DOI:
10.1039/C7RA07306B
(Paper)
RSC Adv., 2017,
7, 41723-41726
Cu-catalyzed intermolecular oxyalkylation of styrenes under air: access to diverse iminolactones†
Received
2nd July 2017
, Accepted 21st August 2017
First published on 25th August 2017
Abstract
A practical, simple, and efficient copper-catalyzed highly regioselective oxyalkylation of styrenes and readily available α-bromoacetamides under air is realized. This reaction exhibits a wide range of functional group tolerance in styrenes and α-bromoacetamides to afford iminolactones.
Difunctionalization of alkenes is a powerful tool for the synthesis of heterocycles through tandem two same or different C–X (X = C, N, O, etc.) bond formations in organic synthesis. Although metal-free as well as transition-metal-catalyzed aminocarbonylation, aminoarylation, aminovinylation, aminoalkynylation, and aminooxygenation reactions of alkenes have been significant developments in recent decades,1 the selective oxyalkylation reactions of alkenes for their efficiency in synthesizing different types of oxygen-containing heterocycles remains challenging.2 N-Substituted iminolactones, which can be easily converted to γ-lactones, are prevalently used as antibacterial agents, aldosterone inhibitors, and proper precursors for the preparation of a wide spectrum of natural compounds.3 However, the selective oxyalkylation of alkenes for the synthesis of N-substituted iminolactones are still rare. In this context, Lei and coworkers developed Ni-catalyzed cyclization of α-haloamides with alkenes through carbon-centered radical addition to the carbonyl oxygen of amides or esters (Scheme 1a).4 Recently, Nishikata and coworkers reported copper-catalyzed oxyalkylation of alkenes to afford iminolactone by controlling the reactivity of the oxygen nucleophile of the amide group (Scheme 1b).5 However, these methods require long reaction time, anhydrous solvent, inert atmosphere protection and sometimes even special apparatuses such as the glovebox to manipulate the reactions. Therefore, a practical and simple methodology towards effective intermolecular oxyalkylation of alkenes is highly desirable. Recently, we developed selectfluor-mediated highly selective radical dioxygenation of alkenes and metal-free catalyzed C–O bond formation reactions directly from C–H bonds.6 As part of our ongoing study on difunctionalization of alkenes6a,d and C–O bond formation reactions, we present herein our recent progress in copper-catalyzed highly regioselective oxyalkylation of styrenes and readily available α-bromoacetamides under air.
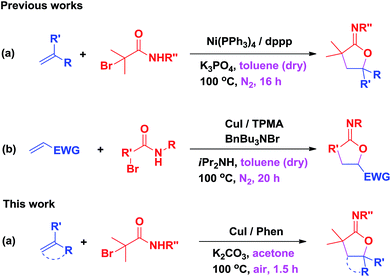 |
| Scheme 1 Oxyalkylation of alkenes with α-bromoacetamides. | |
We started our investigation by applying styrene 1a and 2-bromo-2-methyl-N-phenylpropanamide 2a in a model reaction to optimize the reaction conditions. To our delight, in the preliminary experiment of using 10 mol% of CuI as catalyst, the desired oxyalkylation proceeded to give the desired product 3a in 45% yield in the presence of 1,10-phenanthroline (Phen) and K2CO3 at 100 °C in CH3CN (Table 1, entry 1). Product 3a was obtained in 83% yield when acetone was employed as the solvent (Table 1, entry 2). In contrast, other solvents such as CH2Cl2, DMF, and EtOAc did not perform well (Table 1, entries 3–5). Further investigation on different copper salts revealed that CuBr, CuCl and Cu(OTf)2 were also efficient catalyst for this transformation, affording product 3a in a satisfying 80%, 79% and 81% yield, respectively (Table 1, entries 6–8). Other ligand such as PPh3 and 2,2′-bipyridine (bipy) were less effective than Phen (Table 1, entries 9 and 10). Other bases such as NaOAc and Et3N gave the desired product in lower yield (Table 1, entries 11 and 12). Control experiments demonstrated that CuI, Phen and K2CO3 were all important (entries 13–15). In addition, trace amounts of 3a was obtained when the reaction was performed at 70 °C (Table 1, entry 16).
Table 1 Optimization of the reaction conditionsa
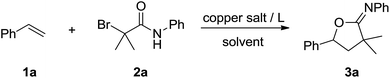
|
Entry |
Metal |
Ligand |
Additive |
Solvent |
Yieldb (%) |
Reactions were carried out with 1a (0.45 mmol), 2a (0.3 mmol), metal (10 mol%), ligand (10 mol%) and additive (2.0 equiv.) in 3 mL solvent under air atmosphere at 100 °C for 1.5 h, unless noted otherwise. Yield of the isolated product. The reaction was performed at 70 °C. Phen = 1,10-phenanthroline, Tf = trifluoromethanesulfonyl, bipy = 2,2′-bipyridine. |
1 |
CuI |
Phen |
K2CO3 |
CH3CN |
45 |
2 |
CuI |
Phen |
K2CO3 |
Acetone |
83 |
3 |
CuI |
Phen |
K2CO3 |
CH2Cl2 |
Trace |
4 |
CuI |
Ph3P |
K2CO3 |
DMF |
18 |
5 |
CuI |
Phen |
K2CO3 |
EtOAc |
Trace |
6 |
CuBr |
Phen |
K2CO3 |
Acetone |
80 |
7 |
CuCl |
Phen |
K2CO3 |
Acetone |
79 |
8 |
Cu(OTf)2 |
Phen |
K2CO3 |
Acetone |
81 |
9 |
CuI |
PPh3 |
K2CO3 |
Acetone |
37 |
10 |
CuI |
bipy |
K2CO3 |
Acetone |
41 |
11 |
CuI |
Phen |
NaOAc |
Acetone |
45 |
12 |
CuI |
Phen |
Et3N |
Acetone |
40 |
13 |
None |
Phen |
K2CO3 |
Acetone |
Trace |
14 |
CuI |
None |
K2CO3 |
Acetone |
Trace |
15 |
CuI |
Phen |
None |
Acetone |
0 |
16c |
CuI |
Phen |
K2CO3 |
Acetone |
Trace |
The scope of the oxyalkylation of styrenes was then investigated under the optimized reaction conditions (Table 1, entry 5). As described in Table 2, a broad range of styrene derivatives were investigated. Generally, styrene substrates bearing electron-donating substituents provided higher yields than those containing electron-withdrawing substituents on the aromatic ring (3b–3h). Halo-substituted styrene derivatives (1b–1d, 1i–1m) were tolerated in the oxyalkylation reaction of styrenes, allowing for further functionalization through a cross-coupling manifold. Starting from disubstituted styrene 1,4-dimethyl-2-vinylbenzene, 3n was obtained in 71% yield. In addition, the cyclic alkene substrates such as indene also provided the desired oxyalkylation product 3o in 70% yield. Notably, 1,1-disubstituted styrenes such as 1p–1r were also effective to provide 3q–3s in 80–86% yields. In addition, starting from methyl acrylate (1s), the corresponding product 3s could be obtained in 78% yields. Remarkably, in all cases, the reactions proceeded smoothly under air, and the desired iminolactones (3a–3r) were consistently obtained in 60–93% yields with high regioselectivity.
Table 2 Reactions of alkenes 1 with 2aa,b
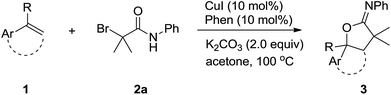
|
Reactions were carried out with 1 (0.45 mmol), 2a (0.3 mmol), CuI (10 mol%), Phen (10 mol%) and K2CO3 (2.0 equiv.) in acetone (3 mL) under air atmosphere at 100 °C for 1.5 h. Yield of the isolated product. |
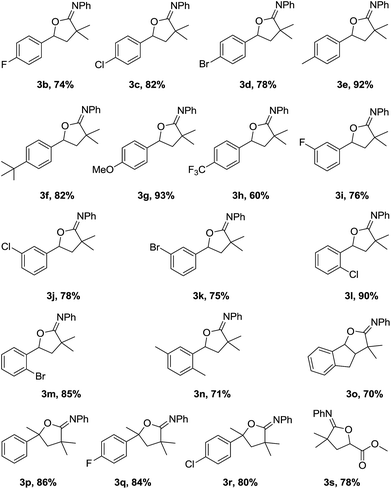 |
This oxyalkylation of styrenes was further expanded to a range of α-bromoacetamides, and the results are shown in Table 3. The results indicate that reactions of α-bromoacetamides with different substituents on the aromatic ring, such as F, Cl, Br, nitryl, methyl, ethyl, tert-butyl, and methoxy, proceeded well with moderate to good yields. This reaction trend is also consistent with the electronic effect of styrenes. In addition, the steric hindrance on the aryl ring also influence the reaction. Slightly decreased yields were achieved for ortho-substituted α-bromoacetamides (2k–2n).
Table 3 Reactions of styrene 1a with α-bromoacetamides 2 a,b
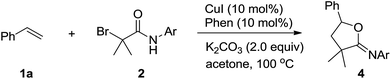
|
Reactions were carried out with 1a (0.45 mmol), 2 (0.3 mmol), CuI (10 mol%), Phen (10 mol%) and K2CO3 (2.0 equiv.) in acetone (3 mL) under air atmosphere at 100 °C for 1.5 h. Yield of the isolated product. |
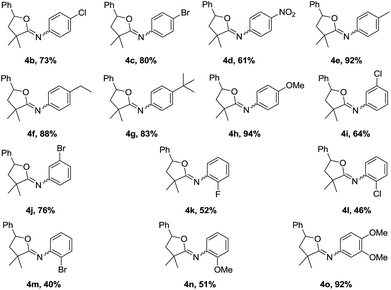 |
Furthermore, a sequential one-pot two-step tandem reaction for efficient synthesis of γ-lactones was investigated (Table 4). For example, the γ-lactone 5a was obtained in 78% yield when the reaction system of 1a with 2a was quenched in situ by HCl (2 M aqueous solution). Starting from 1c and 1f, the corresponding compounds 5b and 5c could be obtained in 75% and 80% yields, respectively. In addition, 1,1-disubstituted styrenes such as prop-1-en-2-ylbenzene 1p was also effective to provide 5d in 76% yield. The present method was applied to citronellol derivatives 3,7-dimethyloct-6-en-1-yl acrylate 6, and the corresponding product 7 was obtained in 56% yield [eqn (1)].
|
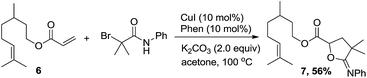 | (1) |
|
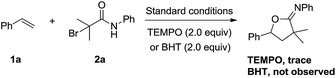 | (2) |
|
 | (3) |
Table 4 Direct synthesis of γ-lactones from styrenes 1 and 2a a,b

|
Reactions conditions: (1) 1 (0.45 mmol), 2a (0.3 mmol), CuI (10 mol%), Phen (10 mol%), K2CO3 (2.0 equiv.) and acetone (3 mL), air, 100 °C, 1.5 h; (2) HCl (2 M), air, 60 °C, 5 h. Yield of the isolated product. |
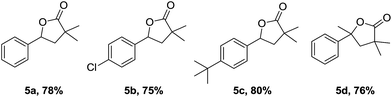 |
Some mechanistic experiments have been investigated to probe the mechanism of this transformation. When the radical scavenger such as 2,2,6,6-tetramethylpiperidine N-oxide (TEMPO, 2.0 equiv.) and 2,6-di-tert-butyl-4-methylphenol (BHT, 2.0 equiv.) were added to the oxyalkylation reaction of 1a under the optimal conditions, no desired products were isolated [eqn (2)]. These results indicate that a radical pathway may be involved under the catalytic system. Further evidence for a radical mechanism was demonstrated by the radical clock experiment with (1-cyclopropylvinyl)benzene as the substrate. The reaction of (1-cyclopropylvinyl)benzene with 2h under the standard reaction conditions produced the ring-expanded products 8 in 31% yield [eqn (3)].7
On the basis of the present experimental results, a possible mechanism was proposed in Scheme 2. The CuI catalyst reacts with 2a to generate a tertiary-alkyl radical species A 8 and a CuII species.9 Then, addition of A to styrene takes place to give benzyl radical B.9a,b,10 The intramolecular addition of benzyl radical to a carbonyl group of amide produces C,11 which then undergoes radical oxidation and deprotonation to give the oxyalkylation product 3a and regenerate CuI in the presence of CuII and K2CO3. According to the hard and soft acid and base theory (HSAB), highly selective C–O bond formation may be due to an easier amide oxygen attack under weakly basic conditions in soft acid solvents acetone.12
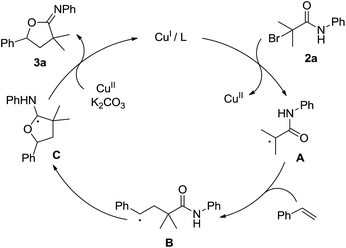 |
| Scheme 2 Proposed mechanism. | |
Conclusions
In conclusion, a novel copper-catalyzed intermolecular oxyalkylation of styrenes and readily available α-bromoacetamides has been reported. This work provided an efficient route to synthesize iminolactones, which can be conveniently converted into γ-lactones. Moreover, this reaction features high regioselectivity, excellent functional-group tolerance, no extra oxidant, no inert atmosphere protection and can greatly simplify the operation and workup procedures, which may be a good alternative for the existing methods. Further mechanistic investigations are underway in our lab.
Conflicts of interest
There are no conflicts to declare.
Acknowledgements
We gratefully acknowledge the Science & Technology Foundation of Henan Province, the Henan province key laboratory of new opto-electronic functional materials and AYNU-KP-A04 for financial support.
Notes and references
-
(a) G. Zhang, B. Gao and H. Huang, Angew. Chem., Int. Ed., 2015, 54, 7657 CrossRef CAS PubMed;
(b) J. Cheng, X. Qi, P. Chen and G. Liu, J. Am. Chem. Soc., 2015, 137, 2480 CrossRef CAS PubMed;
(c) S. Hayashi, H. Yorimitsu and K. Oshima, Angew. Chem., Int. Ed., 2009, 48, 7224 CrossRef CAS PubMed;
(d) K. T. Yip and D. Yang, Org. Lett., 2011, 13, 2134 CrossRef CAS PubMed;
(e) S. Jaegli, J. Dufour, H.-L. Wei, T. Piou, X.-H. Duan, J.-P. Vors, L. Neuville and J. Zhu, Org. Lett., 2010, 12, 4498 CrossRef CAS PubMed;
(f) C. F. Rosewall, P. A. Sibbald, D. V. Liskin and F. E. Michael, J. Am. Chem. Soc., 2009, 131, 9488 CrossRef CAS PubMed;
(g) P. A. Sibbald, C. F. Rosewall, R. D. Swartz and F. E. Michael, J. Am. Chem. Soc., 2009, 131, 15945 CrossRef CAS PubMed;
(h) K. T. Yip, M. Yang, K. L. Law, N. Y. Zhu and D. Yang, J. Am. Chem. Soc., 2006, 128, 3130 CrossRef CAS PubMed;
(i) K. T. Yip, N. Y. Zhu and D. Yang, Org. Lett., 2009, 11, 1911 CrossRef CAS PubMed;
(j) W. He, K. T. Yip, N. Y. Zhu and D. Yang, Org. Lett., 2009, 11, 5626 CrossRef CAS PubMed;
(k) S. Nicolai and J. Waser, Org. Lett., 2011, 13, 6324 CrossRef CAS PubMed;
(l) S. Nicolai, C. Piemontesi and J. Waser, Angew. Chem., Int. Ed., 2011, 50, 4680 CrossRef CAS PubMed;
(m) B. N. Hemric, K. Shen and Q. Wang, J. Am. Chem. Soc., 2016, 138, 5813 CrossRef CAS PubMed;
(n) S. R. Chemler and P. H. Fuller, Chem. Soc. Rev., 2007, 36, 1153 RSC;
(o) T. J. Donohoe, C. K. A. Callens, A. Flores, A. R. Lacy and A. H. Rathi, Chem.–Eur. J., 2011, 17, 58 CrossRef CAS PubMed;
(p) J. Xie, Y.-W. Wang, L.-W. Qi and B. Zhang, Org. Lett., 2017, 19, 1148 CrossRef CAS PubMed;
(q) W.-H. Rao, X.-S. Yin and B.-F. Shi, Org. Lett., 2015, 17, 3758 CrossRef CAS PubMed;
(r) L. Liu and Z. Wang, Green Chem., 2017, 19, 2076 RSC.
-
(a) R. Zhu and S. L. Buchwald, J. Am. Chem. Soc., 2015, 137, 8069 CrossRef CAS PubMed;
(b) X.-J. Wei, D.-T. Yang, L. Wang, T. Song, L.-Z. Wu and Q. Liu, Org. Lett., 2013, 15, 6054 CrossRef CAS PubMed;
(c) L. H. Powell, P. H. Docherty, D. G. Hulcoop, P. D. Kemmitt and J. W. Burton, Chem. Commun., 2008, 22, 2559 RSC.
-
(a) M. A. Oliaruso and J. F. Wolf, in Synthesis of Lactones and Lactams, Wiley, New York, 1993 Search PubMed;
(b) A. Shaabani, E. Soleimani and A. Sarvary, Monatsh. Chem., 2008, 139, 629 CrossRef CAS;
(c) N. Chatani, M. Oshita, M. Tobisu, Y. Ishii and S. Murai, J. Am. Chem. Soc., 2003, 125, 7812 CrossRef CAS PubMed.
- D. Liu, S. Tang, H. Yi, C. Liu, X. Qi, Y. Lan and A. Lei, Chem.–Eur. J., 2014, 20, 15605 CrossRef CAS PubMed.
-
(a) Y. Yamane, K. Miyazaki and T. Nishikata, ACS Catal., 2016, 6, 7418 CrossRef CAS;
(b) T. Nishikata, K. Itonaga, N. Yamaguchi and M. Sumimoto, Org. Lett., 2017, 19, 2686 CrossRef CAS PubMed.
-
(a) Y.-H. Lv, X. Wang, H. Cui, K. Sun, W.-Y. Pu, G. Li, Y.-T. Wu, J.-L. He and X.-R. Ren, RSC Adv., 2016, 6, 74917 RSC;
(b) Y.-H. Lv, K. Sun, T.-T. Wang, G. Li, W.-Y. Pu, N.-N. Chai, H.-H. Shen and Y.-T. Wu, RSC Adv., 2015, 5, 72142 RSC;
(c) Y.-H. Lv, K. Sun, W.-Y. Pu, S.-K. Mao, G. Li, J.-J. Niu, Q. Chen and T.-T. Wang, RSC Adv., 2016, 6, 93486 RSC;
(d) Y.-H. Lv, W.-Y. Pu, H. Cui, J.-L. He and Q.-M. Zhang, Synth. Commun., 2016, 46, 1223 CrossRef CAS.
-
(a) Z. Feng, Q.-Q. Min, H.-Y. Zhao, J.-W. Gu and X. Zhang, Angew. Chem., Int. Ed., 2015, 54, 1270 CrossRef CAS PubMed;
(b) F. Zhang, Q.-Q. Min and X. Zhang, Synthesis, 2015, 47, 2912 CrossRef CAS.
-
(a) J.-H. Fan, W.-T. Wei, M.-B. Zhou, R.-J. Song and J.-H. Li, Angew. Chem., Int. Ed., 2014, 53, 6650 CrossRef CAS PubMed;
(b) Y. Li, B. Liu, X.-H. Ouyang, R.-J. Song and J.-H. Li, Org. Chem. Front., 2015, 2, 1457 RSC;
(c) M. Li, J. Yang, X.-H. Ouyang, Y. Yang, M. Hu, R.-J. Song and J.-H. Li, J. Org. Chem., 2016, 81, 7148 CrossRef CAS PubMed;
(d) M. Hu, R.-J. Song, X.-H. Ouyang, F.-L. Tan, W.-T. Wei and J.-H. Li, Chem. Commun., 2016, 52, 3328 RSC;
(e) X.-H. Ouyang, R.-J. Song, M. Hu, Y. Yang and J.-H. Li, Angew. Chem., Int. Ed., 2016, 55, 3187 CrossRef CAS PubMed;
(f) C.-Y. Wang, G.-H. Pan, F. Chen and J.-H. Li, Chem. Commun., 2017, 53, 4730 RSC;
(g) M. Hu, L.-Y. Guo, Y. Han, F.-L. Tan, R.-J. Song and J.-H. Li, Chem. Commun., 2017, 53, 6081 RSC.
-
(a) T. Nishikata, Y. Noda, R. Fujimoto and T. Sakashita, J. Am. Chem. Soc., 2013, 135, 16372 CrossRef CAS PubMed;
(b) A. J. Clark, Eur. J. Org. Chem., 2016, 2016, 2231 CrossRef CAS;
(c) K. Matyjaszweski, Macromolecules, 2012, 45, 4015 CrossRef;
(d) C. Theunissen, J. Wang and G. Evano, Chem. Sci., 2017, 8, 3465 RSC.
-
(a) C. Liu, S. Tang, D. Liu, J. Yuan, L. Zheng, L. Meng and A. Lei, Angew. Chem., Int. Ed., 2012, 51, 3638 CrossRef CAS PubMed;
(b) I. Ryu, N. Sonoda and D. P. Curran, Chem. Rev., 1996, 96, 177 CrossRef CAS PubMed;
(c) H. Togo, Advanced Free Radical Reactions for Organic Synthesis, 1st edn, Elsevier Ltd., Amsterdam, 2004 Search PubMed.
-
(a) A. S. Kende and J. L. Belletire, Tetrahedron Lett., 1972, 13, 2145 CrossRef;
(b) G. D. Mendenhall, J. D. Protasiewicz, C. E. Brown, K. U. Ingold and J. Lusztyk, J. Am. Chem. Soc., 1994, 116, 1718 CrossRef CAS;
(c) D. A. Harrison, R. N. Schwartz and J. Kagan, J. Am. Chem. Soc., 1970, 92, 5793 CrossRef CAS;
(d) Y. Yamamoto, M. Ohno and S. Eguchi, J. Org. Chem., 1996, 61, 9264 CrossRef CAS;
(e) Y. Yamamoto, M. Ohno and S. Eguchi, J. Org. Chem., 1994, 59, 4707 CrossRef CAS.
- R. G. Pearson, J. Am. Chem. Soc., 1963, 85, 3533 CrossRef CAS.
Footnote |
† Electronic supplementary information (ESI) available. See DOI: 10.1039/c7ra07306b |
|
This journal is © The Royal Society of Chemistry 2017 |
Click here to see how this site uses Cookies. View our privacy policy here.