
Surface plasmon resonance (SPR) is an optical phenomenon occurred in total internal reflection of light at a metal film–liquid interface,9 which is one of the powerful analytical techniques for direct monitoring of molecular interactions. SPR is based on measuring binding-induced changes in refractive index and this platform thus allows for label-free, real-time analysis of the target molecules. In recent years, SPR has been applied in drug discovery,10 biomarkers screening,11 food safety12,13 and environmental monitoring.14,15
Besides, the immunological detection method based on antigen–antibody has provided a new strategy for the detection of cadmium pollution, and there have been many reports about the immunoassay of cadmium.16–18 The members of our research group have also established many methods for detecting metal ions.19–24
Herein, we report a specific and sensitive competitive immunoassay combined SPR for the detection of cadmium (Cd2+) in aqueous solution for the first time. At present, all antibodies specific for cadmium recognize a chelated form of Cd2+, that is, Cd–EDTA.25–29 In our previous study, cadmium ion was respectively coupled to carrier protein (bovine serum albumin, BSA; ovalbumin, OVA) via a phenyl isothiocyanate–EDTA (ITCBE) to form the immunogen (Cd–ITCBE–BSA) and coating antigen (Cd–ITCBE–OVA). Using the hybridoma technique we successfully produced monoclonal antibody (mAb) against Cd2+, which were then characterized by an indirect competitive enzyme linked immunosorbent assay (ELISA).30 Combining the specific mAb with the sensitive and lable-free analytical SPR method, we expect that the SPR based immunoassay will be able to rapid detect Cd2+ with high sensitivity and specificity.
2. Experimental
2.1. Chemicals and reagents
N-Hydroxysuccinimide (NHS), N-(3-dimethylaminopropyl)-N′-ethylcarbodiimide hydrochloride (EDC), 4-(2-hydroxyethyl)-1-piper-azineethanesulfonic acid (HEPES), bovine serum albumin (BSA), chicken egg albumin (OVA), ethanolamine (≧98%), glycine (Vetec™ reagent grade, 98%) were purchased from Aladdin (Shanghai, China). All other chemical reagents were of analytical grade and used without further purification. The antigen and antibody were self-made in this experiment. Detection experiment of Cd2+ in real aqueous samples, lake water sample was collected from Wenying Lake in Datong city and tap water was obtained from our laboratory. In this work, 10 mM HEPES buffer (pH 7.4) containing 150 mM NaCl, 3 mM EDTA, 0.005% (v/v) surfactant P20 was employed as running buffer and 10 mM acetate buffer solution was used as coupling buffer. Ultrapure water was used for the preparation of aqueous solutions.
2.2. Instrumentation
The Biacore X™ (Uppsala, Sweden) and CM5 sensor chip were used for all in situ surface bioaffinity detection. All solutions for experiments were filtered by 0.22 μm microporous membrane and degassed before use. Running buffer was HBS–EP buffer solution (pH 7.4) throughout the instrument at 10 μL min−1 flow rate. CM5 sensor chips and all solutions were kept at room temperature before use.
2.3. Immobilization of antigen
Many methods of antibody immobilization used in SPR have been developed,31,32 however, the antigen was immobilized to determine Cd2+ in this experiment. Under optimal assay conditions, the self-made coating antigen (Cd–ITCBE–OVA) immobilization experiment was carried out at first, the whole process are shown in Fig. 1. A sensor chip CM5 was docked into the Biacore instrument firstly and primed with running buffer (HBS–EP, pH 7.4) at 10 μL min−1 flow rate. The EDC/NHS mixed solution was injected (70 μL, 10 μL min−1) to activate the surface of the sensor chip CM5. This was immediately followed by immobilization antigen (Cd–ITCBE–OVA) (70 μL, 10 μL min−1) prepared in 10 mM sodium acetate buffer (pH 3.75). Then the remaining activate sites of the sensor chip CM5 surface was blocked with 70 μL ethanolamine (pH 8.5). In this way, the immobilization of antigen was completed. Eventually, it was found that the corresponding signal value of the combinative amount of antigen was 2526 RU.
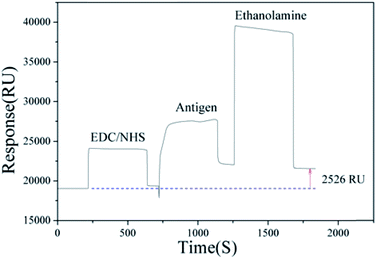 |
| Fig. 1 Immobilization of the antigen. | |
2.4. The whole process of Cd2+ detection assay
The principle and process of the method are illustrated in Scheme 1. The CM5 dextran chip surface was firstly activated by EDC/NHS solution, after the coating antigen was immobilized, then the surface was deactivated with ethanolamine. The coating antigen competed for the mAbs with Cd2+ in the solution. The SPR response change decreased due to the less amount of the mAbs bound on the chip surface, which was caused by the competition of the high concentration of Cd2+ in the solution.
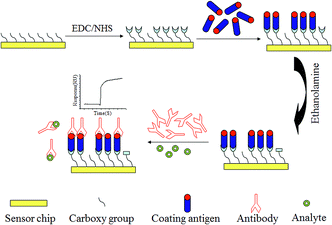 |
| Scheme 1 The principle and process of the SPR based competitive immunoassay for Cd2+. | |
3. Results and discussion
In order to obtain much more sensitive results in this study, the SPR relevant experimental conditions were optimized respectively before the antigen binding experiment. To select the optimal pH value of acetate buffer solution (the antigen diluent), nine parallel experiments were measured so that the corresponding signals were obtained, as shown in Fig. S1 (ESI†), it was seen that the corresponding signal of pH value 3.75 was the strongest one, so the acetate buffer solution of pH value 3.75 was selected. Then choosing the appropriate antigen concentration, five experiments with different antigen dilution rates were carried out, as exhibited in Fig. S2 (ESI†), it was seen that with the dilution rate increasing, the response values were significantly decreased, but due to the high cost of antigen, resulting in 1
:
10 antigen dilution rate was selected. Finally, four parallel experiments with different flow rates were measured respectively, as exhibited in Fig. S3 (ESI†), the 10 μL min−1 flow rate was chosen after comprehensive consideration. After screening, it was concluded that the results were satisfactory under these optimal conditions.
The resulting chip above was used as a sensing surface for detecting Cd2+. The mixed solution (v/v = 1
:
1) of the cadmium mAb solution and Cd–EDTA standard solution was prepared, and Cd–EDTA standard solution was diluted into different concentration with HBS–EP before mixing. These solutions were then injected at 10 μL min−1 flow rate over the coating antigen (Cd–ITCBA–OVA) modified surface for 3 min to allow binding assays. Following this, the sensor surface was regenerated by injected 10 μL 10 mM glycine–HCl (pH 1.7). The corresponding signals and the calibration curve of the SPR based immunoassay for Cd2+ in different standard concentrations are shown in Fig. 2 and 3. It was found that the SPR signal steadily decreased as the concentration of Cd2+ increased. The IC50 value was found to be 23.58 ng mL−1, the limit of detection (LOD) was 1.25 ng mL−1 and the linear range of concentration was 3.57–758.37 ng mL−1. Compared with other methods for Cd2+ detection, our method had a similar or superior linear range and detection limit (Table S1, ESI†).
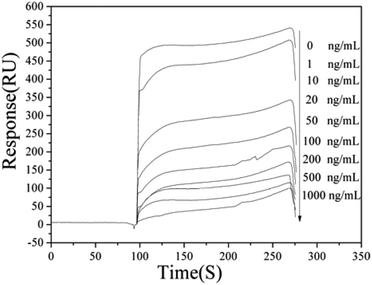 |
| Fig. 2 The SPR signals of the SPR based immunoassay for Cd2+ with different standard concentrations. The arrow shows the signal changes with increasing Cd–EDTA concentrations (0, 1, 10, 20, 50, 100, 200, 500, 1000 ng mL−1). | |
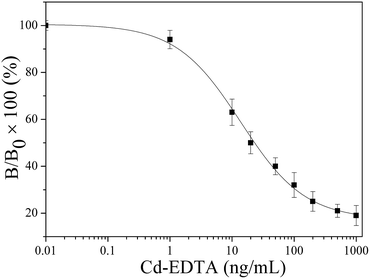 |
| Fig. 3 The calibration curve of the SPR based immunoassay for Cd2+ with different standard concentrations, where B and B0 are the SPR signals of the analyte at the standard point and at the zero concentration of the analyte, respectively. | |
In order to demonstrate the binding of cadmium mAbs to Cd2+ was specific, metal ions such as Cu2+, Ni2+, Co2+, Cr3+, Pb2+, Mg2+ and Zn2+ were selected to test the cross-reactivity. The concentration of the cross-reacting ions were 0–1000 ng mL−1 and applied according to the procedure of the assay, as shown in Fig. 4. One could conclude that no cross-reactivity of the mAbs with Cu2+, Ni2+, Co2+, Cr3+, Pb2+, Mg2+ and Zn2+ respectively, but the cross-reactivity of the mAbs with Hg2+ was 73.12%, which was actually rather serious. The results obtained in this approach were similar to the ELISA using the same mAb.30 It could be caused by the spatial configuration of the chelates that is respectively formed by Hg2+ and Cd2+ with EDTA are quite similar and the interactions of the chelates with the antibody.
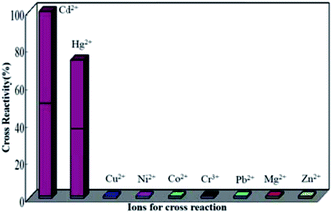 |
| Fig. 4 The cross-reactivity values of the monoclonal antibody with Cd2+ and other ions tested by the proposed SPR based competitive immunoassay. | |
The practical application of the proposed biosensor was evaluated in lake water and tap water. Samples of lake water and tap water were collected from the Wenying Lake and our laboratory, respectively. Prior to testing, the collected water samples were filtered by 0.22 μm filter membrane to remove insoluble substances. Then, the SPR based competitive immunoassay was applied to detect the filtrates which were spiked with Cd–EDTA at the concentration of 10 ng mL−1, 20 ng mL−1 and 50 ng mL−1, respectively. Acceptable recovery rates of 96.80–102.37% were obtained (as shown in Table 1), which suggested that the method could be used for the detection of Cd2+ in real aqueous samples.
Table 1 Determination of Cd2+ in water samples using the proposed method
Samples |
Added (ng mL−1) |
Found, mean ± SD (ng mL−1, n = 3) |
Recovery (%) |
Lake water |
10 |
9.85 ± 0.63 |
98.54 |
20 |
19.36 ± 1.12 |
96.80 |
50 |
48.42 ± 3.07 |
96.84 |
Tap water |
10 |
10.24 ± 0.59 |
102.37 |
20 |
19.52 ± 1.23 |
97.60 |
50 |
49.16 ± 2.85 |
98.31 |
Note that various cellphone (CP)-based technologies have been developed for multifarious applications.33–35 What's more, combining an angle-resolved SPR detection system with cellphone-based devices (CBDs) has been developed and employed for the detection of β2 microglobulin (β2M),36 and a good analysis result was obtained. Hence, in the near future, heavy metal ions may also be detected by combining SPR with CBDs technique so that develop a prospective upcoming devices in the environmental and food monitoring.
4. Conclusions
In summary, a sensitive and specific SPR based competitive immunoassay for the detection of Cd2+ was developed. It provided a new theoretical and technical support for the detection of heavy metal ions. Based on this novel method, the values of IC50 (23.58 ng mL−1) and LOD (1.25 ng mL−1) were obtained. In addition to Hg2+, this method showed good selectivity for Cd2+ without interference of some other heavy metal ions. Moreover, the sensing strategy could real-time monitor and shorten the analytical time, which is shorter than previously reported methods.37–40 This novel biosensor approach not only provides an alternative, sensitive and specific analytical method for the detection of Cd2+, but can also be extended as a useful model for the detection of other small molecular compounds in biological, food and environmental areas.
Conflicts of interest
There are no conflicts of interest to declare.
Acknowledgements
This work was supported by the National Natural Science Foundation of China (21375083), Key Scientific and Technological Projects of Datong City (2015025, 2015023), Scientific and Technological Innovation Programs of Higher Education Institutions in Shanxi (2015179) and Doctoral Scientific Research projects of Shanxi Datong University (2012-B-08, 2014-B-03).
Notes and references
- A. A. Newairy, A. S. El-Sharaky, M. M. Badreldeen, S. M. Eweda and S. A. Sheweita, Toxicology, 2007, 242, 23 CrossRef CAS PubMed
. - S. Nemmiche, D. Chabane-Sari, M. Kadri and P. Guiraud, Toxicol. In Vitro, 2011, 25, 191 CrossRef CAS PubMed
. - M. J. Scoullos, G. H. Vonkeman, I. Thornton and Z. Makuch, Mercury-Cadmium-Lead: Handbook for Sustainable Heavy Metals Policy and Regulation, Springer, Netherlands, 2001 Search PubMed
. -
(a) J. Gasparik, D. Vladarova, M. Capcarova, P. Smehyl, J. Slamecka and P. Garaj, J. Environ. Sci. Health, Part A: Toxic/Hazard. Subst. Environ. Eng., 2010, 45, 818 CrossRef CAS PubMed
;
(b) P. Pohl, TrAC, Trends Anal. Chem., 2009, 28, 117 CrossRef CAS
. -
(a) C. Cloquet, J. Carignan, G. Libourel, T. Sterckeman and E. Perdrix, Environ. Sci. Technol., 2006, 40, 2525 CrossRef CAS PubMed
;
(b) S. P. Dolan, D. A. Nortrup, P. M. Bolger and S. G. Capar, J. Agric. Food Chem., 2003, 51, 1307 CrossRef CAS PubMed
;
(c) M. Grau-Perez, G. Pichler, I. Galan-Chilet, L. S. Briongos-Figuero, P. Rentero-Garrido and R. Lopez-Izquierdo, Environ. Int., 2017, 106, 27 CrossRef CAS PubMed
. - Y. Cai, Y. J. Zhang, D. F. Wu, Y. L. Yu and J. H. Wang, Anal. Chem., 2016, 88, 4192 CrossRef CAS PubMed
. - J. E. L. Villa, R. R. A. Peixoto and S. Cadore, J. Agric. Food Chem., 2014, 62, 8759 CrossRef CAS PubMed
. - Y. J. Zhang, Y. Cai, Y. L. Yu and J. H. Wang, Anal. Chim. Acta, 2017, 976, 45 CrossRef CAS PubMed
. - M. Piliarik, H. Vaisocherova and J. Homola, Methods Mol. Biol., 2009, 503, 65 CAS
. - Y. Fang, Expert Opin. Drug Discovery, 2012, 7, 969 CrossRef CAS PubMed
. - A. Shabani and M. Tabrizian, Analyst, 2013, 138, 6052 RSC
. - C. Situ, J. Buijs, M. H. Mooney and C. T. Elliott, TrAC, Trends Anal. Chem., 2010, 29, 1305 CrossRef CAS
. - M. Piliarik, L. Párová and J. Homola, Biosens. Bioelectron., 2009, 24, 1399 CrossRef CAS PubMed
. - R. Yatabe, T. Onodera and K. Toko, Sensors, 2013, 13, 9294 CrossRef CAS PubMed
. - S. Scarano, M. Mascini, A. P. F. Turner and M. Minunni, Biosens. Bioelectron., 2010, 25, 957 CrossRef CAS PubMed
. - K. Abe, K. Nakamura and T. Arao, J. Sci. Food Agric., 2011, 91, 1392 CrossRef CAS PubMed
. - K. Sasaki, N. Yongvongsoontorn and K. Tawarada, J. Agric. Food Chem., 2009, 57, 4514 CrossRef CAS PubMed
. - I. A. Darwish and D. A. Blake, Anal. Chem., 2001, 73, 1889 CrossRef CAS PubMed
. - J. Qin, X. M. Li, Z. Chen and F. Feng, Sens. Actuators, B, 2016, 232, 611 CrossRef CAS
. - Y. H. Yuan, M. Z. Tian, J. L. Wang, H. Xie, J. Qin and F. Feng, RSC Adv., 2015, 5, 69453 RSC
. - Y. F. Bai, L. Zhao, Z. Chen, H. Y. Wang and F. Feng, Anal. Methods, 2014, 6, 8120 RSC
. - Y. F. Bai, F. Feng, L. Zhao, Z. Chen, H. Y. Wang and Y. L. Duan, Anal. Methods, 2014, 6, 662 RSC
. - Y. Z. Wang, S. Chen, C. Wei, M. M. Xu, J. L. Yao, Y. Li, A. P. Deng and R. N. Gu, Chem. Commun., 2014, 50, 9112 RSC
. - Y. Z. Wang, H. Yang, M. Pschenitza, R. Niessner, Y. Li, D. Knopp and A. P. Deng, Anal. Bioanal. Chem., 2012, 403, 2519 CrossRef CAS PubMed
. - D. A. Blake, P. Chakrabarti, M. Khosraviani, F. M. Hatcher, C. M. Westhoff, P. Goebel, D. E. Wylie and R. C. Blake, J. Biol. Chem., 1996, 271, 27677 CrossRef CAS PubMed
. - K. Tawarada, K. Sasaki, N. Ohmura, N. Matsumoto and H. Saiki, Bunseki Kagaku, 2003, 52, 583 CrossRef CAS
. - K. Sasaki, K. Tawarada, A. Okuyama, K. F. ayama, K. Abe, H. Okuhata, Y. Maruyama, T. Arakane, H. Miyasaka, T. Fujikawa and N. Ohmura, Bunseki Kagaku, 2007, 56, 29 CrossRef CAS
. - X. Zhu, L. Xu, H. Yu, X. Li, D. A. Blake and F. Liu, J. Agric. Food Chem., 2007, 55, 7648 CrossRef CAS PubMed
. - K. Sasaki, N. Yongvongsoontorn, K. Tawarada, Y. Ohnisni, T. Arakane, F. Kayama, K. Abe, S. Oguma and N. Ohmura, J. Agric. Food Chem., 2009, 57, 4514 CrossRef CAS PubMed
. - J. Q. Zhou, F. Feng, Y. Z. Wang and A. P. Deng, Chem. Res. Appl., 2016, 28, 432 Search PubMed
. - S. K. Vashist, C. K. Dixit, B. D. MacCraith and R. O'Kennedy, Analyst, 2011, 136, 4431 RSC
. - S. K. Vashist, E. M. Schneider and J. H. T. Luong, Analyst, 2014, 139, 2237 RSC
. - S. K. Vashist, O. Mudanyali, E. M. Schneider, R. Zengerle and A. Ozcan, Anal. Bioanal. Chem., 2014, 406, 3263 CrossRef CAS PubMed
. - A. Ozcan, Lab Chip, 2014, 14, 3187 RSC
. - S. K. Vashist, P. B. Luppa, L. Y. Yeo, A. Ozcan and J. H. Luong, Trends Biotechnol., 2015, 33, 692 CrossRef CAS PubMed
. - P. Preechaburana, M. C. Gonzalez, A. Suska and D. Filippini, Angew. Chem., 2012, 124, 11753 CrossRef
. - L. Z. Zhao, M. Li, M. M. Liu, Y. C. Zhang, C. L. Wu and Y. Z. Zhang, J. Hazard. Mater., 2016, 301, 233 CrossRef CAS PubMed
. - K. Duarte, C. I. L. Justino, A. C. Freitas, A. M. P. Gomes, A. C. Duarte and T. A. P. Rocha-Santos, TrAC, Trends Anal. Chem., 2015, 64, 183 CrossRef CAS
. - E. Nagles, V. Arancibia, R. Ríos and C. Rojas, Int. J. Electrochem. Sci., 2012, 7, 5521 CAS
. - M. C. Talio, M. Alesso, M. Acosta, V. S. Wills and L. P. Fernández, Talanta, 2017, 174, 221 CrossRef CAS PubMed
.
Footnotes |
† Electronic supplementary information (ESI) available. See DOI: 10.1039/c7ra07635e |
‡ These authors contributed equally to this work. |
|
This journal is © The Royal Society of Chemistry 2017 |
Click here to see how this site uses Cookies. View our privacy policy here.